7.6: Sex Chromosomes
- Page ID
- 4850
\( \newcommand{\vecs}[1]{\overset { \scriptstyle \rightharpoonup} {\mathbf{#1}} } \)
\( \newcommand{\vecd}[1]{\overset{-\!-\!\rightharpoonup}{\vphantom{a}\smash {#1}}} \)
\( \newcommand{\id}{\mathrm{id}}\) \( \newcommand{\Span}{\mathrm{span}}\)
( \newcommand{\kernel}{\mathrm{null}\,}\) \( \newcommand{\range}{\mathrm{range}\,}\)
\( \newcommand{\RealPart}{\mathrm{Re}}\) \( \newcommand{\ImaginaryPart}{\mathrm{Im}}\)
\( \newcommand{\Argument}{\mathrm{Arg}}\) \( \newcommand{\norm}[1]{\| #1 \|}\)
\( \newcommand{\inner}[2]{\langle #1, #2 \rangle}\)
\( \newcommand{\Span}{\mathrm{span}}\)
\( \newcommand{\id}{\mathrm{id}}\)
\( \newcommand{\Span}{\mathrm{span}}\)
\( \newcommand{\kernel}{\mathrm{null}\,}\)
\( \newcommand{\range}{\mathrm{range}\,}\)
\( \newcommand{\RealPart}{\mathrm{Re}}\)
\( \newcommand{\ImaginaryPart}{\mathrm{Im}}\)
\( \newcommand{\Argument}{\mathrm{Arg}}\)
\( \newcommand{\norm}[1]{\| #1 \|}\)
\( \newcommand{\inner}[2]{\langle #1, #2 \rangle}\)
\( \newcommand{\Span}{\mathrm{span}}\) \( \newcommand{\AA}{\unicode[.8,0]{x212B}}\)
\( \newcommand{\vectorA}[1]{\vec{#1}} % arrow\)
\( \newcommand{\vectorAt}[1]{\vec{\text{#1}}} % arrow\)
\( \newcommand{\vectorB}[1]{\overset { \scriptstyle \rightharpoonup} {\mathbf{#1}} } \)
\( \newcommand{\vectorC}[1]{\textbf{#1}} \)
\( \newcommand{\vectorD}[1]{\overrightarrow{#1}} \)
\( \newcommand{\vectorDt}[1]{\overrightarrow{\text{#1}}} \)
\( \newcommand{\vectE}[1]{\overset{-\!-\!\rightharpoonup}{\vphantom{a}\smash{\mathbf {#1}}}} \)
\( \newcommand{\vecs}[1]{\overset { \scriptstyle \rightharpoonup} {\mathbf{#1}} } \)
\( \newcommand{\vecd}[1]{\overset{-\!-\!\rightharpoonup}{\vphantom{a}\smash {#1}}} \)
\(\newcommand{\avec}{\mathbf a}\) \(\newcommand{\bvec}{\mathbf b}\) \(\newcommand{\cvec}{\mathbf c}\) \(\newcommand{\dvec}{\mathbf d}\) \(\newcommand{\dtil}{\widetilde{\mathbf d}}\) \(\newcommand{\evec}{\mathbf e}\) \(\newcommand{\fvec}{\mathbf f}\) \(\newcommand{\nvec}{\mathbf n}\) \(\newcommand{\pvec}{\mathbf p}\) \(\newcommand{\qvec}{\mathbf q}\) \(\newcommand{\svec}{\mathbf s}\) \(\newcommand{\tvec}{\mathbf t}\) \(\newcommand{\uvec}{\mathbf u}\) \(\newcommand{\vvec}{\mathbf v}\) \(\newcommand{\wvec}{\mathbf w}\) \(\newcommand{\xvec}{\mathbf x}\) \(\newcommand{\yvec}{\mathbf y}\) \(\newcommand{\zvec}{\mathbf z}\) \(\newcommand{\rvec}{\mathbf r}\) \(\newcommand{\mvec}{\mathbf m}\) \(\newcommand{\zerovec}{\mathbf 0}\) \(\newcommand{\onevec}{\mathbf 1}\) \(\newcommand{\real}{\mathbb R}\) \(\newcommand{\twovec}[2]{\left[\begin{array}{r}#1 \\ #2 \end{array}\right]}\) \(\newcommand{\ctwovec}[2]{\left[\begin{array}{c}#1 \\ #2 \end{array}\right]}\) \(\newcommand{\threevec}[3]{\left[\begin{array}{r}#1 \\ #2 \\ #3 \end{array}\right]}\) \(\newcommand{\cthreevec}[3]{\left[\begin{array}{c}#1 \\ #2 \\ #3 \end{array}\right]}\) \(\newcommand{\fourvec}[4]{\left[\begin{array}{r}#1 \\ #2 \\ #3 \\ #4 \end{array}\right]}\) \(\newcommand{\cfourvec}[4]{\left[\begin{array}{c}#1 \\ #2 \\ #3 \\ #4 \end{array}\right]}\) \(\newcommand{\fivevec}[5]{\left[\begin{array}{r}#1 \\ #2 \\ #3 \\ #4 \\ #5 \\ \end{array}\right]}\) \(\newcommand{\cfivevec}[5]{\left[\begin{array}{c}#1 \\ #2 \\ #3 \\ #4 \\ #5 \\ \end{array}\right]}\) \(\newcommand{\mattwo}[4]{\left[\begin{array}{rr}#1 \amp #2 \\ #3 \amp #4 \\ \end{array}\right]}\) \(\newcommand{\laspan}[1]{\text{Span}\{#1\}}\) \(\newcommand{\bcal}{\cal B}\) \(\newcommand{\ccal}{\cal C}\) \(\newcommand{\scal}{\cal S}\) \(\newcommand{\wcal}{\cal W}\) \(\newcommand{\ecal}{\cal E}\) \(\newcommand{\coords}[2]{\left\{#1\right\}_{#2}}\) \(\newcommand{\gray}[1]{\color{gray}{#1}}\) \(\newcommand{\lgray}[1]{\color{lightgray}{#1}}\) \(\newcommand{\rank}{\operatorname{rank}}\) \(\newcommand{\row}{\text{Row}}\) \(\newcommand{\col}{\text{Col}}\) \(\renewcommand{\row}{\text{Row}}\) \(\newcommand{\nul}{\text{Nul}}\) \(\newcommand{\var}{\text{Var}}\) \(\newcommand{\corr}{\text{corr}}\) \(\newcommand{\len}[1]{\left|#1\right|}\) \(\newcommand{\bbar}{\overline{\bvec}}\) \(\newcommand{\bhat}{\widehat{\bvec}}\) \(\newcommand{\bperp}{\bvec^\perp}\) \(\newcommand{\xhat}{\widehat{\xvec}}\) \(\newcommand{\vhat}{\widehat{\vvec}}\) \(\newcommand{\uhat}{\widehat{\uvec}}\) \(\newcommand{\what}{\widehat{\wvec}}\) \(\newcommand{\Sighat}{\widehat{\Sigma}}\) \(\newcommand{\lt}{<}\) \(\newcommand{\gt}{>}\) \(\newcommand{\amp}{&}\) \(\definecolor{fillinmathshade}{gray}{0.9}\)The nuclei of human cells contain 22 autosomes and 2 sex chromosomes. In females, the sex chromosomes are the 2 X chromosomes. Males have one X chromosome and one Y chromosome. The presence of the Y chromosome is decisive for unleashing the developmental program that leads to a baby boy.
The Y Chromosome
In making sperm by meiosis, the X and Y chromosomes must separate in anaphase just as homologous autosomes do. This occurs without a problem because, like homologous autosomes, the X and Y chromosome synapse during prophase of meiosis I. There is a small region of homology shared by the X and Y chromosome and synapsis occurs at that region.
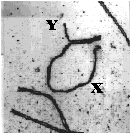
This image, shows synapsis of the X and Y chromosomes of a mouse during prophase of meiosis I. Crossing over occurs in two regions of pairing, called the pseudoautosomal regions. These are located at opposite ends of the chromosome.
The Pseudoautosomal Regions
The pseudoautosomal regions get their name because any genes located within them (so far only 9 have been found) are inherited just like any autosomal genes. Males have two copies of these genes: one in the pseudoautosomal region of their Y, the other in the corresponding portion of their X chromosome. So males can inherit an allele originally present on the X chromosome of their father and females can inherit an allele originally present on the Y chromosome of their father.

