15.8B: Synapses
- Page ID
- 5647
The coordination of cellular activities in animals is usually considered to involve the endocrine system (where the response is to hormones: chemicals secreted into the blood by endocrine glands and carried by the blood to the responding cell) and a nervous system (response to electrical impulses passing from the central nervous system to muscles and glands). However, in fact, coordination by the nervous system is also chemical. Most neurons achieve their effect by releasing chemicals, the neurotransmitters, on a receiving cell: another neuron (a "postsynaptic" neuron), a muscle cell and a gland cell. So the real distinction between nervous and endocrine coordination is that nervous coordination is faster and more localized. Neurotransmitters are chemicals that act in a paracrine fashion.
The junction between the axon terminals of a neuron and the receiving cell is called a synapse. Synapses at muscle fibers are also called neuromuscular junctions or myoneural junctions.
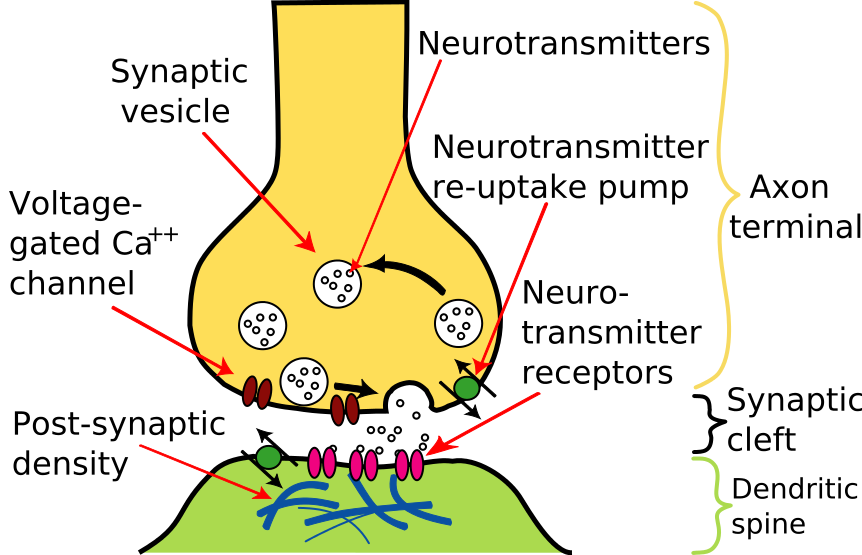
- Action potentials travel down the axon of the neuron to its end(s), the axon terminal(s).
- Each axon terminal is swollen forming a synaptic knob.
- The synaptic knob is filled with membrane-enclosed vesicles containing a neurotransmitter.
- Arrival of an action potential at the synaptic knob opens Ca2+ channels in the plasma membrane.
- The influx of Ca2+ triggers the exocytosis of some of the vesicles.
- Their neurotransmitter is released into the synaptic cleft.
- The neurotransmitter molecules bind to receptors on the postsynaptic membrane.
- These receptors are ligand-gated ion channels.
Excitatory synapses
The neurotransmitter at excitatory synapses depolarizes the postsynaptic membrane (of a neuron in this diagram). Example: acetylcholine (ACh)
- Binding of acetylcholine to its receptors on the postsynaptic cell opens up ligand-gated sodium channels.
- These allow an influx of Na+ ions, reducing the membrane potential.
- This reduced membrane potential is called an excitatory postsynaptic potential or EPSP.
- If depolarization of the postsynaptic membrane reaches threshold, an action potential is generated in the postsynaptic cell.
Inhibitory synapses
The neurotransmitter at inhibitory synapses hyperpolarizes the postsynaptic membrane. Example: gamma aminobutyric acid (GABA) at certain synapses in the brain.
The GABAA receptor is a ligand-gated chloride channel. Binding of GABA to the receptors increases the influx of chloride (Cl−) ions into the postsynaptic cell raising its membrane potential and thus inhibiting it. (The neurotransmitter glycine acts in the same way at synapses in the spinal cord and the base of the brain.) This is a fast response - taking only about 1 millisecond. Binding of GABA to GABAB receptors activates an internal G protein and a "second messenger" that leads to the opening of nearby potassium (K+) channels. As you might expect, this is a slower response, taking as long as 1 second.
