5.2: The Ability to Adhere to Host Cells and Resist Physical Removal
- Page ID
- 3161
- Briefly describe 3 different mechanisms by which bacteria can adhere to host cells and colonize and state how this can promote colonization.
- State an advantage for bacteria in being able to switch the adhesive tips of their pili.
- Define biofilm and state at least 3 benefits associated with bacteria living as a community within a biofilm.
Highlighted Bacterium
- Read the description of Neisseria memingitidis andmatch the bacterium with the description of the organism and the infection it causes.
One of the body's innate immune defenses is the ability to physically remove bacteria from the body through such means as the constant shedding of surface epithelial cells from the skin and mucous membranes, the removal of bacteria by such means as coughing, sneezing, vomiting, and diarrhea, and bacterial removal by bodily fluids such as saliva, blood, mucous, and urine. Bacteria may resist this physical removal by producing pili, cell wall adhesin proteins, and/or biofilm-producing capsules. In addition, the physical attachment of bacteria to host cells can also serve as a signal for the activation of genes involved in bacterial virulence. This process is known as signal transduction.
Using Pili (fimbriae) to Adhere to Host Cells
As seen in Unit 1, pili enable some organisms to adhere to receptors on target host cells (Figure \(\PageIndex{5}\).2.1) and thus colonize and resist flushing by the body. Pili are thin, protein tubes originating from the cytoplasmic membrane and are found in virtually all Gram-negative bacteria, but not in many Gram-positive bacteria.
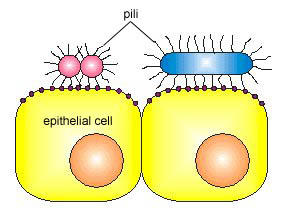
The pilus has a shaft composed of a protein called pilin. At the end of the shaft is the adhesive tip structure having a shape corresponding to that of specific glycoprotein or glycolipid receptors on a host cell (Figure \(\PageIndex{5}\).2.3). Because both the bacteria and the host cells have a negative charge, pili may enable the bacteria to bind to host cells without initially having to get close enough to be pushed away by electrostatic repulsion. Once attached to the host cell, the pili can depolymerize and enable adhesions in the bacterial cell wall to make more intimate contact. There is also evidence that the binding of pili to host cell receptors can serve as a trigger for activating the synthesis of some cell wall adhesins.
Bacteria are constantly losing and reforming pili as they grow in the body and the same bacterium may switch the adhesive tips of the pili in order to adhere to different types of cells and evade immune defenses (Figure \(\PageIndex{2}\).2.3). E. coli, for example, is able to make over 30 different types of pili.
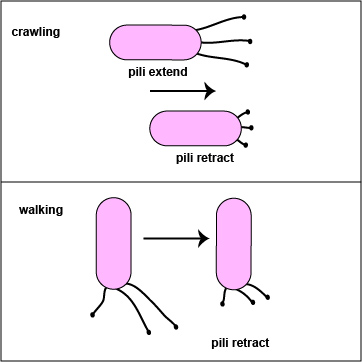
One class of pili, known as type IV pili, not only allows for attachment but also enable a twitching motility. They are located at the poles of bacilli and allow for a gliding motility along a solid surface such as a host cell. Extension and retraction of these pili allows the bacterium to drag itself along the solid surface (Figure \(\PageIndex{4}\)). In addition, bacteria can use their type IV pili to "slingshot" the bacterium over a cellular surface. In this case, as the pili contract they are thought to become taut like a stretched rubber band. When an anchoring pilus detaches, the taut pili "slingshot" the bacterium in the opposite direction (Figure \(\PageIndex{5}\)). This motion typically alternates with the twitching motility and enables a more rapid motion and direction change than with the twitching motility because the rapid slingshotting motion reduces the viscosity of the surrounding biofilm.