Genes outside the pseudoautosomal regions
Although 95% of the Y chromosome lies between the pseudoautosomal regions, only 27 different functional genes have been found here. Over half of this region is genetically-barren heterochromatin. Of the 27 genes found in the euchromatin, some encode proteins used by all cells. The others encode proteins that appear to function only in the testes. A key player in this latter group is SRY.
SRY
SRY (for sex-determining region Y) is a gene located on the short (p) arm just outside the pseudoautosomal region. It is the master switch that triggers the events that converts the embryo into a male. Without this gene, you get a female instead.
What is the evidence?
- On very rare occasions aneuploid humans are born with such karyotypes as XXY, XXXY, and even XXXXY. Despite their extra X chromosomes, all these cases are male.
- This image shows two mice with an XX karyotype (and thus they should be female). However, as you may be able to see, they have a male phenotype. This is because they are transgenic for SRY. Fertilized XX eggs were injected with DNA carrying the SRY gene.
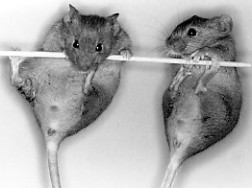
Although these mice have testes, male sex hormones, and normal mating behavior, they are sterile.
- Another rarity: XX humans with testicular tissue because a translocation has placed the SRY gene on one of the X chromosomes
- Still another rarity that demonstrates the case: women with an XY karyotype who, despite their Y chromosome, are female because of a destructive mutation in SRY.
In 1996, a test based on a molecular probe for SRY was used to ensure that potential competitors for the women's Olympic events in Atlanta had no SRY gene. But because of possibilities like that in case 4, this testing is no longer used to screen female Olympic athletes.
The X Chromosome
The X chromosome carries nearly 1,000 genes but few, if any, of these have anything to do directly with sex. However, the inheritance of these genes follows special rules. These arise because:
- males have only a single X chromosome
- almost all the genes on the X have no counterpart on the Y; thus
- any gene on the X, even if recessive in females, will be expressed in males.
Genes inherited in this fashion are described as sex-linked or, more precisely, X-linked.
X-Linkage example
Hemophilia is a blood clotting disorder caused by a mutant gene encoding either
- clotting factor VIII, causing hemophilia A or
- clotting factor IX, causing hemophilia B.
Both genes are located on the X chromosome (shown here in red). With only a single X chromosome, males who inherit the defective gene (always from their mother) will be unable to produce the clotting factor and suffer from difficult-to-control episodes of bleeding. In heterozygous females, the unmutated copy of the gene will provide all the clotting factor they need. Heterozygous females are called "carriers" because although they show no symptoms, they pass the gene on to approximately half their sons, who develop the disease, and half their daughters, who also become carriers.
X | Y | |
---|---|---|
X | XX | XY |
Xh | XhX | XhY |
Women rarely suffer from hemophilia because to do so they would have to inherit a defective gene from their father as well as their mother. Until recently, few hemophiliacs ever became fathers.
X-chromosome Inactivation (XCI)
Human females inherit two copies of every gene on the X chromosome, whereas males inherit only one (with some exceptions: the 9 pseudoautosomal genes and the small number of "housekeeping" genes found on the Y). But for the hundreds of other genes on the X, are males at a disadvantage in the amount of gene product their cells produce? The answer is no, because females have only a single active X chromosome in each cell.
During interphase, chromosomes are too tenuous to be stained and seen by light microscopy. However, a dense, stainable structure, called a Barr body (after its discoverer) is seen in the interphase nuclei of female mammals. The Barr body is one of the X chromosomes. Its compact appearance reflects its inactivity. So, the cells of females have only one functioning copy of each X-linked gene — the same as males.
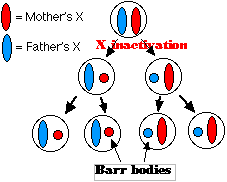
X-chromosome inactivation occurs early in embryonic development. In a given cell, which of a female's X chromosomes becomes inactivated and converted into a Barr body is a matter of chance (except in marsupials like the kangaroo, where it is always the father's X chromosome that is inactivated). After inactivation has occurred, all the descendants of that cell will have the same chromosome inactivated. Thus X-chromosome inactivation creates clones with differing effective gene content. An organism whose cells vary in effective gene content and hence in the expression of a trait, is called a genetic mosaic.
