1.39: Control of Microbial Growth
- Page ID
- 79458
\( \newcommand{\vecs}[1]{\overset { \scriptstyle \rightharpoonup} {\mathbf{#1}} } \)
\( \newcommand{\vecd}[1]{\overset{-\!-\!\rightharpoonup}{\vphantom{a}\smash {#1}}} \)
\( \newcommand{\id}{\mathrm{id}}\) \( \newcommand{\Span}{\mathrm{span}}\)
( \newcommand{\kernel}{\mathrm{null}\,}\) \( \newcommand{\range}{\mathrm{range}\,}\)
\( \newcommand{\RealPart}{\mathrm{Re}}\) \( \newcommand{\ImaginaryPart}{\mathrm{Im}}\)
\( \newcommand{\Argument}{\mathrm{Arg}}\) \( \newcommand{\norm}[1]{\| #1 \|}\)
\( \newcommand{\inner}[2]{\langle #1, #2 \rangle}\)
\( \newcommand{\Span}{\mathrm{span}}\)
\( \newcommand{\id}{\mathrm{id}}\)
\( \newcommand{\Span}{\mathrm{span}}\)
\( \newcommand{\kernel}{\mathrm{null}\,}\)
\( \newcommand{\range}{\mathrm{range}\,}\)
\( \newcommand{\RealPart}{\mathrm{Re}}\)
\( \newcommand{\ImaginaryPart}{\mathrm{Im}}\)
\( \newcommand{\Argument}{\mathrm{Arg}}\)
\( \newcommand{\norm}[1]{\| #1 \|}\)
\( \newcommand{\inner}[2]{\langle #1, #2 \rangle}\)
\( \newcommand{\Span}{\mathrm{span}}\) \( \newcommand{\AA}{\unicode[.8,0]{x212B}}\)
\( \newcommand{\vectorA}[1]{\vec{#1}} % arrow\)
\( \newcommand{\vectorAt}[1]{\vec{\text{#1}}} % arrow\)
\( \newcommand{\vectorB}[1]{\overset { \scriptstyle \rightharpoonup} {\mathbf{#1}} } \)
\( \newcommand{\vectorC}[1]{\textbf{#1}} \)
\( \newcommand{\vectorD}[1]{\overrightarrow{#1}} \)
\( \newcommand{\vectorDt}[1]{\overrightarrow{\text{#1}}} \)
\( \newcommand{\vectE}[1]{\overset{-\!-\!\rightharpoonup}{\vphantom{a}\smash{\mathbf {#1}}}} \)
\( \newcommand{\vecs}[1]{\overset { \scriptstyle \rightharpoonup} {\mathbf{#1}} } \)
\( \newcommand{\vecd}[1]{\overset{-\!-\!\rightharpoonup}{\vphantom{a}\smash {#1}}} \)
\(\newcommand{\avec}{\mathbf a}\) \(\newcommand{\bvec}{\mathbf b}\) \(\newcommand{\cvec}{\mathbf c}\) \(\newcommand{\dvec}{\mathbf d}\) \(\newcommand{\dtil}{\widetilde{\mathbf d}}\) \(\newcommand{\evec}{\mathbf e}\) \(\newcommand{\fvec}{\mathbf f}\) \(\newcommand{\nvec}{\mathbf n}\) \(\newcommand{\pvec}{\mathbf p}\) \(\newcommand{\qvec}{\mathbf q}\) \(\newcommand{\svec}{\mathbf s}\) \(\newcommand{\tvec}{\mathbf t}\) \(\newcommand{\uvec}{\mathbf u}\) \(\newcommand{\vvec}{\mathbf v}\) \(\newcommand{\wvec}{\mathbf w}\) \(\newcommand{\xvec}{\mathbf x}\) \(\newcommand{\yvec}{\mathbf y}\) \(\newcommand{\zvec}{\mathbf z}\) \(\newcommand{\rvec}{\mathbf r}\) \(\newcommand{\mvec}{\mathbf m}\) \(\newcommand{\zerovec}{\mathbf 0}\) \(\newcommand{\onevec}{\mathbf 1}\) \(\newcommand{\real}{\mathbb