1.38: Virus Bioassay
- Page ID
- 79461
\( \newcommand{\vecs}[1]{\overset { \scriptstyle \rightharpoonup} {\mathbf{#1}} } \)
\( \newcommand{\vecd}[1]{\overset{-\!-\!\rightharpoonup}{\vphantom{a}\smash {#1}}} \)
\( \newcommand{\id}{\mathrm{id}}\) \( \newcommand{\Span}{\mathrm{span}}\)
( \newcommand{\kernel}{\mathrm{null}\,}\) \( \newcommand{\range}{\mathrm{range}\,}\)
\( \newcommand{\RealPart}{\mathrm{Re}}\) \( \newcommand{\ImaginaryPart}{\mathrm{Im}}\)
\( \newcommand{\Argument}{\mathrm{Arg}}\) \( \newcommand{\norm}[1]{\| #1 \|}\)
\( \newcommand{\inner}[2]{\langle #1, #2 \rangle}\)
\( \newcommand{\Span}{\mathrm{span}}\)
\( \newcommand{\id}{\mathrm{id}}\)
\( \newcommand{\Span}{\mathrm{span}}\)
\( \newcommand{\kernel}{\mathrm{null}\,}\)
\( \newcommand{\range}{\mathrm{range}\,}\)
\( \newcommand{\RealPart}{\mathrm{Re}}\)
\( \newcommand{\ImaginaryPart}{\mathrm{Im}}\)
\( \newcommand{\Argument}{\mathrm{Arg}}\)
\( \newcommand{\norm}[1]{\| #1 \|}\)
\( \newcommand{\inner}[2]{\langle #1, #2 \rangle}\)
\( \newcommand{\Span}{\mathrm{span}}\) \( \newcommand{\AA}{\unicode[.8,0]{x212B}}\)
\( \newcommand{\vectorA}[1]{\vec{#1}} % arrow\)
\( \newcommand{\vectorAt}[1]{\vec{\text{#1}}} % arrow\)
\( \newcommand{\vectorB}[1]{\overset { \scriptstyle \rightharpoonup} {\mathbf{#1}} } \)
\( \newcommand{\vectorC}[1]{\textbf{#1}} \)
\( \newcommand{\vectorD}[1]{\overrightarrow{#1}} \)
\( \newcommand{\vectorDt}[1]{\overrightarrow{\text{#1}}} \)
\( \newcommand{\vectE}[1]{\overset{-\!-\!\rightharpoonup}{\vphantom{a}\smash{\mathbf {#1}}}} \)
\( \newcommand{\vecs}[1]{\overset { \scriptstyle \rightharpoonup} {\mathbf{#1}} } \)
\( \newcommand{\vecd}[1]{\overset{-\!-\!\rightharpoonup}{\vphantom{a}\smash {#1}}} \)
\(\newcommand{\avec}{\mathbf a}\) \(\newcommand{\bvec}{\mathbf b}\) \(\newcommand{\cvec}{\mathbf c}\) \(\newcommand{\dvec}{\mathbf d}\) \(\newcommand{\dtil}{\widetilde{\mathbf d}}\) \(\newcommand{\evec}{\mathbf e}\) \(\newcommand{\fvec}{\mathbf f}\) \(\newcommand{\nvec}{\mathbf n}\) \(\newcommand{\pvec}{\mathbf p}\) \(\newcommand{\qvec}{\mathbf q}\) \(\newcommand{\svec}{\mathbf s}\) \(\newcommand{\tvec}{\mathbf t}\) \(\newcommand{\uvec}{\mathbf u}\) \(\newcommand{\vvec}{\mathbf v}\) \(\newcommand{\wvec}{\mathbf w}\) \(\newcommand{\xvec}{\mathbf x}\) \(\newcommand{\yvec}{\mathbf y}\) \(\newcommand{\zvec}{\mathbf z}\) \(\newcommand{\rvec}{\mathbf r}\) \(\newcommand{\mvec}{\mathbf m}\) \(\newcommand{\zerovec}{\mathbf 0}\) \(\newcommand{\onevec}{\mathbf 1}\) \(\newcommand{\real}{\mathbb R}\) \(\newcommand{\twovec}[2]{\left[\begin{array}{r}#1 \\ #2 \end{array}\right]}\) \(\newcommand{\ctwovec}[2]{\left[\begin{array}{c}#1 \\ #2 \end{array}\right]}\) \(\newcommand{\threevec}[3]{\left[\begin{array}{r}#1 \\ #2 \\ #3 \end{array}\right]}\) \(\newcommand{\cthreevec}[3]{\left[\begin{array}{c}#1 \\ #2 \\ #3 \end{array}\right]}\) \(\newcommand{\fourvec}[4]{\left[\begin{array}{r}#1 \\ #2 \\ #3 \\ #4 \end{array}\right]}\) \(\newcommand{\cfourvec}[4]{\left[\begin{array}{c}#1 \\ #2 \\ #3 \\ #4 \end{array}\right]}\) \(\newcommand{\fivevec}[5]{\left[\begin{array}{r}#1 \\ #2 \\ #3 \\ #4 \\ #5 \\ \end{array}\right]}\) \(\newcommand{\cfivevec}[5]{\left[\begin{array}{c}#1 \\ #2 \\ #3 \\ #4 \\ #5 \\ \end{array}\right]}\) \(\newcommand{\mattwo}[4]{\left[\begin{array}{rr}#1 \amp #2 \\ #3 \amp #4 \\ \end{array}\right]}\) \(\newcommand{\laspan}[1]{\text{Span}\{#1\}}\) \(\newcommand{\bcal}{\cal B}\) \(\newcommand{\ccal}{\cal C}\) \(\newcommand{\scal}{\cal