5.9.2: Kingdom Plantae - Adaptations
- Page ID
- 105418
\( \newcommand{\vecs}[1]{\overset { \scriptstyle \rightharpoonup} {\mathbf{#1}} } \)
\( \newcommand{\vecd}[1]{\overset{-\!-\!\rightharpoonup}{\vphantom{a}\smash {#1}}} \)
\( \newcommand{\id}{\mathrm{id}}\) \( \newcommand{\Span}{\mathrm{span}}\)
( \newcommand{\kernel}{\mathrm{null}\,}\) \( \newcommand{\range}{\mathrm{range}\,}\)
\( \newcommand{\RealPart}{\mathrm{Re}}\) \( \newcommand{\ImaginaryPart}{\mathrm{Im}}\)
\( \newcommand{\Argument}{\mathrm{Arg}}\) \( \newcommand{\norm}[1]{\| #1 \|}\)
\( \newcommand{\inner}[2]{\langle #1, #2 \rangle}\)
\( \newcommand{\Span}{\mathrm{span}}\)
\( \newcommand{\id}{\mathrm{id}}\)
\( \newcommand{\Span}{\mathrm{span}}\)
\( \newcommand{\kernel}{\mathrm{null}\,}\)
\( \newcommand{\range}{\mathrm{range}\,}\)
\( \newcommand{\RealPart}{\mathrm{Re}}\)
\( \newcommand{\ImaginaryPart}{\mathrm{Im}}\)
\( \newcommand{\Argument}{\mathrm{Arg}}\)
\( \newcommand{\norm}[1]{\| #1 \|}\)
\( \newcommand{\inner}[2]{\langle #1, #2 \rangle}\)
\( \newcommand{\Span}{\mathrm{span}}\) \( \newcommand{\AA}{\unicode[.8,0]{x212B}}\)
\( \newcommand{\vectorA}[1]{\vec{#1}} % arrow\)
\( \newcommand{\vectorAt}[1]{\vec{\text{#1}}} % arrow\)
\( \newcommand{\vectorB}[1]{\overset { \scriptstyle \rightharpoonup} {\mathbf{#1}} } \)
\( \newcommand{\vectorC}[1]{\textbf{#1}} \)
\( \newcommand{\vectorD}[1]{\overrightarrow{#1}} \)
\( \newcommand{\vectorDt}[1]{\overrightarrow{\text{#1}}} \)
\( \newcommand{\vectE}[1]{\overset{-\!-\!\rightharpoonup}{\vphantom{a}\smash{\mathbf {#1}}}} \)
\( \newcommand{\vecs}[1]{\overset { \scriptstyle \rightharpoonup} {\mathbf{#1}} } \)
\( \newcommand{\vecd}[1]{\overset{-\!-\!\rightharpoonup}{\vphantom{a}\smash {#1}}} \)
Kingdom Plantae - Adaptations
- Please read and watch the following Learning Resources
- Reading the material for understanding, and taking notes during videos, will take approximately 2 hours.
- Optional Activities are embedded.
- Bolded terms are located at the end of the unit in the Glossary. There is also a Unit Summary at the end of the Unit.
- To navigate to the Unit 3 Glossary, use the Contents menu at the top of the page OR the right arrow on the side of the page.
- If on a mobile device, use the Contents menu at the top of the page OR the links at the bottom of the page.
- Discuss the challenges to plant life on land
- Describe the adaptations that allowed plants to colonize the land
- Discuss the importance of adaptations to life on land
- Discuss the type of seeds produced by gymnosperms, as well as other characteristics of gymnosperms
- Describe the main parts of a flower and their purpose
- Detail the life cycle of an angiosperm
- Discuss the two main groups of flowering plants
Introduction
As plants adapted to life on land, they had to contend with several challenges in the terrestrial environment, the largest being a lack of easy access to water as compared to an aquatic environment.