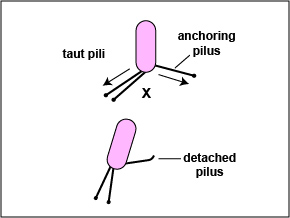
This enables bacteria with these types of pili within a biofilm to move around a cellular surface and find an optimum area on that cell for attachment and growth once they have initially bound. Bacteria with type IV pili include Pseudomonas aeruginosa, Neisseria gonorrhoeae, Neisseria meningitidis, and Vibrio cholerae.
Examples of bacteria using pili to colonize:
1. To cause infection, Neisseria gonorrhoeae must first colonize a mucosal surface composed of columnar epithelial cells. Pili allow for this initial binding and, in fact, N. gonorrhoeae is able to rapidly lose pili and synthesize new ones with a different adhesive tip, enabling the bacterium to adhere to a variety of tissues and cells including sperm, the epithelial cells of the mucous membranes lining the throat, genitourinary tract, rectum, and the conjunctiva of the eye. Subsequently, the bacterium is able to make more intimate contact with the host cell surface by way of a cell wall adhesin called Opa (see below).
2. The pili of Neisseria meningitidis allow it to adhere to mucosal epithelial cells in the nasopharynx where it is often asymptomatic. From there, however, it sometimes enters the blood and meninges and causes septicemia and meningitis. Type IV pili are thought to help the bacterium cross the blood brain barrier.
Click on this link, read the description of Neisseria meningitidis, and be able to match the bacterium with its description on an exam.
3. Uropathogenic strains of Escherichia coli can produce pili that enable the bacterium to adhere to the urinary epithelium and cause urinary tract infections. They also produce afimbrial adhesins (see below) for attachment to epithelial cells. Enteropathogenic E. coli (EPEC) use pili to adhere to intestinal mucosal cells.
- To view an electron micrograph E. coli with pili, see Dennis Kunkel's Microscopy at the University of Hawaii-Manoa.
- To view electron micrographs of enteropathogenic E. coli (EPEC) adhering to intestinal cells, see Donnenberg Lab Images at the University of Maryland Medical School.
4. Pili of Vibrio cholerae allow it to adhere to cells of the intestinal mucosa and resist the flushing action of diarrhea.
5. Pili of Pseudomonas aeruginosa allow it to initially colonize wounds or the lung.
Using Adhesins to Adhere to Host Cells
Adhesins are surface proteins found in the cell wall of various bacteria that bind to specific receptor molecules on the surface of host cells and enable the bacterium to adhere intimately to that cell in order to colonize and resist physical removal (Figure \(\PageIndex{6}\)). Many, if not most bacteria probably use one or more adhesins to colonize host cells.
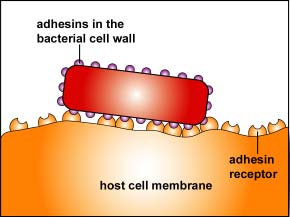
For example:
1. Streptococcus pyogenes (see electron micrograph) (group A beta streptococci) produce a number of adhesins
a. Protein F that binds to fibronectin , a common protein on epithelial cells. In this way it is able to adhere to the lymphatics and mucous membranes of the upper respiratory tract and cause streptococcal pharyngitis (strep throat).
b. Lipoteichoic acid binds to fibronectin on epithelial cells.
c. M-protein also functions as an adhesin.
2. The tip of the spirochete Treponema pallidum contains adhesins that are able to bind to fibronectin on epithelial cells.
Scanning electron Micrograph of T. pallidum adhering to a host cell by its tip.
3. The tip of the spirochete Borrelia burgdorferi contains adhesins that can bind to various host cells.
4. Escherichia coli O157 utilizes a type 3 secretion system to inject effector proteins into intestinal epithelial cells. Some of these cause polymerization of actin at the cell surface and this pushes the host cell cytoplasmic membrane up to form a pedestal. Another effector protein inserts into the membrane of the pedestal to serve as a receptor molecule for E. coli adhesins (Figure \(\PageIndex{7}\)).