S}\) \(\newcommand{\wcal}{\cal W}\) \(\newcommand{\ecal}{\cal E}\) \(\newcommand{\coords}[2]{\left\{#1\right\}_{#2}}\) \(\newcommand{\gray}[1]{\color{gray}{#1}}\) \(\newcommand{\lgray}[1]{\color{lightgray}{#1}}\) \(\newcommand{\rank}{\operatorname{rank}}\) \(\newcommand{\row}{\text{Row}}\) \(\newcommand{\col}{\text{Col}}\) \(\renewcommand{\row}{\text{Row}}\) \(\newcommand{\nul}{\text{Nul}}\) \(\newcommand{\var}{\text{Var}}\) \(\newcommand{\corr}{\text{corr}}\) \(\newcommand{\len}[1]{\left|#1\right|}\) \(\newcommand{\bbar}{\overline{\bvec}}\) \(\newcommand{\bhat}{\widehat{\bvec}}\) \(\newcommand{\bperp}{\bvec^\perp}\) \(\newcommand{\xhat}{\widehat{\xvec}}\) \(\newcommand{\vhat}{\widehat{\vvec}}\) \(\newcommand{\uhat}{\widehat{\uvec}}\) \(\newcommand{\what}{\widehat{\wvec}}\) \(\newcommand{\Sighat}{\widehat{\Sigma}}\) \(\newcommand{\lt}{<}\) \(\newcommand{\gt}{>}\) \(\newcommand{\amp}{&}\) \(\definecolor{fillinmathshade}{gray}{0.9}\)- Describe the structures of viruses, including their sizes.
- Identify examples of different virus morphologies (e.g. helical, icosohedral, etc.)
- Explain how tobacco mosaic virus infects cells, spreads, and replicates.
- Tell that specific viruses will only infect specific species.
- Describe how TMV has significant impacts on agriculture.
- Extract TMV from tobacco.
- Conduct a bioassay using TMV, analyze results of the experiment by t-test, and state conclusions of the experiment.
Introduction to Viruses
Viruses are noncellular parasitic entities that cannot be classified within any living kingdom. They can infect organisms as diverse as bacteria, plants, and animals. In fact, viruses exist in a sort of netherworld between a living organism and a nonliving entity. Living things grow, metabolize, and reproduce. In contrast, viruses are not cellular, do not have a metabolism or grow, and cannot divide by cell division. Viruses can copy, or replicate themselves; however, they are entirely dependent on resources derived from their host cells to produce progeny viruses—which are assembled in their mature form. No one knows exactly when or how viruses evolved or from what ancestral source because viruses have not left a fossil record. Some virologists contend that modern viruses are a mosaic of bits and pieces of nucleic acids picked up from various sources along their respective evolutionary paths.
Viruses are diverse entities: They vary in structure, methods of replication, and the hosts they infect. Nearly all forms of life—from prokaryotic bacteria and archaeans, to eukaryotes such as plants, animals, and fungi—have viruses that infect them. While most biological diversity can be understood through evolutionary history (such as how species have adapted to changing environmental conditions and how different species are related to one another through common descent), much about virus origins and evolution remains unknown.
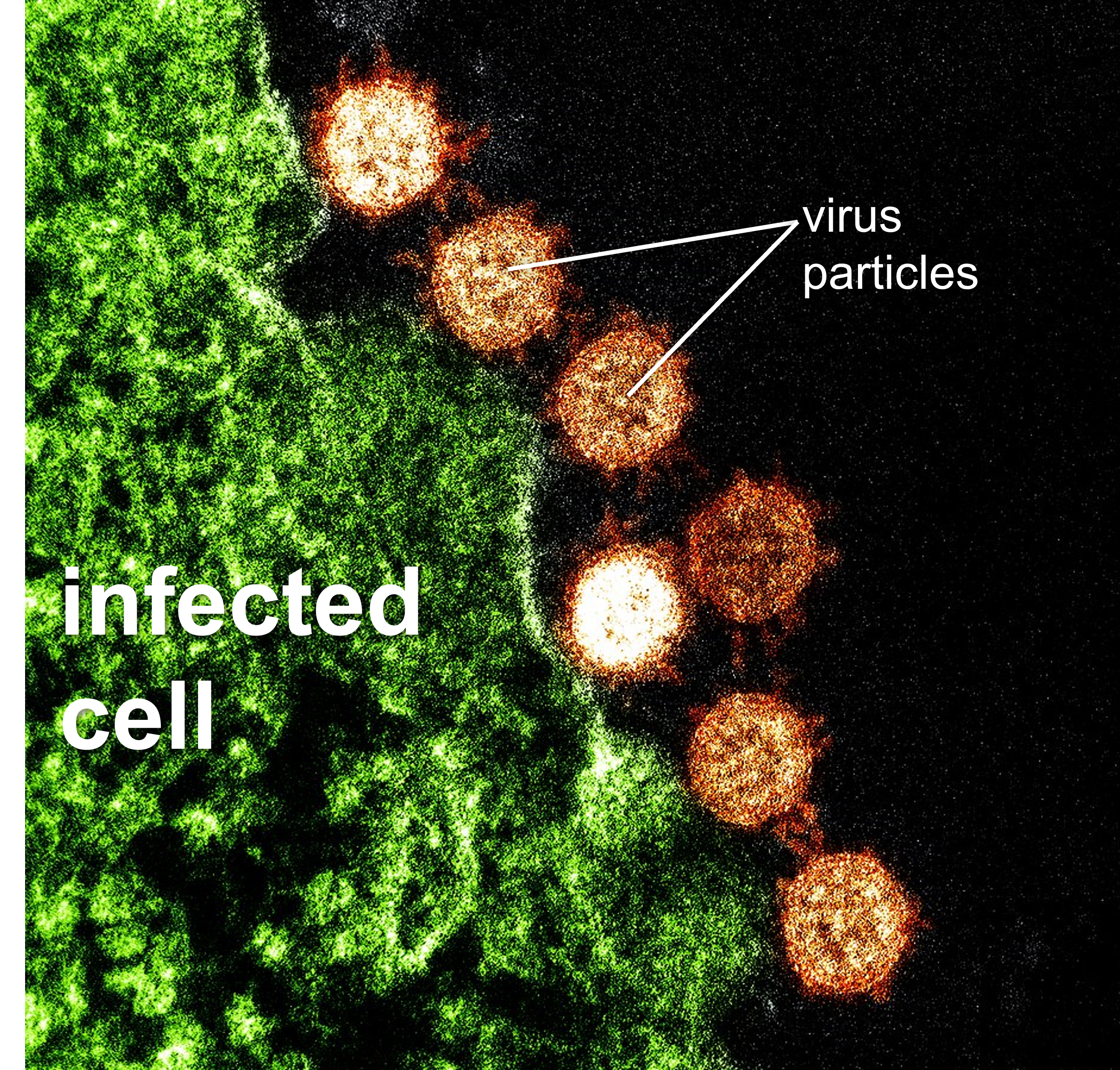
Figure 1: Electron micrograph of the SARS virus (red) attached to an infected cell (green). Note how very small virus particles are and they are not cellular.