Plant Adaptations to Life on Land
Water has been described as the “universal solvent.” The cell’s interior is a watery soup: in this medium, most small molecules dissolve and diffuse, and the majority of the chemical reactions of metabolism take place. Desiccation, or drying out, is a constant danger for an organism on land when exposed to air. Even when parts of a plant are close to a source of water, the aerial structures are likely to dry out. Water also provides buoyancy to organisms and there is no need for structural support to help the organism maintain a shape or orientation. On land, plants needed to develop structural support since air does not provide the same lift as water. The organism is also subject to bombardment by mutagenic radiation, because air does not filter out as many of the ultraviolet rays of sunlight as water. Additionally, the male gametes must reach the female gametes using new strategies, because swimming is no longer possible. Therefore, both gametes and zygotes must be protected from desiccation. The successful land plants developed strategies to deal with all of these challenges. Not all adaptations appeared at once. Some species never moved very far from the aquatic environment, whereas others went on to conquer the driest environments on Earth.
To balance these survival challenges, life on land offers several advantages. First, sunlight is abundant. Water filters sunlight, altering the spectral quality and reducing the amount of light absorbed by the photosynthetic pigment chlorophyll. Soils provide nutrients where the plants grow, rather than depending on currents and diffusion to bring them. Finally, land plants evolved before land animals; therefore, plants were initially able to evolve without herbivores (although they did have pathogens). As some animals evolved to land-dwelling, plants developed strategies to deter predation: from spines and thorns to toxic chemicals.
Early land plants, like the early land animals, did not live very far from an abundant source of water and developed survival strategies to combat dryness. One of these strategies is called tolerance. Many mosses, for example, can dry out to a brown and brittle mat, but as soon as rain or a flood makes water available, mosses will absorb it and are restored to their healthy green appearance. Another strategy is to colonize environments with high humidity, where droughts are uncommon. Ferns, which are considered an early lineage of plants, thrive in damp and cool places such as the understory of temperate forests. Later, plants moved away from moist or aquatic environments using resistance to desiccation, rather than tolerance. These plants, like cacti, minimize the loss of water to such an extent they can survive in extremely dry environments.
The most successful adaptation was the development of new structures that gave plants the advantage when colonizing new and dry environments. Four major adaptations are found in all terrestrial plants: the alternation of generations, a sporangium in which the spores are formed, a gametangium that produces haploid cells, and apical meristem tissue in roots and shoots. The evolution of a waxy cuticle and a cell wall containing lignin, a complex polymer impermeable to water, also contributed to the success of land plants. These adaptations are noticeably lacking in the closely related green algae—another reason for the debate over their placement in the plant kingdom.
In this 7.5-minute video from Bozeman Science, you will be taken through an overview of plants, their unique characteristics, and their classification.
Questions after watching: Based on the information you learned in this video, create an evolutionary tree of plants containing the 4 groups: bryophytes, ferns and relatives, gymnosperms, and angiosperms. Include the adaptation that evolved at each node and led to the diversification of the plant groups at the appropriate location.
Alternation of Generations
Alternation of generations describes a life cycle in which an organism has both haploid and diploid multicellular stages (Figure \(\PageIndex{1}\)).
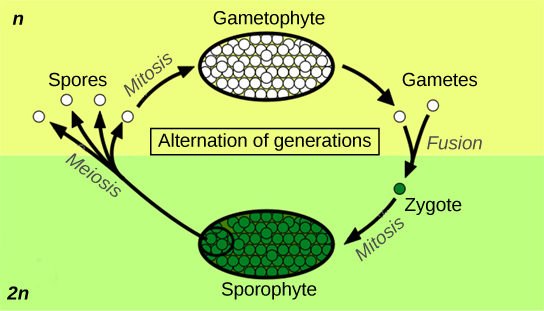
Haplontic refers to a lifecycle in which there is a dominant haploid stage, and diplontic refers to a lifecycle in which the diploid is the dominant life stage. Humans are diplontic. Most plants exhibit alternation of generations, which is described as haplodiplodontic: the haploid multicellular form, known as a gametophyte, is followed in the development sequence by a multicellular diploid organism: the sporophyte. The gametophyte gives rise to the gametes by mitosis. This can be the most obvious phase of the life cycle of the plant, as in the bryophytes, or it can occur in a microscopic structure, such as a pollen grain, in vascular plants. The sporophyte stage is barely noticeable in the non-vascular plants.
Alternation of generations means that plants produce:
- only haploid multicellular organisms
- only diploid multicellular organisms
- only diploid multicellular organisms with single-celled haploid gametes
- both haploid and diploid multicellular organisms
- Answer
-
D
Sporangia and Gametangia
Gametangia (singular, gametangium) are structures observed on multicellular haploid gametophytes. In the gametangia, precursor cells give rise to gametes by mitosis. The male gametangium (antheridium) releases sperm. Many seedless plants produce sperm equipped with flagella that enable them to swim in a moist environment to the archegonia: the female gametangium. The embryo develops inside the archegonium as the sporophyte. Gametangia are prominent in seedless plants, but are very rarely found in seed plants.