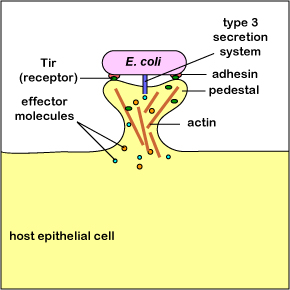
5. Helicobacter pylori use a type 4 secretion system to inject effector proteins into stomach epithelial cells to induce these host cells to display more receptors on their surface for H. pylori adhesins.
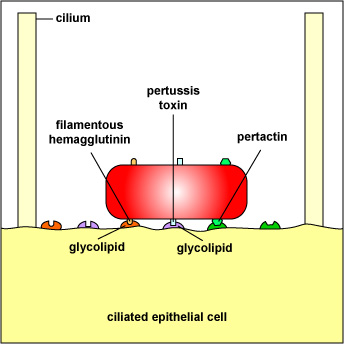
6. Bordetella pertussis produces several adhesins (Figure \(\PageIndex{8}\)):
a. Filamentous hemagglutinin is an adhesin that allows the bacterium to adhere to galactose residues of the glycolipids on the membrane of ciliated epithelial cells of the respiratory tract.
b. Pertussis toxin also functions as an adhesin. One subunit of the pertussis toxin remains bound to the bacterial cell wall while another subunit binds to the glycolipids on the membrane of ciliated epithelial cells of the respiratory tract.
c. B. Pertussis also produces an adhesin called pertactin that further enables the bacterium to adhere to cells.
7. Neisseria gonorrhoeae produces an adhesin called Opa (protein II) that enables the bacterium to make a more intimate contact with the host cell after it first adheres with its pili. Like with adhesive tips of pili, N. gonorrhoeae has multiple alleles for Opa protein adhesins enabling the bacterium to adhere to a variety of host cell types.
8. Staphylococcus aureus uses protein A as an adhesin to adhere to various host cells. It also helps the bacterium to resist phagocytosis.
Using Biofilms to Adhere to Host Cells
Many normal flora bacteria produce a capsular polysaccharide matrix or glycocalyx to form a biofilm on host tissue. Biofilms are groups of bacteria attached to a surface and enclosed in a common secreted adhesive matrix, typically polysaccharide in nature. Many pathogenic bacteria, as well as normal flora and many environmental bacteria, form complex bacterial communities as biofilms.
Bacteria in biofilms are often able to communicate with one another by a process called quorum sensing and are able to interact with and adapt to their environment as a population of bacteria rather than as individual bacteria. By living as a community of bacteria as a biofilm, these bacteria are better able to:
- resist attack by antibiotics;
- trap nutrients for bacterial growth and remain in a favorable niche;
- adhere to environmental surfaces and resist flushing;
- live in close association and communicate with other bacteria in the biofilm; and
- resist phagocytosis and attack by the body's complement pathways.
Biofilms are, therefore, functional, interacting, and growing bacterial communities. Biofilms even contain their own water channels for delivering water and nutrients throughout the biofilm community.
- Electron micrograph of a biofilm of Haemophilus influenzae from Biomedcentral.com
- Photomicrograph of a biofilm with water channels from Centers for Disease Control and Prevention Rodney M. Donlan: "Biofilms: Microbial Life on Surfaces"
- Biofilm of Pseudomonas aeruginosa from the Ausubel Lab, Department of Molecular Biology, Massachusetts General Hospital
- Scanning electron micrograph of Staphylococcus aureus forming a biofilm in an indwelling catheter courtesy of CDC.
- Biofilm of Staphylococcus aureus from Montana State University
For example:
1. Streptococcus mutans, and Streptococcus sobrinus , two bacteria implicated in initiating dental caries, break down sucrose into glucose and fructose. Streptococcus mutans can uses an enzyme called dextransucrase to convert sucrose into a sticky polysaccharide called dextran that forms a biofilm enabling the bacteria to adhere to the enamel of the tooth and initiate plaque formation.
This dextran mesh traps the S. mutans and S. sobrinus, along with other bacteria and debris, and forms plaque. S. mutans and S. sobrinus also ferment glucose in order to produce energy. The fermentation of glucose results in the production of lactic acid that is released onto the surface of the tooth and initiates decay.
