13.1: Introduction to Geology
- Last updated
- Save as PDF
- Page ID
- 94320
What is Geology?
In its broadest sense, geology is the study of Earth—its interior and its exterior surface, the minerals, rocks and other materials that are around us, the processes that have resulted in the formation of those materials, the water that flows over the surface and through the ground, the changes that have taken place over the vastness of geological time, and the changes that we can anticipate will take place in the near future. Geology is a science, meaning that we use deductive reasoning and scientific methods to understand geological problems. It is, arguably, the most integrated of all of the sciences because it involves the understanding and application of all of the other sciences: physics, chemistry, biology, mathematics, astronomy, and others. But unlike most of the other sciences, geology has an extra dimension, that of time—deep time—billions of years of it. Geologists study the evidence that they see around them, but in most cases, they are observing the results of processes that happened thousands, millions, and even billions of years in the past. Those were processes that took place at incredibly slow rates—millimeters per year to centimeters per year—but because of the amount of time available, they produced massive results.
Geology is displayed on a grand scale in mountainous regions, perhaps nowhere better than the Rocky Mountains in Canada (Figure \(\PageIndex{1}\)). The peak on the right is Rearguard Mountain, which is a few kilometers northeast of Mount Robson, the tallest peak in the Canadian Rockies (3,954 meters). The large glacier in the middle of the photo is the Robson Glacier. The river flowing from Robson Glacier drains into Berg Lake in the bottom right. There are many geological features portrayed here. The sedimentary rock that these mountains are made of formed in ocean water over 500 million years ago. A few hundred million years later, these beds were pushed east for tens to hundreds of kilometers by tectonic plate convergence and also pushed up to thousands of meters above sea level. Over the past two million years this area—like most of the rest of Canada—has been repeatedly glaciated, and the erosional effects of those glaciations are obvious. The Robson Glacier is now only a small remnant of its size during the Little Ice Age of the 15th to 18th centuries, and even a lot smaller that it was just over a century ago in 1908. The distinctive line on the slope on the left side of both photos shows the elevation of the edge of the glacier a few hundred years ago. Like almost all other glaciers in the world, it receded after the 18th century because of natural climate change, is now receding even more rapidly because of human-caused climate change.
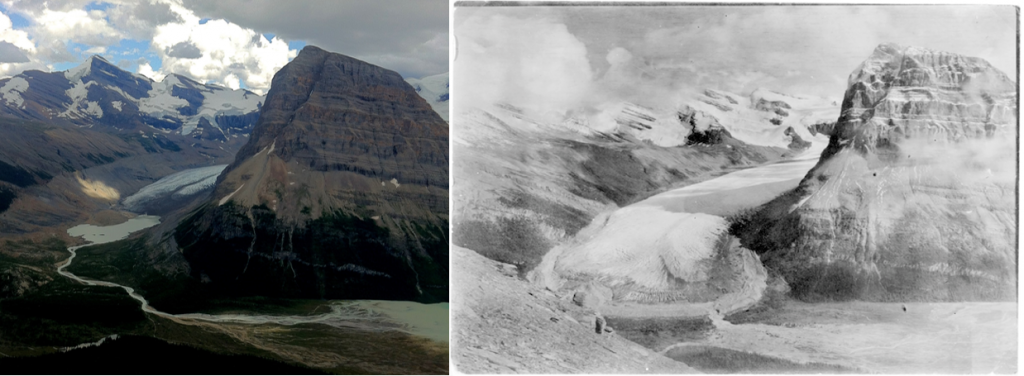
(left): © Steven Earle. CC BY. (right): A.P. Coleman. Public domain. Source: Arthur P. Coleman Collection at Victoria University Library.
How Earth Formed
The Big Bang (12-15 billion years ago) produced the atoms of hydrogen and helium. Elements as heavy as lithium followed the Big Bang within minutes. Eventually, under the pervasive influence of gravity, the hydrogen and helium were aggregated into immense masses that became increasingly compressed under enormously high pressure and temperature. When the pressure and temperature were sufficiently intense, nuclear fusion reactions began to occur within the masses, at which point they had become young stars. Stars such as red giants fused hydrogen and helium nuclei to form elements from carbon (the foundation of life) to calcium (now our bones and teeth). Supernova explosions formed and ejected heavier elements such as iron (for red blood cells). Hydrogen and helium are still, however, the most abundant elements, comprising more than 99.9% of the mass of the universe.