The sporophyte bears the sporangia (singular, sporangium): organs that first appeared in the land plants. The term “sporangia” literally means “spore in a vessel,” as it is a reproductive sac that contains spores (Figure \(\PageIndex{2}\)). The spores are later released by the sporangia and disperse in the environment. Two different types of spores are produced in land plants, the megaspore (female) and the microspore (male), resulting in the separation of sexes at different points in the lifecycle.

Seedless Plants
The sporophyte of seedless plants is diploid and results from fusion of two gametes. Seedless non-vascular plants produce only one kind of spore and are called homosporous. The gametophyte phase is dominant in these plants. After germinating from a spore, the resulting gametophyte produces both male and female gametangia, usually on the same individual. In contrast, heterosporous plants produce two morphologically different types of spores. The male spores are called microspores, because of their smaller size, and develop into the male gametophyte; the comparatively larger megaspores develop into the female gametophyte. Heterospory is observed in a few seedless vascular plants and in all seed plants.
When the haploid spore germinates in a hospitable environment, it generates a multicellular gametophyte by mitosis. The gametophyte supports the zygote formed from the fusion of gametes and the resulting young sporophyte (vegetative form). The cycle then begins anew.
Seed Plants
Gymnosperms are seed plants that use an exposed seed to reproduce. This contrasts to angiosperms, or flowering plants, that enclose the seeds in fruits or nuts. The life cycle of gymnosperm alternates between sporophyte (2n) and gametophyte (1n) stages. The bulk of their lives are spent in the sporophyte stage, but while they do the organism hosts a female gametophyte. You can think of it as the sex organs of the plant, or its ovaries (Figure \(\PageIndex{3}\)). All gymnosperms are heterosporous. The male and female reproductive organs can form in cones or strobili. Male and female sporangia are produced either on the same plant, described as monoecious (“one home” or bisexual), or on separate plants, referred to as dioecious (“two homes” or unisexual).

Figure \(\PageIndex{3}\): At what stage does the diploid zygote form?
- When the female cone begins to bud from the tree
- At fertilization
- When the seeds drop from the tree
- When the pollen tube begins to grow
- Answer
-
B. The diploid zygote forms after the pollen tube has finished forming, so that the male generative nuclei can fuse with the female gametophyte.
In this 6-minute video, explore the long-distance pollination process of naked seed plants, the gymnosperms.
Questions after watching: What is the difference between male cones and female cones in a pine tree? If the gametes of pine trees are inside cones, how do the female and male gametes meet to achieve fertilization?
The adult, or sporophyte, phase is the main phase of an angiosperm’s life cycle (Figure \(\PageIndex{4}\)). Like gymnosperms, angiosperms are heterosporous. Therefore, they generate microspores, which will generate pollen grains as the male gametophytes, and megaspores, which will form an ovule that contains female gametophytes. Inside the anthers’ microsporangia, male gametophytes divide by meiosis to generate haploid microspores, which, in turn, undergo mitosis and give rise to pollen grains. Each pollen grain contains two cells: one generative cell that will divide into two sperm and a second cell that will become the pollen tube cell. The ovary contains the female megasporangia which will give rise to the haploid megaspores.