Viruses were first discovered after the development of a porcelain filter—the Chamberland-Pasteur filter—that could remove all bacteria visible in the microscope from any liquid sample. In 1886, Adolph Meyer demonstrated that a disease of tobacco plants—tobacco mosaic disease—could be transferred from a diseased plant to a healthy one via liquid plant extracts. In 1892, Dmitri Ivanowski showed that this disease could be transmitted in this way even after the Chamberland-Pasteur filter had removed all viable bacteria from the extract. Still, it was many years before it was proved that these “filterable” infectious agents were not simply very small bacteria but were a new type of very small, disease-causing particle.
Virus Structures
Most virions, or single virus particles, are very small, about 20 to 250 nanometers in diameter. However, some recently discovered viruses from amoebae range up to 1000 nm in diameter. With the exception of large virions, like the poxvirus and other large DNA viruses, viruses cannot be seen with a light microscope. It was not until the development of the electron microscope in the late 1930s that scientists got their first good view of the structure of the tobacco mosaic virus, discussed above, and other viruses. The surface structure of virions can be observed by both scanning and transmission electron microscopy, whereas the internal structures of the virus can only be observed in images from a transmission electron microscope. The use of electron microscopy and other technologies has allowed for the discovery of many viruses of all types of living organisms.
*viruses in this interactive tool include: measles virus, hiv, phage, influenza virus, hepatitis virus, and rhinovirus
V
iruses are noncellular, meaning they are biological entities that do not have a cellular structure. They therefore lack most of the components of cells, such as organelles, ribosomes, and the plasma membrane. A virion consists of a nucleic acid core (DNA or RNA), an outer protein coating or capsid, and sometimes an outer envelope made of protein and phospholipid membranes derived from the host cell. Viruses may also contain additional proteins, such as enzymes, within the capsid or attached to the viral genome. The most obvious difference between members of different viral families is the variation in their morphology, which is quite diverse. An interesting feature of viral complexity is that the complexity of the host does not necessarily correlate with the complexity of the virion. In fact, some of the most complex virion structures are found in the bacteriophages—viruses that infect the simplest living organisms, bacteria.

Figure 2: Viruses have diverse shapes. (a) Helical viruses have protein capsids form a hollow tube containing the viral genetic molecule(s). (b) Icosahedral viruses is a three-dimensional hollow structure formed by 20 faces (icosahedron) with the viral genetic material inside. (c) Enveloped viruses are surrounding by a lipid membrane that arose from the plasma membrane of a host cell. (d) Complex or head-and-tail viruses have a head-like structure formed by the capsid with genetic material inside, and a sheath or tail-like structure. (credit a “micrograph”: modification of work by USDA ARS; credit b “micrograph”: modification of work by U.S. Department of Energy) (credit d: modification of work by U.S. Department of Energy, Office of Science, LBL, PBD)
Viruses come in many shapes and sizes, but these features are consistent for each viral family. As we have seen, all virions have a nucleic acid genome covered by a protective capsid. The proteins of the capsid are encoded in the viral genome, and are called capsomeres. Some viral capsids are simple helices or polyhedral “spheres,” whereas others are quite complex in structure.