R}\) \(\newcommand{\twovec}[2]{\left[\begin{array}{r}#1 \\ #2 \end{array}\right]}\) \(\newcommand{\ctwovec}[2]{\left[\begin{array}{c}#1 \\ #2 \end{array}\right]}\) \(\newcommand{\threevec}[3]{\left[\begin{array}{r}#1 \\ #2 \\ #3 \end{array}\right]}\) \(\newcommand{\cthreevec}[3]{\left[\begin{array}{c}#1 \\ #2 \\ #3 \end{array}\right]}\) \(\newcommand{\fourvec}[4]{\left[\begin{array}{r}#1 \\ #2 \\ #3 \\ #4 \end{array}\right]}\) \(\newcommand{\cfourvec}[4]{\left[\begin{array}{c}#1 \\ #2 \\ #3 \\ #4 \end{array}\right]}\) \(\newcommand{\fivevec}[5]{\left[\begin{array}{r}#1 \\ #2 \\ #3 \\ #4 \\ #5 \\ \end{array}\right]}\) \(\newcommand{\cfivevec}[5]{\left[\begin{array}{c}#1 \\ #2 \\ #3 \\ #4 \\ #5 \\ \end{array}\right]}\) \(\newcommand{\mattwo}[4]{\left[\begin{array}{rr}#1 \amp #2 \\ #3 \amp #4 \\ \end{array}\right]}\) \(\newcommand{\laspan}[1]{\text{Span}\{#1\}}\) \(\newcommand{\bcal}{\cal B}\) \(\newcommand{\ccal}{\cal C}\) \(\newcommand{\scal}{\cal S}\) \(\newcommand{\wcal}{\cal W}\) \(\newcommand{\ecal}{\cal E}\) \(\newcommand{\coords}[2]{\left\{#1\right\}_{#2}}\) \(\newcommand{\gray}[1]{\color{gray}{#1}}\) \(\newcommand{\lgray}[1]{\color{lightgray}{#1}}\) \(\newcommand{\rank}{\operatorname{rank}}\) \(\newcommand{\row}{\text{Row}}\) \(\newcommand{\col}{\text{Col}}\) \(\renewcommand{\row}{\text{Row}}\) \(\newcommand{\nul}{\text{Nul}}\) \(\newcommand{\var}{\text{Var}}\) \(\newcommand{\corr}{\text{corr}}\) \(\newcommand{\len}[1]{\left|#1\right|}\) \(\newcommand{\bbar}{\overline{\bvec}}\) \(\newcommand{\bhat}{\widehat{\bvec}}\) \(\newcommand{\bperp}{\bvec^\perp}\) \(\newcommand{\xhat}{\widehat{\xvec}}\) \(\newcommand{\vhat}{\widehat{\vvec}}\) \(\newcommand{\uhat}{\widehat{\uvec}}\) \(\newcommand{\what}{\widehat{\wvec}}\) \(\newcommand{\Sighat}{\widehat{\Sigma}}\) \(\newcommand{\lt}{<}\) \(\newcommand{\gt}{>}\) \(\newcommand{\amp}{&}\) \(\definecolor{fillinmathshade}{gray}{0.9}\)- Define the following terms: fomites, sterilization, asepsis, sepsis, commercial sterilization, disinfection, sanitization, antisepsis, degerming
- Give at least five examples of the categories of physical controls for microbes and what they involve.
- Give at least three examples of categories of chemical controls for microbes and what they involve.
- Successfully conduct an experiment comparing soap, disinfectant, and untreated surfaces (fomites) and the microbial load present.
- Graph and analyze results from control of microbial growth experiment.
- Interpret t-test results and state conclusions based on the interpretation.
Clean Enough?