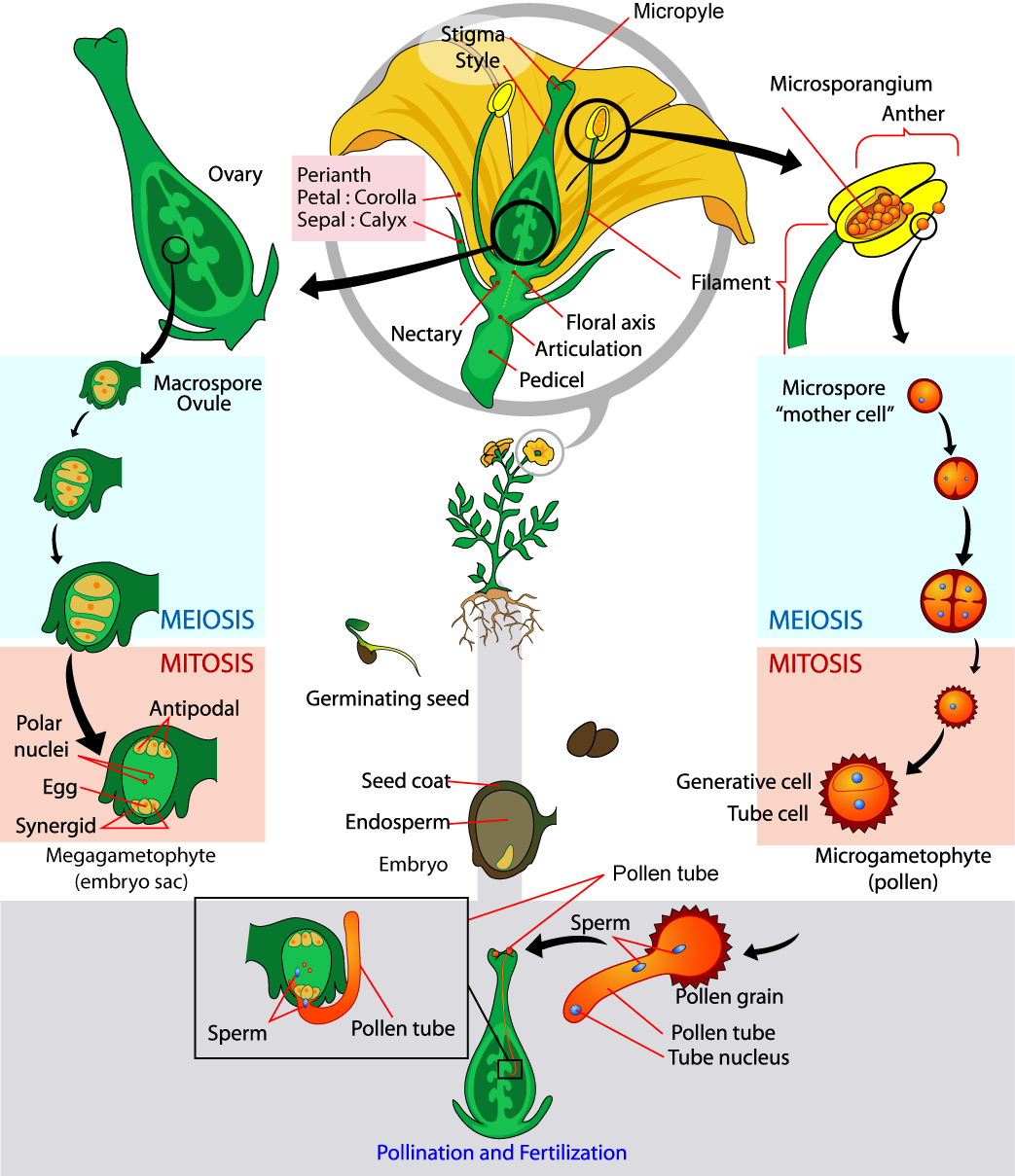
Hank gets into the dirty details about vascular plant reproduction: they use the basic alternation of generations developed by nonvascular plants 470 million years ago, but they've tricked it out so that it works a whole lot different compared to the way it did back in the Ordovician swamps where it got its start.
Apical Meristems
Shoots and roots of plants increase in length through rapid cell division in a tissue called the apical meristem, which is a small zone of cells found at the shoot tip or root tip (Figure \(\PageIndex{5}\)). The apical meristem is made of undifferentiated cells that continue to proliferate throughout the life of the plant. Elongation of the shoots and roots allows a plant to access additional space and resources: light in the case of the shoot, and water and minerals in the case of roots. A separate meristem, called the lateral meristem, produces cells that increase the diameter of tree trunks.

Additional Land Plant Adaptations
Seeds and Pollen
Protection of the embryo is a major requirement for land plants. The vulnerable embryo must be sheltered from desiccation and other environmental hazards. In both seedless and seed plants, the female gametophyte provides protection and nutrients to the embryo as it develops into the new generation of sporophyte. This distinguishing feature of land plants gave the group its alternate name of embryophytes.