In general, viruses structures can be classified as: helical, icosahedral, enveloped, and complex, also known as head-and-tail. Helical capsids are long and cylindrical. Many plant viruses are helical, including TMV. Icosahedral viruses have shapes that are roughly spherical, such as those of poliovirus or herpesviruses. Enveloped viruses have membranes derived from the host cell that surrounds the capsids. Animal viruses, such as HIV, are frequently enveloped. Head-and-tail viruses infect bacteria and have a head that is similar to icosahedral viruses and a tail shaped like helical viruses.
Viral Reproduction
All viruses depend on cells for reproduction and metabolic processes. By themselves, viruses do not encode for all of the enzymes necessary for viral replication. But within a host cell, a virus can commandeer cellular machinery to produce more viral particles. Bacteriophages replicate only in the cytoplasm, since prokaryotic cells do not have a nucleus or organelles. In eukaryotic cells, most DNA viruses can replicate inside the nucleus, with an exception observed in the large DNA viruses, such as the poxviruses, that can replicate in the cytoplasm. With a few exceptions, RNA viruses that infect animal cells replicate in the cytoplasm. An important exception that will be highlighted later is Influenza virus.
The life cycle of bacteriophages has been a good model for understanding how viruses affect the cells they infect, since similar processes have been observed for eukaryotic viruses, which can cause immediate death of the cell or establish a latent or chronic infection.
The Lytic Cycle
During the lytic cycle of virulent phage, the bacteriophage takes over the cell, reproduces new phages, and destroys the cell. T-even phage is a good example of a well-characterized class of virulent phages. There are five stages in the bacteriophage lytic cycle. Attachment is the first stage in the infection process in which the phage interacts with specific bacterial surface receptors (e.g., lipopolysaccharides and OmpC protein on host surfaces). Most phages have a narrow host range and may infect one species of bacteria or one strain within a species. This unique recognition can be exploited for targeted treatment of bacterial infection by phage therapy or for phage typing to identify unique bacterial subspecies or strains. The second stage of infection is entry or penetration. This occurs through contraction of the tail sheath, which acts like a hypodermic needle to inject the viral genome through the cell wall and membrane. The phage head and remaining components remain outside the bacteria.

Figure 3: A virulent phage shows only the lytic cycle pictured here. In the lytic cycle, the phage replicates and lyses the host cell. 1. Attachment: the phage attaches to the surface of the host. 2. Penetration: the viral DNA enters the host cell. 3. Biosynthesis: phage DNA replicates and phage proteins are made. 4. Maturation: New phage particles are assembled. 5. Lysis: The cell lyses, releasing the newly made phages.
The third stage of infection is biosynthesis of new viral components. After entering the host cell, the virus synthesizes virus-encoded endonucleases to degrade the bacterial chromosome. It then hijacks the host cell to replicate, transcribe, and translate the necessary viral components (capsomeres, sheath, base plates, tail fibers, and viral enzymes) for the assembly of new viruses. Polymerase genes are usually expressed early in the cycle, while capsid and tail proteins are expressed later. During the maturation phase, new virions are created. To liberate free phages, the bacterial cell wall is disrupted by phage proteins such as holin or lysozyme. The final stage is release. Mature viruses burst out of the host cell in a process called lysis and the progeny viruses are liberated into the environment to infect new cells.
The Lysogenic Cycle
In a lysogenic cycle, the phage genome also enters the cell through attachment and penetration. A prime example of a phage with this type of life cycle is the lambda phage. During the lysogenic cycle, instead of killing the host, the phage genome integrates into the bacterial chromosome and becomes part of the host. The integrated phage genome is called a prophage. A bacterial host with a prophage is called a lysogen. The process in which a bacterium is infected by a temperate phage is called lysogeny. It is typical of temperate phages to be latent or inactive within the cell. As the bacterium replicates its chromosome, it also replicates the phage’s DNA and passes it on to new daughter cells during reproduction. The presence of the phage may alter the phenotype of the bacterium, since it can bring in extra genes (e.g., toxin genes that can increase bacterial virulence). This change in the host phenotype is called lysogenic conversion or phage conversion. Some bacteria, such as Vibrio cholerae and Clostridium botulinum, are less virulent in the absence of the prophage. The phages infecting these bacteria carry the toxin genes in their genome and enhance the virulence of the host when the toxin genes are expressed. In the case of V. cholera, phage encoded toxin can cause severe diarrhea; in C. botulinum, the toxin can cause paralysis. During lysogeny, the prophage will persist in the host chromosome until induction, which results in the excision of the viral genome from the host chromosome. After induction has occurred the temperate phage can proceed through a lytic cycle and then undergo lysogeny in a newly infected cell.