In both cases, the resulting facilitated diffusion of ions (chloride IN; potassium OUT) increases the membrane potential (to as much as −80 mv). This increased membrane potential is called an inhibitory postsynaptic potential (IPSP) because it counteracts any excitatory signals that may arrive at that neuron. A hyperpolarized neuron appears to have an increased threshold. Actually, the threshold voltage (about −50 mv) has not changed. It is simply a question of whether the depolarization produced by excitatory synapses on the cell minus the hyperpolarizing effect of inhibitory synapses can reach this value or not.
Neurotransmitters
Acetylcholine (ACh)
Widely used at synapses in the peripheral nervous system. Released at the terminals of
- all motor neurons activating skeletal muscle.
- all preganglionic neurons of the autonomic nervous system.
- the postganglionic neurons of the parasympathetic branch of the autonomic nervous system.
Also mediates transmission at some synapses in the brain. These include synapses involved in the acquisition of short-term memory. Drugs that enhance ACh levels - acetylcholinesterase inhibitors - are now used in elderly patients with failing memory (e.g., Alzheimer's patients).
Nicotinic vs. Muscarinic Acetylcholine Receptors
ACh acts on two different types of receptor:
- Nicotinic receptors are
- found at the neuromuscular junction of skeletal (only) muscles
- on the post-ganglionic neurons of the parasympathetic nervous system
- on many neurons in the brain (e.g. neurons in the hypothalamus whose activation by nicotine suppresses appetite)
- Nicotine is an agonist (hence the name)
- Curare is an antagonist (hence its ability to paralyze skeletal muscles)
- Muscarinic receptors are
- Found at the neuromuscular junctions of cardiac and smooth muscle as well as on glands and on the post-ganglionic neurons of the sympathetic nervous system.
- Muscarine (a toxin produced by certain mushrooms) is an agonist.
- Atropine is an antagonist (hence its use in acetylcholinesterase poisoning).
Amino acids
- Glutamic acid (Glu); used at excitatory synapses in the central nervous system (CNS). Essential for long term potentiation (LTP), a form of memory.
Like GABA, Glu acts on two types of CNS synapses:- FAST (~1 msec) with Glu opening ligand-gated Na+ channels;
- SLOW (~1 sec) with Glu binding to G-protein-coupled receptors receptors that turn on a "second messenger" cascade of biochemical changes that open channels allowing Na+ into the cell.
- Gamma aminobutyric acid (GABA); used at inhibitory synapses in the CNS.
- Glycine (Gly). Also used at inhibitory synapses in the CNS. In fact, both GABA and glycine are released together at some inhibitory synapses.
Catecholamines
Synthesized from tyrosine (Tyr).
- Noradrenaline (also called norepinephrine). Released by postganglionic neurons of the sympathetic branch of the autonomic nervous system. Also used at certain synapses in the CNS.
- Dopamine. Used at certain synapses in the CNS.
Other monoamines
- Serotonin (also known as 5-hydroxytryptamine or 5HT). Synthesized from tryptophan (Trp).
- Histamine
Both of these neurotransmitters are confined to synapses in the brain. However, serotonin is also secreted from the duodenum, where it acts in a paracrine manner to stimulate intestinal peristalsis, and as a circulating hormone, where it is taken up by platelets and also suppresses bone formation.
Peptides
A selection of 9 of the 40 or more peptides that are suspected to serve as neurotransmitters in the brain. The first six also serve as hormones.
- Vasopressin
- Oxytocin
- Somatostatin
- Gonadotropin-releasing hormone (GnRH)
- Angiotensin II
- Cholecystokinin (CCK)
- Substance P
- Two enkephalins
- Met-enkephalin (Tyr-Gly-Gly-Phe-Met)
- Leu-enkephalin (Tyr-Gly-Gly-Phe-Leu)
ATP
ATP - probably along with another neurotransmitter is released at some synapses in the brain.
Synaptic Plasticity
Most neurons release a single neurotransmitter at the synapses at their axon terminals. However, some exceptions have been found.