Unlike bryophyte and fern spores (which are haploid cells dependent on moisture for rapid development of gametophytes), seeds contain a diploid embryo that will germinate into a sporophyte. Storage tissue to sustain growth and a protective coat give seeds their superior evolutionary advantage. Several layers of hardened tissue prevent desiccation, and free reproduction from the need for a constant supply of water. Furthermore, seeds remain in a state of dormancy, induced by desiccation and the hormone abscisic acid, until conditions for growth become favorable. Whether blown by the wind, floating on water, or carried away by animals, seeds are scattered in an expanding geographic range, thus avoiding competition with the parent plant.
Pollen grains are male gametophytes and are carried by wind, water, or a pollinator. The whole structure is protected from desiccation and can reach the female organs without dependence on water, a major evolutionary development for land-based plants. Male gametes reach female gametophyte and the egg cell gamete though a pollen tube: an extension of a cell within the pollen grain. The sperm of modern gymnosperms lack flagella, but in cycads and the Gingko, the sperm still possess flagella that allow them to swim down the pollen tube to the female gamete; however, they are enclosed in a pollen grain.
Cuticle
In land plants, a waxy, waterproof cover called a cuticle protects the leaves and stems from desiccation. However, the cuticle also prevents intake of carbon dioxide needed for the synthesis of carbohydrates through photosynthesis. To overcome this, stomata, or pores that open and close to regulate gases and water vapor, appeared in plants as they moved away from moist environments into drier habitats.
UVB Filters
Water filters ultraviolet-B (UVB) light, which is harmful to all organisms, especially those that must absorb light to survive. This filtering does not occur for land plants. This presented an additional challenge to land colonization, which was met by the evolution of biosynthetic pathways for the synthesis of protective flavonoids and other compounds: pigments that absorb UV wavelengths of light and protect the aerial parts of plants from photodynamic damage.
Secondary Metabolites
Plants cannot avoid being eaten by animals. Instead, they synthesize a large range of poisonous secondary metabolites: complex organic molecules such as alkaloids, whose noxious smells and unpleasant taste deter animals. These toxic compounds can also cause severe diseases and even death, thus discouraging predation. Humans have used many of these compounds for centuries as drugs, medications, or spices. In contrast, as plants co-evolved with animals, the development of sweet and nutritious metabolites lured animals into providing valuable assistance in dispersing pollen grains, fruit, or seeds. Plants have been enlisting animals to be their helpers in this way for hundreds of millions of years.
Vascular Tissue: Xylem and Phloem
As plants adapted to dry land and evolved strategies for dealing with the dry environment, new organs and structures made their appearance. Early land plants did not grow more than a few inches off the ground, competing for light on these low mats. By developing a shoot and growing taller, individual plants captured more light. Because air offers substantially less support than water, land plants incorporated more rigid molecules in their stems (and later, tree trunks). In small plants such as single-celled algae, simple diffusion suffices to distribute water and nutrients throughout the organism. However, for plants to evolve larger forms, the evolution of vascular tissue for the distribution of water and solutes was a prerequisite. The vascular system contains xylem and phloem tissues. Xylem conducts water and minerals absorbed from the soil up to the shoot, while phloem transports food derived from photosynthesis throughout the entire plant. A root system evolved to take up water and minerals from the soil and to anchor the increasingly taller shoot in the soil.
Roots: Support for the Plant
Roots are not well preserved in the fossil record. Nevertheless, it seems that roots appeared later in evolution than vascular tissue. The development of an extensive network of roots represented a significant new feature of vascular plants. Thin rhizoids attached bryophytes to the substrate, but these rather flimsy filaments did not provide a strong anchor for the plant; neither did they absorb substantial amounts of water and nutrients. In contrast, roots, with their prominent vascular tissue system, transfer water and minerals from the soil to the rest of the plant. The extensive network of roots that penetrates deep into the soil to reach sources of water also stabilizes trees by acting as a ballast or anchor. The majority of roots establish a symbiotic relationship with fungi, forming mycorrhizae, which benefit the plant by greatly increasing the surface area for absorption of water and soil minerals and nutrients.
Leaves, Sporophylls, and Strobili
The appearance of true leaves in the pterophytes improved plants' photosynthetic efficiency. Leaves capture more sunlight with their increased surface area by employing more chloroplasts to trap light energy and convert it to chemical energy, which is then used to fix atmospheric carbon dioxide into carbohydrates. The carbohydrates are exported to the rest of the plant by the conductive cells of phloem tissue.