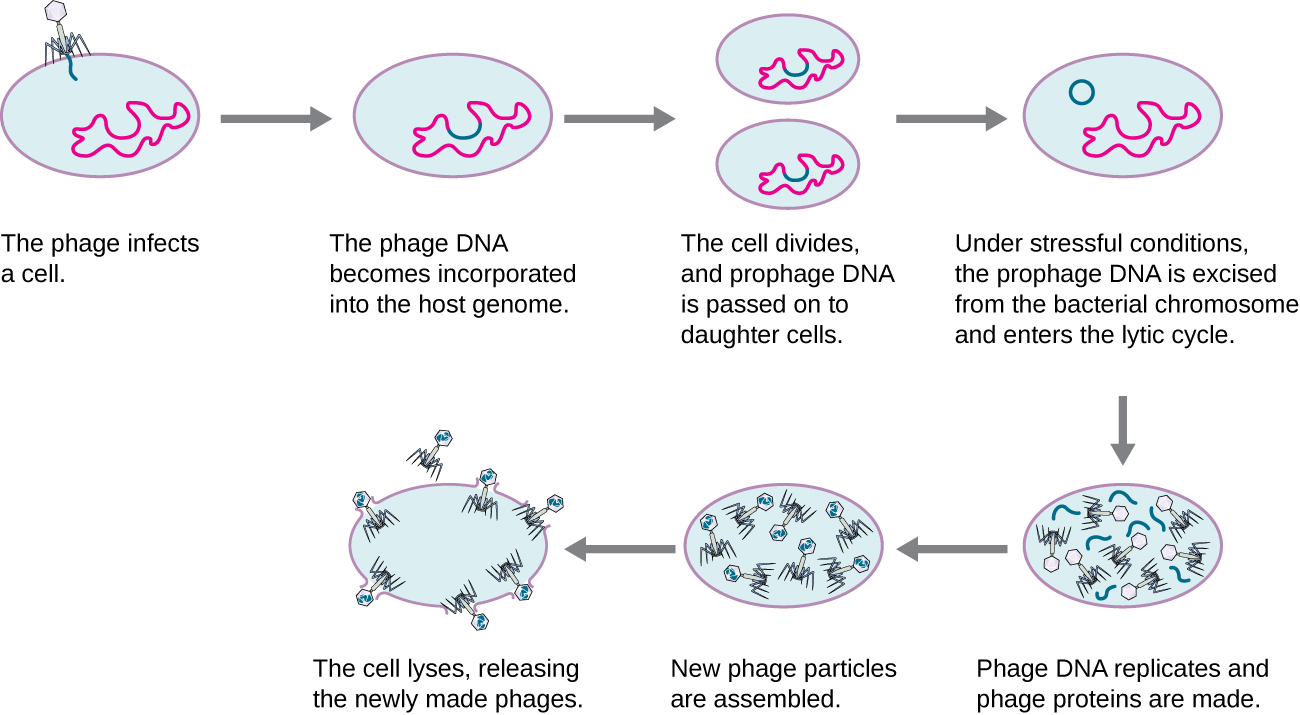
Figure 4: A temperate bacteriophage has both lytic and lysogenic cycles. In the lysogenic cycle, phage DNA is incorporated into the host genome, forming a prophage, which is passed on to subsequent generations of cells. Environmental stressors such as starvation or exposure to toxic chemicals may cause the prophage to be excised and enter the lytic cycle.
Introduction to Plant Viruses
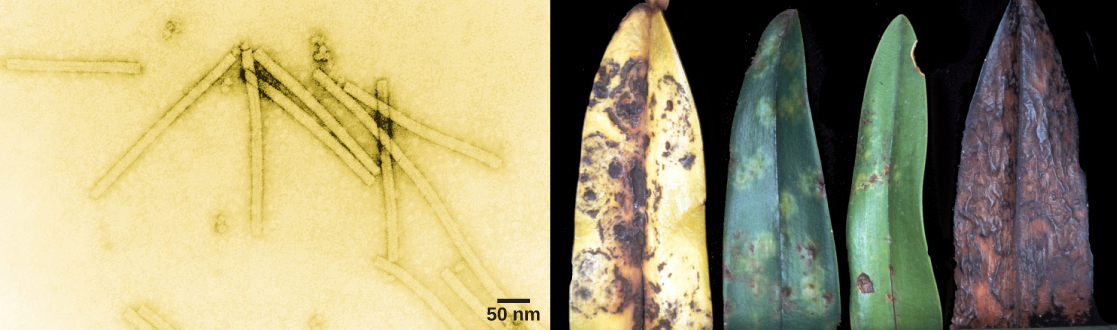
Figure 5: The tobacco mosaic virus (TMV), seen here by transmission electron microscopy (left), was the first virus to be discovered. The virus causes disease in tobacco and other plants, such as the orchid (right). (credit a: USDA ARS; credit b: modification of work by USDA Forest Service, Department of Plant Pathology Archive North Carolina State University; scale-bar data from Matt Russell)
Most plant viruses, like the tobacco mosaic virus, have single-stranded (+) RNA genomes. However, there are also plant viruses in most other virus categories. Unlike bacteriophages, plant viruses do not have active mechanisms for delivering the viral genome across the protective cell wall. For a plant virus to enter a new host plant, some type of mechanical damage must occur. This damage is often caused by weather, insects, animals, fire, or human activities like farming or landscaping. Movement from cell to cell within a plant can be facilitated by viral modification of plasmodesmata (cytoplasmic threads that pass from one plant cell to the next). Additionally, plant offspring may inherit viral diseases from parent plants. Plant viruses can be transmitted by a variety of vectors, through contact with an infected plant’s sap, by living organisms such as insects and nematodes, and through pollen. The transfer of a virus from one plant to another is known as horizontal transmission, whereas the inheritance of a virus from a parent is called vertical transmission.