Mechanism of X-chromosome inactivation
Inactivation of an X chromosome requires a gene on that chromosome called XIST.
- XIST is transcribed into a long noncoding RNA.
- XIST RNA accumulates along the X chromosome containing the active XIST gene and proceeds to inactivate all (or almost all) of the hundreds of other genes on that chromosome.
- Barr bodies are inactive X chromosomes "painted" with XIST RNA.
The Sequence of Events in Mice
- During the first cell divisions of the female mouse zygote, the XIST locus on the father's X chromosome is expressed so most of his X-linked genes are silent.
- By the time the blastocyst has formed, the silencing of the paternal X chromosome still continues in the trophoblast (which will go on to form the placenta) but
- in the inner cell mass (the ICM, which will go on to form the embryo) transcription of XIST ceases on the paternal X chromosome allowing its hundreds of other genes to be expressed. The shut-down of the XIST locus is done by methylating XIST regulatory sequences. So the pluripotent stem cells of the ICM express both X chromosomes.
- However, as embryonic development proceeds, X-chromosome inactivation begins again. But this time it is entirely random. There is no predicting whether it will be the maternal X or the paternal X that is inactivated in a given cell.
Some genes on the X chromosome escape inactivation
What about those 18 genes that are found on the Y as well as the X? There should be no need for females to inactivate one copy of these to keep in balance with the situation in males. And, as it turns out, these genes escape inactivation in females. Just how they manage this is still under investigation.
X-chromosome Abnormalities
As we saw above, people are sometimes found with abnormal numbers of X chromosomes. Unlike most cases of aneuploidy, which are lethal, the phenotypic effects of aneuploidy of the X chromosome are usually not severe.
Examples:
- Females with but a single intact X chromosome (usually the one she got from her mother) in some (thus a genetic mosaic) or all of her cells show a variable constellation of phenotypic traits called Turner syndrome. For those girls that survive to birth, the phenotypic effects are generally mild because each cell has a single functioning X chromosome like those of XX females. Number of Barr bodies = zero.
- XXX, XXXX, XXXXX karyotypes: all females with mild phenotypic effects because in each cell all the extra X chromosomes are inactivated. Number of Barr bodies = number of X chromosomes minus one.
- Klinefelter's syndrome: people with XXY or XXXY karyotypes are males (because of their Y chromosome). But again, the phenotypic effects of the extra X chromosomes are mild because, just as in females, the extra Xs are inactivated and converted into Barr bodies.
Sex Determination in Other Animals
Although the male fruit fly, Drosophila melanogaster, is X-Y, the Y chromosome does not dictate its maleness but rather the absence of a second X. Furthermore, instead of females shutting down one X to balance the single X of the males — as we do — male flies double the output of their single X relative to that of females.
In birds, moths, schistosomes, and some lizards, the male has two of the same chromosome (designated ZZ), whereas the female has "heterogametic" chromosomes (designated Z and W). In chickens, a single gene on the Z chromosome (designated DMRT1), when present in a double dose (ZZ), produces males while the presence of only one copy of the gene produces females (ZW).
Environmental Sex Determination
In some cold-blooded vertebrates such as
- fishes
- reptiles (e.g. certain snakes, lizards, turtles, and all crocodiles and alligators)
- invertebrates (e.g. certain crustaceans),
sex is determined after fertilization — not by sex chromosomes deposited in the egg.
The choice is usually determined by the temperature at which early embryonic development takes place.
- In some cases (e.g. many turtles and lizards), a higher temperature during incubation favors the production of females.
- In other cases (e.g., alligators), a higher temperature favors the production of males.
Even in cases (e.g. some lizards) where there are sex chromosomes, a high temperature can convert a genotypic male (ZZ) into a female.
Hermaphrodites
Hermaphrodites have both male and female sex organs. Many species of fish are hermaphroditic.
Some start out as one sex and then, in response to stimuli in their environment, switch to the other.
Other species have both testes and ovaries at the same time (but seldom fertilize themselves). However, populations of C. elegans consist mostly of hermaphrodites and these only fertilize themselves.
Hermaphroditic fishes have no sex chromosomes.