The Sun is an ordinary star, one of billions of billions that exist in the universe. The Sun, its eight orbiting planets, plus miscellaneous comets, meteors, asteroids, and other materials (such as space dust) are collectively known as the solar system. This particular region of the universe is organized and held together by a balance of the attractive force of gravitation and counteracting influences associated with rotation and orbiting (these same forces, along with continuing expansion from the initial big bang, also organize the universe). The age of the solar system (and of Earth) is at least 4.6 billion years. The solar system began as a rotating cloud of stardust. About 4.6 billion years ago, a nearby star exploded and sent a shock wave through the dust cloud, increasing its rate of spin. As a result, most of the mass became concentrated in the middle, forming the sun. Smaller concentrations of mass rotating around the center formed the planets, including Earth.
You can watch a video showing how Earth formed at this link:http://www.youtube.com/watch?v=-x8-KMR0nx8.
Earth is the third-closest planet to the Sun. Earth is a dense planet, as are other so-called terrestrial planets located relatively close to the Sun: Mercury, Venus, and Mars. The mass of these planets consists almost entirely of heavier elements such as iron, nickel, magnesium, aluminum, and silicon. These inner planets were formed by a selective condensing of heavier elements out of the primordial planetary nebula (the disk of gases and other matter that slowly rotated around the Sun during the early stages of formation of its solar system). This happened because the inner planets were subjected to relatively intense heating by solar radiation, which caused lighter gases such as hydrogen and helium to disperse further away, to the extent that they ended up mostly in the outer, cooler planets. Meanwhile the terrestrial planets retained heavier elements. Consequently, the more distant planets in the solar system, such as Jupiter and Saturn, are relatively large, gaseous, and diffuse in character. Most of their volume is composed of an extensive atmosphere of hydrogen and helium, although these planets may contain heavier elements in their core.
At first, Earth was molten and lacked an atmosphere and oceans. The main source of heat at that time was probably the decay of naturally-occurring radioactive elements. Gradually, the planet cooled and formed a solid crust. As the planet continued to cool, volcanoes released gases, which eventually formed an atmosphere. The early atmosphere contained ammonia, methane,water vapor, and carbon dioxide but only a trace of oxygen. As the atmosphere became denser, clouds formed and rain fell. Water from rain, and perhaps from comets and asteroidsthat struck Earth as well, eventually formed the oceans. The ancient atmosphere and oceans represented by the picture in Figure \(\PageIndex{2}\) would be toxic to today’s life, but they set the stage for life to begin.
Figure \(\PageIndex{2}\): Ancient Earth. This is how ancient Earth may have looked after its atmosphere and oceans formed.
Geological Time
In 1788, after many years of geological study, James Hutton, one of the great pioneers of geology, wrote the following about the age of Earth: The result, therefore, of our present enquiry is, that we find no vestige of a beginning — no prospect of an end.[1] Of course he wasn’t exactly correct, there was a beginning and there will be an end to Earth, but what he was trying to express is that geological time is so vast that we humans, who typically live for less than a century, have no means of appreciating how much geological time there is. Hutton didn’t even try to assign an age to Earth, but we now know that it is approximately 4,570 million years old. Using the scientific notation for geological time, that is 4,570 Ma (for mega annum or “millions of years”) or 4.57 Ga (for gigaannum or billions of years). More recent dates can be expressed in ka (kiloannum); for example, the last cycle of glaciation ended at approximately 11.7 ka or 11,700 years ago.
In order to describe the time relationships between rock formations and fossils, scientists developed a relative geologic time scale in which the earth's history is divided and subdivided into time divisions. The three eons (Phanerozoic, Proterozoic, and Archean) represent the largest time divisions (measured in billions of years). They in turn are subdivided into Eras, Periods and Epochs. Major discontinuities in the geologic record and in the corresponding biological (fossil) record are chosen as boundary lines between the different time segments. For example, the Cretaceous-Tertiary boundary (65 million years ago) marks a sudden mass extinction of species, including the dinosaurs. Through the use of modern quantitative techniques, some rocks and organic matter can be accurately dated using the decay of naturally-occurring radioactive isotopes. Therefore, absolute ages can be assigned to some parts of the geologic time scale.