Symptoms of viral diseases vary according to the virus and its host. One common symptom is hyperplasia, the abnormal proliferation of cells that causes the appearance of plant tumors known as galls. Other viruses induce hypoplasia, or decreased cell growth, in the leaves of plants, causing thin, yellow areas to appear. Still other viruses affect the plant by directly killing plant cells, a process known as cell necrosis. Other symptoms of plant viruses include malformed leaves; black streaks on the stems of the plants; altered growth of stems, leaves, or fruits; and ring spots, which are circular or linear areas of discoloration found in a leaf.
Table 1: Some Common Symptoms of Plant Viral Diseases
Symptom | Appears as |
---|---|
Hyperplasia | Galls (tumors) |
Hypoplasia | Thinned, yellow splotches on leaves |
Cell necrosis | Dead, blackened stems, leaves, or fruit |
Abnormal growth patterns | Malformed stems, leaves, or fruit |
Discoloration | Yellow, red, or black lines, or rings in stems, leaves, or fruit |
Plant viruses can seriously disrupt crop growth and development, significantly affecting our food supply. They are responsible for poor crop quality and quantity globally, and can bring about huge economic losses annually. Others viruses may damage plants used in landscaping. Some viruses that infect agricultural food plants include the name of the plant they infect, such as tomato spotted wilt virus, bean common mosaic virus, and cucumber mosaic virus. In plants used for landscaping, two of the most common viruses are peony ring spot and rose mosaic virus. There are far too many plant viruses to discuss each in detail, but symptoms of bean common mosaic virus result in lowered bean production and stunted, unproductive plants. In the ornamental rose, the rose mosaic disease causes wavy yellow lines and colored splotches on the leaves of the plant.
Tobacco Mosaic Virus
The tobacco mosaic virus (TMV) is a plant pathogen that causes tobacco mosaic disease. TMV infects tobacco plants, tomato plants, bean plants, and other plant species within the plant family Solanaceae (sometimes called "nightshades") (Scholthof, 2000). Tobacco mosaic disease has been reported as early as the early 1800s in Colombia where tobacco was an important agricultural crop (Scholthof, 2008). This virus impacted the quality of the tobacco by causing it to taste incredibly bitter, and therefore could devastate the value of tobacco crops (Scholthof, 2008).
TMV is an RNA virus with a helical capsid. When TMV infects a tobacco plant, it can cause abnormal growth patterns (e.g. curling leaves), cell necrosis, and discoloration - see Figure 5 above (Scholthof, 2000). The impacts of TMV on tomato plant today are more profound (~20% crop loss) than on tobacco crops, since TMV-resistant tobacco crops are typically selected and grown agriculturally (~1% crop loss by TMV) (Scholthof, 2000).

Figure 6: Structure of tobacco mosaic virus (TMV).
As mentioned above in the Introduction to Plant Viruses section, plant cells have a thick cell wall that viruses cannot penetrate, and TMV is no different. A plant cell must be injured in order for TMV to cause infection (Scholthof, 2000). Transfer of the TMV particles involves mechanical transmission from a farm tool carrying the virus, a farm worker's hand that is carrying the virus from another plant or from tobacco they have used, or from an infected leaf to non-infected leaf when they make contact with each other (Scholthof, 2000). After TMV enters a plant cell, the protein capsid disassembles to release the RNA genome of the virus (Scholthof, 2000). Within minutes of a plant cell being infected with TMV, the released viral RNA starts to be used by the host cell's ribosomes to begin making viral proteins (Scholthof, 2000). In summary, the TMV viral proteins replicate the viral RNA genome (to make more virus particles), form subunits for the capsid (to make more virus particles), and facilitate transfer of the virus between adjacent plant cells through cell-to-cell plant communication junctions called plasmodesmata (Scholthof, 2000). Newly synthesized virus particles can not only travel through plasmodesmata, they can also enter the plant's phloem (passages that connect the entire plant), and therefore infect the entire plant (Scholthof, 2000). If an infected cell has its cell wall damaged, virus particles can be transmitted from that cell to infect other plants (Scholthof, 2000).