- neurons that release one transmitter at some of their terminals, a different one at others
- neurons that switch from one neurotransmitter to a different one when the stimulus reaching them changes. Example: interneurons in the hypothalamus of the rat that release dopamine when the rats are exposed to short-day photoperiods (which these nocturnal animals like) but switch to releasing somatostatin when the rats are exposed to long days (which they don't like).
Turning Synapses Off
Once its job is done, the neurotransmitter must be removed from the synaptic cleft to prepare the synapse for the arrival of the next action potential. Two methods are used:
- Reuptake. The neurotransmitter is taken back into the synaptic knob of the presynaptic neuron by active transport. All the neurotransmitters except acetylcholine use this method.
- Acetylcholine is removed from the synapse by enzymatic breakdown into inactive fragments. The enzyme used is acetylcholinesterase.
Nerve gases used in warfare (e.g., sarin) and the organophosphate insecticides (e.g., parathion) achieve their effects by inhibiting acetylcholinesterase thus allowing ACh to remain active. Atropine is used as an antidote because it blocks ACh muscarinic receptors.
Drugs and Synapses
Many drugs that alter mental state achieve at least some of their effects by acting at synapses.
GABA Receptors
The GABAA receptor is a ligand-gated chloride channel. Activation of the receptors increases the influx of chloride (Cl−) ions into the postsynaptic cell raising its membrane potential and thus inhibiting it. A number of drugs bind to the GABAA receptor. They bind at sites different from the spot where GABA itself binds, but increase the strength of GABA's binding to its site. Thus they enhance the inhibitory effect of GABA in the CNS. These drugs include sedatives like phenobarbital and anti-anxiety drugs like Valium, Librium, Halcion (all members of a group called benzodiazepines). In view of their common action, it is not surprising that they act additively; taken together these drugs can produce dangerous overdoses. The recreational (and illegal) drug γ-hydroxybutyrate binds to the GABAB receptor.
Catecholamine synapses
Many antidepressant drugs (the so-called tricyclic antidepressants like amitriptyline ["Elavil"]) interfere with the reuptake of noradrenaline and serotonin from their synapses and thus enhance their action at the synapse. The popular antidepressant fluoxetine ("Prozac"), seems to block only the reuptake of serotonin.
Dopamine synapses
One class of dopamine receptor is bound by such drugs as chlorpromazine and haloperidol. Binding of these drugs leads to increased synthesis of dopamine at the synapse and eases some of the symptoms of schizophrenia.
Synapses blocking pain signals
The two enkephalins are released at synapses on neurons involved in transmitting pain signals back to the brain. The enkephalins hyperpolarize the postsynaptic membrane thus inhibiting it from transmitting these pain signals. The ability to perceive pain is vital. However, faced with massive, chronic, intractable pain, it makes sense to have a system that decreases its own sensitivity. Enkephalin synapses provide this intrinsic pain-suppressing system.
Opiates such as heroin, morphine, codeine, and methadone bind these same receptors. This makes them excellent pain killers. However, they are also highly addictive.
- By binding to enkephalin receptors, they enhance the pain-killing effects of the enkephalins.
- A homeostatic reduction in the sensitivity of these synapses compensates for continued exposure to opiates.
- This produces tolerance, the need for higher doses to achieve the prior effect.
- If use of the drug ceases, the now relatively insensitive synapses respond less well to the soothing effects of the enkephalins, and the painful symptoms of withdrawal are produced.
Electrical Synapses
Electrical synapses are a rare exception to the general rule that neurons signal other neurons by release of chemical neurotransmitters. Some properties of electrical synapses:
- The two neurons are connected by gap junctions, the space between them being much smaller (~2 nm) than that at chemical synapses (~20 nm).
- In many cases, transmission of action potentials can pass in either direction.
- Transmission between neurons is as much as ten times faster than in chemical synapses.
- Thus electrical synapses can mediate, for example, the very rapid escape response of crayfish that encounter a threat.
- Electrical synapses in the CNS permit groups of interneurons to fire together.
- They are found in vertebrates (e.g. in the hippocampus of the brain) as well as in invertebrates like the crayfish.