A version of the geological time scale is included as Figure \(\PageIndex{3}\). Unlike time scales you’ll see in other places, or even later in this book, this time scale is linear throughout its length, meaning that 50 Ma during the Cenozoic is the same thickness as 50 Ma during the Hadean—in each case about the height of the “M” in Ma. The Pleistocene glacial epoch began at about 2.6 Ma, which is equivalent to half the thickness of the thin grey line at the top of the yellow bar marked “Cenozoic.” Most other time scales have earlier parts of Earth’s history compressed so that more detail can be shown for the more recent parts. That makes it difficult to appreciate the extent of geological time.

Figure \(\PageIndex{3}\) The geological time scale. The Hadean eon (3800 Ma to 4570 Ma), Archean eon (2500 Ma to 3800 Ma), and Proterozoic eon (542 Ma to 2500 Ma) make up 88% of geological time. The Phanerozoic eon makes up the last 12% of geological time. The Phanerozoic eon (0 Ma to 542 Ma) contains the Paleozoic, Mesozoic, and Cenozoic eras. © Steven Earle. CC BY.
To create some context, the Phanerozoic Eon (the last 542 million years) is named for the time during which visible (phaneros) life (zoi) is present in the geological record. In fact, large organisms—those that leave fossils visible to the naked eye—have existed for a little longer than that, first appearing around 600 Ma, or a span of just over 13% of geological time. Animals have been on land for 360 million years, or 8% of geological time. Mammals have dominated since the demise of the dinosaurs around 65 Ma, or 1.5% of geological time, and the genus Homo has existed since approximately 2.8 Ma, or 0.06% (1/1,600th) of geological time.
Geologists (and geology students) need to understand geological time. That doesn’t mean memorizing the geological time scale; instead, it means getting your mind around the concept that although most geological processes are extremely slow, very large and important things can happen if such processes continue for enough time.
For example, the Atlantic Ocean between Nova Scotia and northwestern Africa has been getting wider at a rate of about 2.5 centimeters (cm) per year. Imagine yourself taking a journey at that rate—it would be impossibly and ridiculously slow. And yet, since it started to form at around 200 Ma (just 4% of geological time), the Atlantic Ocean has grown to a width of over 5,000 kilometers (km)!
A useful mechanism for understanding geological time is to scale it all down into one year. The origin of the solar system and Earth at 4.57 Ga would be represented by January 1, and the present year would be represented by the last tiny fraction of a second on New Year’s Eve. At this scale, each day of the year represents 12.5 million years; each hour represents about 500,000 years; each minute represents 8,694 years; and each second represents 145 years. Some significant events in Earth’s history, as expressed on this time scale, are summarized on Table \(\PageIndex{1}\).
[Skip Table] | ||
Event | Approximate Date | Calendar Equivalent |
---|---|---|
Formation of oceans and continents | 4.5 to 4.4 Ga | January |
Evolution of the first primitive life forms | 3.8 Ga | early March |
Formation of British Columbia’s oldest rocks | 2.0 Ga | July |
Evolution of the first multi-celled animals | 0.6 Ga or 600 Ma | November 15 |
Animals first crawled onto land | 360 Ma | December 1 |
Vancouver Island reached North America and the Rocky Mountains were formed | 90 Ma | December 25 |
Extinction of the non-avian dinosaurs | 65 Ma | December 26 |
Beginning of the Pleistocene ice age | 2 Ma or 2000 ka | 8 p.m., December 31 |
Retreat of the most recent glacial ice from southern Canada | 14 ka | 11:58 p.m., December 31 |
Arrival of the first people in British Columbia | 10 ka | 11:59 p.m., December 31 |
Arrival of the first Europeans on the west coast of what is now Canada | 250 years ago | 2 seconds before midnight, December 31 |
References
- Hutton, J, 1788. Theory of the Earth; or an investigation of the laws observable in the composition, dissolution, and restoration of land upon the Globe. Transactions of the Royal Society of Edinburgh. ↵
Contributors and Attributions
Modified by Kyle Whittinghill from the following sources
- 5.4: How Earth Formed by CK-12: Biology Concepts, is licensed CC BY-NC
- What is Geology and Geeological Time from Physical Geology by Steven Earle (licensed under a Creative Commons Attribution 4.0 International License)
- The Solid Earth from AP Environmental Science by University of California College Prep
- The Physical World from Environmental Science: A Canadian Perspective by Bill Freedman (Creative Commons Attribution NonCommercial)