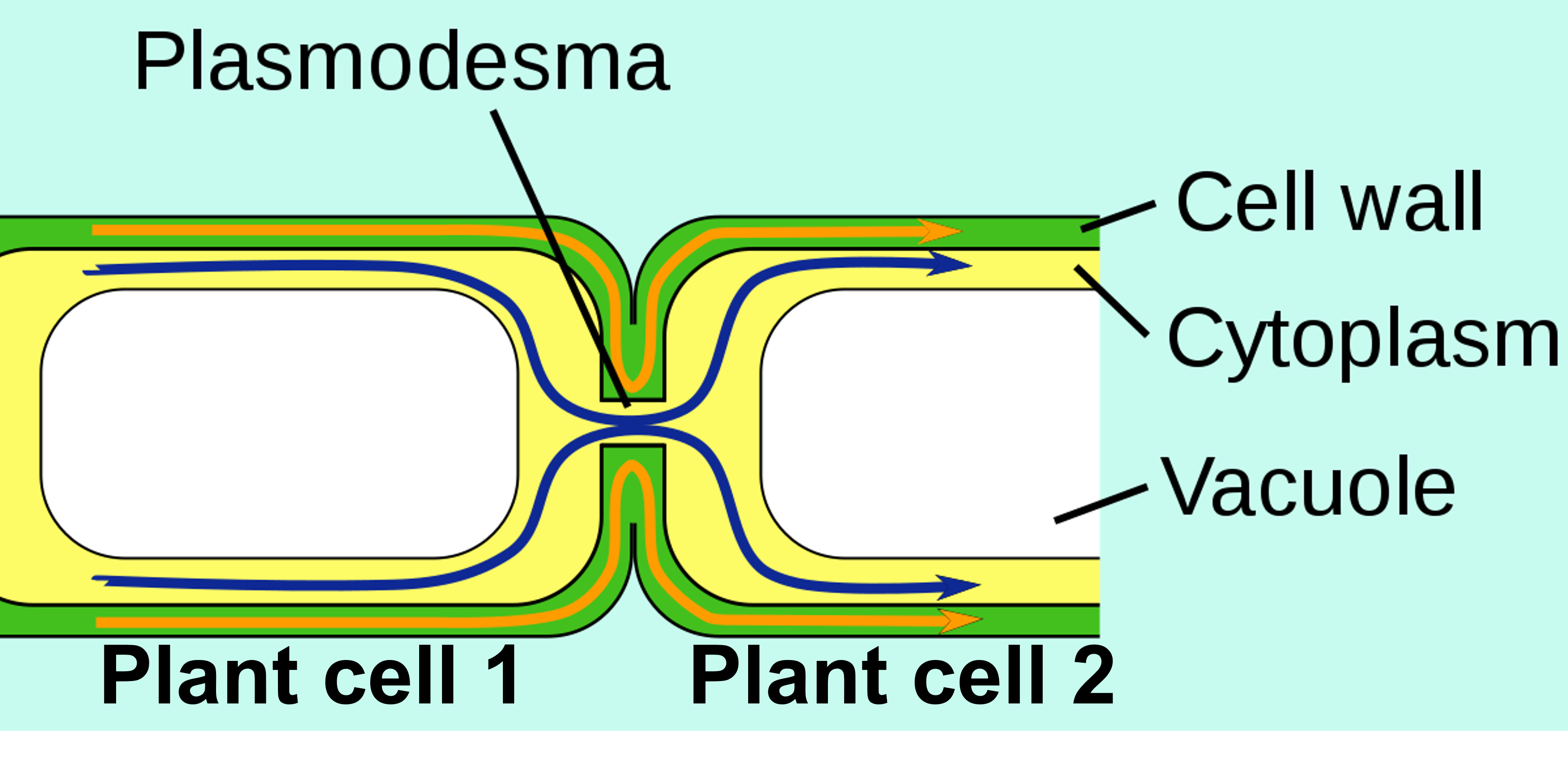
Figure 7: Two adjacent plant cells are shown and their connection with plasmodesmata. A "movement protein" coded in the TMV RNA genome facilitates movement of the virus particles and infection of adjacent cells using these plasmodesmata (Scholthof, 2000).
Laboratory Instructions
Grow Plants to Infect
- Prepare a plant pot with soil.
- Plant 5-6 tomato or bean seeds as instructed on the seed package.
- Water and provide light for the plants for 4-6 weeks as instructed on the seed package.
What is a "Bioassay?"
An assay is a type of scientific test used to determine the composition of a substance. In this case, we will assay tobacco to determine:
- Does this tobacco contain TMV?
- How much infective TMV is in the tobacco?
- Does milk have an impact on the ability of TMV to infect plant cells?
"Bio-" relates to life. In this case, "bio-" refers to the use of a plant used in this assay. Viruses cannot be grown on their own - they require cells. In this case, a living organism (a plant) is used to enable us to experiment with this virus.
Bioassay Instructions
Wash your hands with soap anytime they may have touched anything that might contain tobacco mosaic virus. You may need to wash your hands multiple times throughout this laboratory protocol and be sure to wash your hands at the end of the laboratory so you do not carry and spread the virus into the environment.
This will prevent spread of the virus and prevent cross-contamination in the experiment.
Thoroughly clean any laboratory materials (test tubes, stir rods, etc.) that could have come into contact with the tobacco mosaic virus with disinfectant.
This will prevent spread of the virus and prevent cross-contamination in the experiment.
Create a Virus Extract from Tobacco
- Mix tobacco from 2-3 different sources (different brands of tobacco).
- Use a mortar and pestle to produce finely ground tobacco. This will damage the cell walls of the tobacco to release the tobacco mosaic virus from infected cells.
- Weigh 1 g of tobacco.
- Measure 15 mL of diatomaceous earth dissolved in phosphate buffer and pour into a small beaker. The diatomaceous earth will be important for creating friction and causing damage to plant leaves to enable infection.
- Add the 1 g of tobacco to the 15 mL of phosphate buffer with diatomaceous earth.
- Use a stir rod to further mash the tobacco and stir it into the liquid. This will release tobacco mosaic virus into the phosphate buffer.
- Put a funnel into a test tube.
- Line the inside of the funnel with 3-4 layers of cheesecloth.
- Pour the tobacco-phosphate buffer-diatomaceous earth mixture through the cheesecloth. This will remove tobacco particles, but allow the diatomaceous earth and buffer carrying the tobacco mosaic virus into the test tube.