In addition to photosynthesis, leaves play another role in the life of plants. Pine cones, mature fronds of ferns, and flowers are all sporophylls, leaves that were modified structurally to bear sporangia. Strobili are cone-like structures that contain sporangia. They are prominent in conifers and are commonly known as pine cones.
Flowers and Fruits
Angiosperms produce their gametes in separate organs, which are usually housed in a flower (Figure \(\PageIndex{6}\)). Both fertilization and embryo development take place inside this anatomical structure that provides a stable system of sexual reproduction largely sheltered from environmental fluctuations. Flowering plants are the most diverse phylum on Earth after insects; flowers come in a bewildering array of sizes, shapes, colors, smells, and arrangements. Most flowers have a mutualistic pollinator, with the distinctive features of flowers reflecting the nature of the pollination agent. The relationship between pollinator and flower characteristics is one of the great examples of coevolution.

Following fertilization, the ovule grows into a seed. The surrounding tissues of the ovary thicken, developing into a fruit that will protect the seed inside and often ensure its dispersal over a wide geographic range. Not all fruits develop from an ovary; such structures are “false fruits.” Like flowers, fruit can vary tremendously in appearance, size, smell, and taste. Tomatoes, walnut shells, and avocados are all examples of fruit. As with pollen and seeds, fruits also act as agents of dispersal. Some may be carried away by the wind. Many attract animals that will eat the fruit and pass the seeds through their digestive systems, then deposit the seeds in another location. Cockleburs are covered with stiff, hooked spines that can hook into fur (or clothing) and hitch a ride on an animal for long distances. The cockleburs that clung to the velvet trousers of an enterprising Swiss hiker, George de Mestral, inspired his invention of the loop and hook fastener he named Velcro.
Besides the seed, what other major structure diminishes a plant’s reliance on water for reproduction?
- flower
- fruit
- pollen
- spore
- Answer
-
C. pollen
How organisms acquired traits that allow them to colonize new environments—and how the contemporary ecosystem is shaped—are fundamental questions of evolution. Paleobotany (the study of extinct plants) addresses these questions through the analysis of fossilized specimens retrieved from field studies, reconstituting the morphology of organisms that disappeared long ago. Paleobotanists trace the evolution of plants by following the modifications in plant morphology: shedding light on the connection between existing plants by identifying common ancestors that display the same traits. This field seeks to find transitional species that bridge gaps in the path to the development of modern organisms. Fossils are formed when organisms are trapped in sediments or environments where their shapes are preserved. Paleobotanists collect fossil specimens in the field and place them in the context of the geological sediments and other fossilized organisms surrounding them. The activity requires great care to preserve the integrity of the delicate fossils and the layers of rock in which they are found.
One of the most exciting recent developments in paleobotany is the use of analytical chemistry and molecular biology to study fossils. Preservation of molecular structures requires an environment free of oxygen, since oxidation and degradation of material through the activity of microorganisms depend on its presence. One example of the use of analytical chemistry and molecular biology is the identification of oleanane, a compound that deters pests. Up to this point, oleanane appeared to be unique to flowering plants; however, it has now been recovered from sediments dating from the Permian, much earlier than the current dates given for the appearance of the first flowering plants. Paleobotanists can also study fossil DNA, which can yield a large amount of information, by analyzing and comparing the DNA sequences of extinct plants with those of living and related organisms. Through this analysis, evolutionary relationships can be built for plant lineages.
Some paleobotanists are skeptical of the conclusions drawn from the analysis of molecular fossils. For example, the chemical materials of interest degrade rapidly when exposed to air during their initial isolation, as well as in further manipulations. There is always a high risk of contaminating the specimens with extraneous material, mostly from microorganisms. Nevertheless, as technology is refined, the analysis of DNA from fossilized plants will provide invaluable information on the evolution of plants and their adaptation to an ever-changing environment.
How did the development of a vascular system contribute to the increase in size of plants?
- Answer
-
Plants became able to transport water and nutrients and not be limited by rates of diffusion. Vascularization allowed the development of leaves, which increased efficiency of photosynthesis and provided more energy for plant growth.