How clean is clean? People wash their cars and vacuum the carpets, but most would not want to eat from these surfaces. Similarly, we might eat with silverware cleaned in a dishwasher, but we could not use the same dishwasher to clean surgical instruments. As these examples illustrate, “clean” is a relative term. Car washing, vacuuming, and dishwashing all reduce the microbial load on the items treated, thus making them “cleaner.” But whether they are “clean enough” depends on their intended use. Because people do not normally eat from cars or carpets, these items do not require the same level of cleanliness that silverware does. Likewise, because silverware is not used for invasive surgery, these utensils do not require the same level of cleanliness as surgical equipment, which requires sterilization to prevent infection.

Figure 1: Most environments, including cars, are not sterile. A study1 analyzed 11 locations within 18 different cars to determine the number of microbial colony-forming units (CFUs) present. The center console harbored by far the most microbes (506 CFUs), possibly because that is where drinks are placed (and often spilled). Frequently touched sites also had high concentrations. (credit “photo”: modification of work by Jeff Wilcox)
Why not play it safe and sterilize everything? Sterilizing everything we come in contact with is impractical, as well as potentially dangerous. As this chapter will demonstrate, sterilization protocols often require time- and labor-intensive treatments that may degrade the quality of the item being treated or have toxic effects on users. Therefore, the user must consider the item’s intended application when choosing a cleaning method to ensure that it is “clean enough.”
Fomites
To prevent the spread of human disease, it is necessary to control the growth and abundance of microbes in or on various items frequently used by humans. Inanimate items, such as doorknobs, toys, or towels, which may harbor microbes and aid in disease transmission, are called fomites. Two factors heavily influence the level of cleanliness required for a particular fomite and, hence, the protocol chosen to achieve this level. The first factor is the application for which the item will be used. For example, invasive applications that require insertion into the human body require a much higher level of cleanliness than applications that do not. The second factor is the level of resistance to antimicrobial treatment by potential pathogens. For example, foods preserved by canning often become contaminated with the bacterium Clostridium botulinum, which produces the neurotoxin that causes botulism. Because C. botulinum can produce endospores that can survive harsh conditions, extreme temperatures and pressures must be used to eliminate the endospores. Other organisms may not require such extreme measures and can be controlled by a procedure such as washing clothes in a laundry machine.
Control of Microbial Growth: Sterilization
The most extreme protocols for microbial control aim to achieve sterilization: the complete removal or killing of all vegetative cells, endospores, and viruses from the targeted item or environment. Sterilization protocols are generally reserved for laboratory, medical, manufacturing, and food industry settings, where it may be imperative for certain items to be completely free of potentially infectious agents. Sterilization can be accomplished through either physical means, such as exposure to high heat, pressure, or filtration through an appropriate filter, or by chemical means. Chemicals that can be used to achieve sterilization are called sterilants. Sterilants effectively kill all microbes and viruses, and, with appropriate exposure time, can also kill endospores.
For many clinical purposes, aseptic technique is necessary to prevent contamination of sterile surfaces. Aseptic technique involves a combination of protocols that collectively maintain sterility, or asepsis, thus preventing contamination of the patient with microbes and infectious agents. Failure to practice aseptic technique during many types of clinical procedures may introduce microbes to the patient’s body and put the patient at risk for sepsis, a systemic inflammatory response to an infection that results in high fever, increased heart and respiratory rates, shock, and, possibly, death. Medical procedures that carry risk of contamination must be performed in a sterile field, a designated area that is kept free of all vegetative microbes, endospores, and viruses. Sterile fields are created according to protocols requiring the use of sterilized materials, such as packaging and drapings, and strict procedures for washing and application of sterilants. Other protocols are followed to maintain the sterile field while the medical procedure is being performed.