- The liquid in this test tube will hereafter be referred to as the "virus extract."
- Wash your hands thoroughly.
The "virus extract" contains tobacco mosaic virus. Any solution that this virus extract is added to should also contain the virus. Any solution that does not contain this virus extract should not contain the virus.
Prepare Three Test Samples & Apply to Plant Leaves
Prepare Plant for Sample Application
- Choose three different branches of the plant that have healthy-looking leaves.
- Use masking tape to create a "flag" on each branch, each labeled as:
- Control (No Virus)
- Virus in Water
- Virus in Milk
Control (No Virus)
- Label a clean test tube as "Control (No Virus)."
- Measure 1.5 mL of the phosphate buffer containing diatomaceous earth and pour into the test tube.
- Measure 1.5 mL of deionized water and pour into the test tube.
- Gently mix the test tube by lightly swirling of lightly flicking the bottom of the test tube with your finger.
- Dip a sterile cotton swab into the Control (No Virus) solution.
- Gently swab the top surface of one leaf on the plant branch you labeled "Control (No Virus)"
- Repeat steps 5-6 until all leaves on the "Control (No Virus)" branch have been swabbed. If any of the leaves on this branch already have discoloration, pull the leaf off the plant instead of swabbing it.
Virus in Water
- Label a clean test tube as "Virus in Water."
- Measure 1.5 mL of the virus extract solution and pour into the test tube.
- Measure 1.5 mL of deionized water and pour into the test tube.
- Gently mix the test tube by lightly swirling of lightly flicking the bottom of the test tube with your finger.
- Dip a sterile cotton swab into the Virus in Water solution.
- Gently swab the top surface of one leaf on the plant branch you labeled "Virus in Water" being careful not to touch any other leaves on the plant.
- Repeat steps 5-6 until all leaves on the "Virus in Water" branch have been swabbed. If any of the leaves on this branch already have discoloration, pull the leaf off the plant instead of swabbing it.
- Wash your hands well after this step to prevent spreading the virus to tools, bench tops, etc.
Virus with Milk
- Label a clean test tube as "Virus with Milk."
- Measure 1.5 mL of the virus extract solution and pour into the test tube.
- Measure 1.5 mL of milk and pour into the test tube.
- Gently mix the test tube by lightly swirling of lightly flicking the bottom of the test tube with your finger.
- Dip a sterile cotton swab into the Virus with Milk solution.
- Gently swab the top surface of one leaf on the plant branch you labeled "Virus with Milk" being careful not to touch any other leaves on the plant.
- Repeat steps 5-6 until all leaves on the "Virus with Milk" branch have been swabbed. If any of the leaves on this branch already have discoloration, pull the leaf off the plant instead of swabbing it.
- Wash your hands well after this step to prevent spreading the virus to tools, bench tops, etc.
Post-Inoculation Plant Care
- Continue caring for plants as you did before Inoculation (light and water as indicated on the seed package). Take care that the leaves of the plants do not touch each other.
- Wait 2-3 weeks before examining plant leaves and collecting data.
Results & Questions
# of Lesions on Leaf 1 | # of Lesions on Leaf 2 | # of Lesions on Leaf 3 | # of Lesions on Leaf 4 | # of Lesions on Leaf 5 | # of Lesions on Leaf 6 | Average # of Lesions | |
---|---|---|---|---|---|---|---|
Control (No Virus) | |||||||
Virus in Water | |||||||
Virus with Milk |
Class Average # of Lesions | t-test Range | |
---|---|---|
Control (No Virus) | ||
Virus in Water | ||
Virus with Milk |
- Fill in the table above with data from the tobacco mosaic bioassay. Depending on the number of leaves on the plant, you may or may not need every box in the table above.
- Share the average number of lesions per leaf for Control (No Virus), Virus in Water, and Virus with Milk with your instructor. They will calculate the class average for each and t-test ranges. Write these data in the table above.
- Examine the class average of lesions and the t-test ranges and interpret the t-test statistic. Write conclusions about whether or not these data are statistically significantly different from each other (see the t-test Statistical Analysis section in the Control of Microbial Growth lab for guidance):
- Control vs. Virus in Water:
- Control vs. Virus with Milk:
- Virus in Water vs. Virus with Milk:
- Explain how TMV infects plant cells.
- Why was diatomaceous earth used in this experiment (think about how TMV infects plant cells)?
- How can TMV spread in a plant?
- What plant species does TMV infect?
- Can TMV infect humans? Explain your answer by discussing the specificity of viruses.
- Define bioassay.
Attributions
- Apoplast and symplast pathways.svg by Jackacon, vectorised by Smartse is in the public domain
- Biology 2e by OpenStax is licensed under CC BY 4.0
- Microbiology by OpenStax is licensed under CC BY 4.0
- SARS Virus Particles (43093982224).jpg by NIAID is in the public domain
- TMV Structure.png by Graham Colm is licsensed under CC BY-SA 3.0
Works Cited
- Scholthof, K-B.G. 2000. Tobacco mosaic virus. The Plant Health Instructor. DOI: 10.1094/PHI-I-2000-1010-01
Updated 2005. - Scholthof, K-B. G. 2008. Tobacco Mosaic Virus: The Beginning of Plant Pathology. Online. APSnet Features. doi: 10.1094/APSnetFeatures-2008-0408