Sweetgrass (Hierochloe odorata) is an aromatic angiosperm that belongs to the Order Poales, which contains many of the grasses and sedges. It is a hardy perennial monocot that is found from the plains of North America to the Arctic Circle. As an herbaceous plant, it grows to approximately 100 cm and reproduces primarily vegetatively through rhizomes, modified stems that grow underground (Figure \(\PageIndex{7}\)).

"Sweetgrass is revered as a sacred plant. For many it is a cultural keystone species, reflecting a group’s cultural identity and embodying their values and beliefs. Sweetgrass leaves at the harvestable stage are bright green, and, depending on when they are picked and how quickly they are dried, turn light green to straw-coloured. For weaving into baskets, the dried leaves are soaked, and then coiled or twined, alone or with other materials as the foundation. Experienced weavers often mix sweetgrass dried in different ways to create subtle designs, or add a few dyed leaves as coloured decorations.
On the Great Plains of southern Alberta, the Siksika and their relatives have many uses for sweetgrass, which they call sipátsimo. They use it as a ceremonial incense during daily prayers, burn it at major tribal events such as the Sundance, and use the smoke to purify tribal dancers. Siksika also used sweetgrass leaves to treat saddle sores on their horses, packed braided sweetgrass leaves into saddles, and fed their horses sweetgrass to give them stamina. They also inhaled sweetgrass smoke or drank an infusion of the plant for colds, coughs and sore throats, and used water in which the stems had been soaked as an eyewash.
Sweetgrass was used to make a hair wash and hair tonic, not only by the Siksika, but by the Nlaka’pamux of British Columbia, who call sweetgrass xásxast (literally, ‘good-good’). The Nlaka’pamux were said to have traded for sweetgrass braids from the Siksika. Other Interior Salish Peoples, as well as the Ktunaxa of British Columbia, also use sweetgrass for ceremonial and medicinal purposes. On the far West Coast of Canada, the Nuxalk have been using sweetgrass from the estuary of the Bella Coola River for ceremonial purposes for generations; according to some, they also learned about using sweetgrass from the Siksika, who were said to have visited the Nuxalk at some point in the early 20th century."
In this 16-minute video, you will be introduced to the usage and cultural significance of sweetgrass for the Mi'kmaw culture. You will also be introduced to natural history of sweetgrass.
Question after watching: What practice did the indigenous population engaged in that might have contributed to the spread of sweetgrass?
Adapted from: Turner, N. (2018). Sweetgrass. In The Canadian Encyclopedia. Retrieved from https://www.thecanadianencyclopedia.ca/en/article/sweetgrass
Biodiversity of Plants
Biodiversity ensures a resource for new food crops and medicines. Plant life balances ecosystems, protects watersheds, mitigates erosion, moderates climate and provides shelter for many animal species. Threats to plant diversity, however, come from many angles. The explosion of the human population, especially in tropical countries where birth rates are highest and economic development is in full swing, is leading to human encroachment into forested areas. To feed the larger population, humans need to obtain arable land, so there is massive clearing of trees. The need for more energy to power larger cities and economic growth therein leads to the construction of dams, the consequent flooding of ecosystems, and increased emissions of pollutants. Other threats to tropical forests come from poachers, who log trees for their precious wood. Ebony and Brazilian rosewood, both on the endangered list, are examples of tree species driven almost to extinction by indiscriminate logging.
The number of plant species becoming extinct is increasing at an alarming rate. Because ecosystems are in a delicate balance, and seed plants maintain close symbiotic relationships with animals—whether predators or pollinators—the disappearance of a single plant can lead to the extinction of connected animal species. A real and pressing issue is that many plant species have not yet been cataloged, and so their place in the ecosystem is unknown. These unknown species are threatened by logging, habitat destruction, and loss of pollinators. They may become extinct before we have the chance to begin to understand the possible impacts from their disappearance.
Efforts to preserve biodiversity take several lines of action, from preserving heirloom seeds to barcoding species. Heirloom seeds come from plants that were traditionally grown in human populations, as opposed to the seeds used for large-scale agricultural production. Barcoding is a technique in which one or more short gene sequences, taken from a well-characterized portion of the genome, are used to identify a species through DNA analysis.Thumbnail: Sunflower (Sunfola variety) against a blue sky. (CC BY-NC 3.0 / cropped from original; Fir0002/Flagstaffotos via Wikipedia).