One food sterilization protocol, commercial sterilization, uses heat at a temperature low enough to preserve food quality but high enough to destroy common pathogens responsible for food poisoning, such as C. botulinum. Because C. botulinum and its endospores are commonly found in soil, they may easily contaminate crops during harvesting, and these endospores can later germinate within the anaerobic environment once foods are canned. Metal cans of food contaminated with C. botulinum will bulge due to the microbe’s production of gases; contaminated jars of food typically bulge at the metal lid. To eliminate the risk for C. botulinum contamination, commercial food-canning protocols are designed with a large margin of error. They assume an impossibly large population of endospores (1012 per can) and aim to reduce this population to 1 endospore per can to ensure the safety of canned foods. For example, low- and medium-acid foods are heated to 121 °C for a minimum of 2.52 minutes, which is the time it would take to reduce a population of 1012 endospores per can down to 1 endospore at this temperature. Even so, commercial sterilization does not eliminate the presence of all microbes; rather, it targets those pathogens that cause spoilage and foodborne diseases, while allowing many nonpathogenic organisms to survive. Therefore, “sterilization” is somewhat of a misnomer in this context, and commercial sterilization may be more accurately described as “quasi-sterilization.”
Control of Microbial Growth: Non-Sterilizing Approaches
Sterilization protocols require procedures that are not practical, or necessary, in many settings. Various other methods are used in clinical and nonclinical settings to reduce the microbial load on items. Although the terms for these methods are often used interchangeably, there are important distinctions.
The type of protocol required to achieve the desired level of cleanliness depends on the particular item to be cleaned. For example, those used clinically are categorized as critical, semicritical, and noncritical. Critical items must be sterile because they will be used inside the body, often penetrating sterile tissues or the bloodstream; examples of critical items include surgical instruments, catheters, and intravenous fluids. Gastrointestinal endoscopes and various types of equipment for respiratory therapies are examples of semicritical items; they may contact mucous membranes or nonintact skin but do not penetrate tissues. Semicritical items do not typically need to be sterilized but do require a high level of disinfection. Items that may contact but not penetrate intact skin are noncritical items; examples are bed linens, furniture, crutches, stethoscopes, and blood pressure cuffs. These articles need to be clean but not highly disinfected.