- Scanning electron micrograph of Streptococcus growing in the enamel of a tooth.© Lloyd Simonson, author. Licensed for use, ASM MicrobeLibrary.
- Scanning electron micrograph of dental plaque.© H. Busscher, H. van der Mei, W. Jongebloed, R Bos, authors. Licensed for use, ASM MicrobeLibrary.
2. Most children suffering from chronic ear infection (otitis media) have a biofilm of bacteria in their middle ear. This biofilm contains bacteria such as Streptococcus pneumoniae, Haemophilus influenzae, and Moraxella catarrhalis and enables the bacteria to chronically colonize the middle ear as well as resist body defenses and antibiotics.
3. Planktonic Pseudomonas aeruginosa uses its polar flagellum to move through water or mucus and make contact with a solid surface such as the body's mucous membranes. It then can use pili and cell wall adhesins to attach to the epithelial cells of the mucous membrane. Attachment activates signaling and quorum sensing genes to eventually enable the population of P. aeruginosa to start synthesizing a polysaccharide biofilm composed of alginate. As the biofilm grows, the bacteria lose their flagella to become nonmotile and secrete a variety of enzymes that enable the population to obtain nutrients from the host cells. Eventually the biofilm mushrooms up and develops water channels to deliver water and nutrients to all the bacteria within the biofilm. As the biofilm begins to get too crowded with bacteria, quorum sensing enables some of the Pseudomonas to again produce flagella, escape the biofilm, and colonize a new location (See Figs. 9A-9H).
Many chronic and difficult-to-treat infections are caused by bacteria in biofilms. Within biofilms, bacteria grow more slowly, exhibit different gene expression than free planktonic bacteria, and are more resistant to antimicrobial agents such as antibiotics because of the reduced ability of these chemicals to penetrate the dense biofilms matrix. Biofilms have been implicated in tuberculosis, kidney stones, Staphylococcus infections, Legionnaires' disease, and periodontal disease. It is further estimated that as many as 10 million people a year in the US may develop biofilm-associated infections as a result of invasive medical procedures and surgical implants.
- Scanning electron micrograph of Listeria growing on a stainless steel surface. © Amy Lee Wong, author. Licensed for use, ASM MicrobeLibrary.
- Scanning electron micrograph of Pseudomonas growing on bronchial mucosa. © Hiroyuki Kobayashi, author. Licensed for use, ASM MicrobeLibrary.
- Scanning electron micrograph of Staphylococcus aureus forming a biofilm in an indwelling catheter courtesy of CDC.
- Article and computer-generated model of biofilm formation courtesy of NIH.
YouTube movie and animation: What are Biofilms?
Pseudomonas aeruginosa, a common cause of serious respiratory infections on people with cystic fibrosis, produces a single polar flagellum, can secrete a polysaccharide slime composed of alginate, and is able to produce both pili and cell wall adhesins. How could each of these factors contribute to the bacterium's pathogenosis and in what order might they be used?
Summary
- One of the body's innate immune defenses is the ability to physically remove bacteria from the body.
- Bacteria may resist physical removal by producing pili, cell wall adhesin proteins, and/or biofilm-producing capsules that enable bacteria to adhere to host cells.
- At the end of the shaft of a bacterial pilus is an adhesive tip structure having a shape corresponding to that of specific receptor on a host cell for initial attachment. Bacteria can typically make a variety of different adhesive tips enabling them to attach to different host cell receptors.
- Cell wall adhesins are surface proteins found in the cell wall of various bacteria that bind tightly to specific receptor molecules on the surface of host cells. Bacteria can typically make a variety of different cell wall adhesins enabling them to attach to different host cell receptors.
- Biofilms are groups of bacteria attached to a surface and enclosed in a common secreted adhesive matrix, typically polysaccharide in nature. Many pathogenic bacteria, as well as normal flora and many environmental bacteria, form complex bacterial communities as biofilms.
- Many chronic and difficult-to-treat infections are caused by bacteria in biofilms.