Figure 1 summarizes common protocols, definitions, applications, and agents used to control microbial growth.
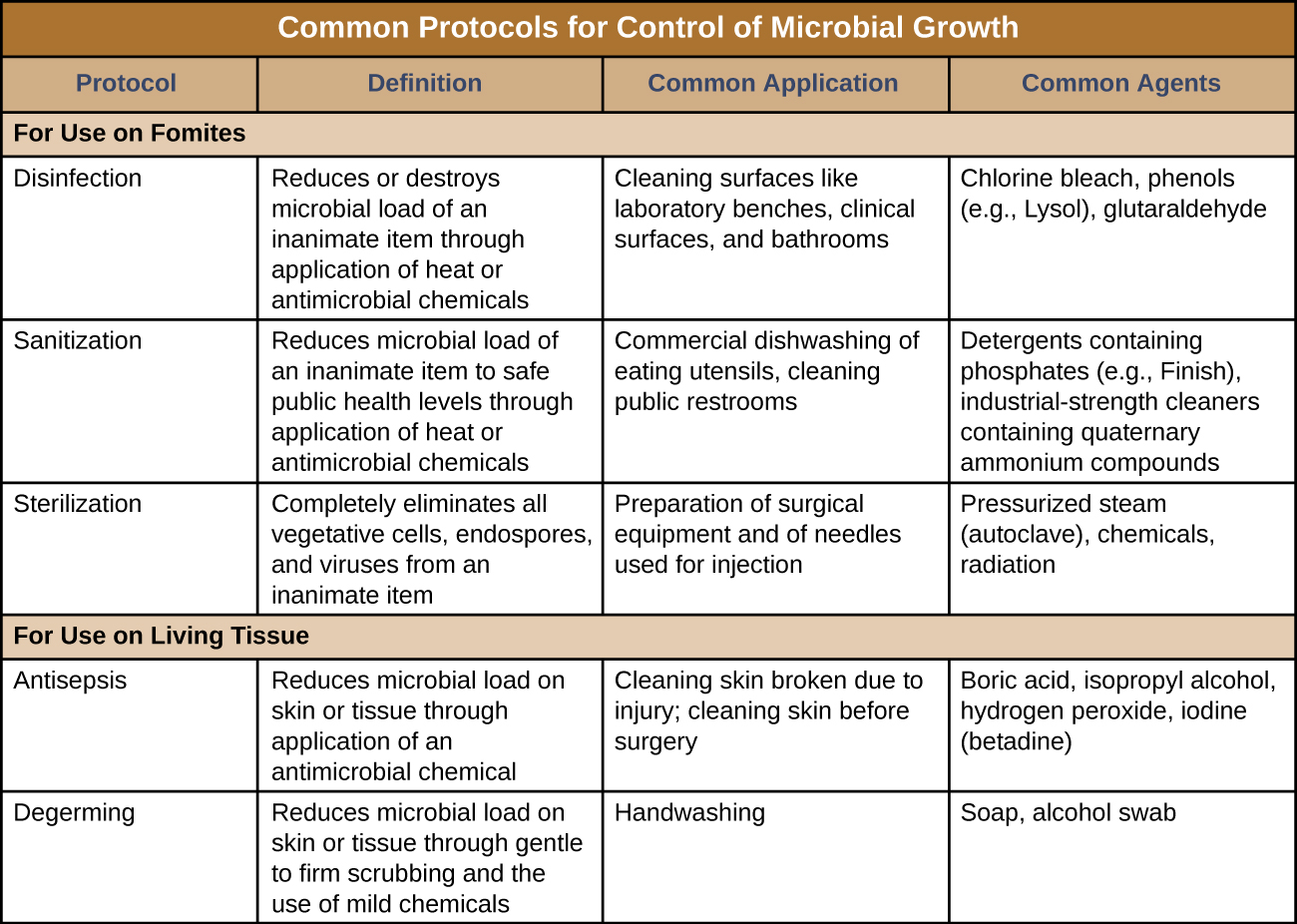
Figure 2: Summary of different approaches for reducing microbes. For use on fomites includes disinfection, sanitization, and sterilization. For use on living tissue involves antisepsis (for broken tissues and Preparation for surgery) and degerming (handwashing). The only method that completely eliminates all microbes is sterilization. All other approaches are for reducing microbial load.
Disinfection
The process of disinfection inactivates most microbes on the surface of a fomite by using antimicrobial chemicals or heat. Because some microbes remain, the disinfected item is not considered sterile. Ideally, disinfectants should be fast acting, stable, easy to prepare, inexpensive, and easy to use. An example of a natural disinfectant is vinegar; its acidity kills most microbes. Chemical disinfectants, such as chlorine bleach or products containing chlorine, are used to clean nonliving surfaces such as laboratory benches, clinical surfaces, and bathroom sinks. Typical disinfection does not lead to sterilization because endospores tend to survive even when all vegetative cells have been killed.
Antisepsis
Unlike disinfectants, antiseptics are antimicrobial chemicals safe for use on living skin or tissues. Examples of antiseptics include hydrogen peroxide and isopropyl alcohol. The process of applying an antiseptic is called antisepsis. In addition to the characteristics of a good disinfectant, antiseptics must also be selectively effective against microorganisms and able to penetrate tissue deeply without causing tissue damage.
Degerming
The act of handwashing is an example of degerming, in which microbial numbers are significantly reduced by gently scrubbing living tissue, most commonly skin, with a mild chemical (e.g., soap) to avoid the transmission of pathogenic microbes. Wiping the skin with an alcohol swab at an injection site is another example of degerming. These degerming methods remove most (but not all) microbes from the skin’s surface.
Sanitization
The term sanitization refers to the cleansing of fomites to remove enough microbes to achieve levels deemed safe for public health. For example, commercial dishwashers used in the food service industry typically use very hot water and air for washing and drying; the high temperatures kill most microbes, sanitizing the dishes. Surfaces in hospital rooms are commonly sanitized using a chemical disinfectant to prevent disease transmission between patients.
Physical Approaches for Controlling Microbial Growth
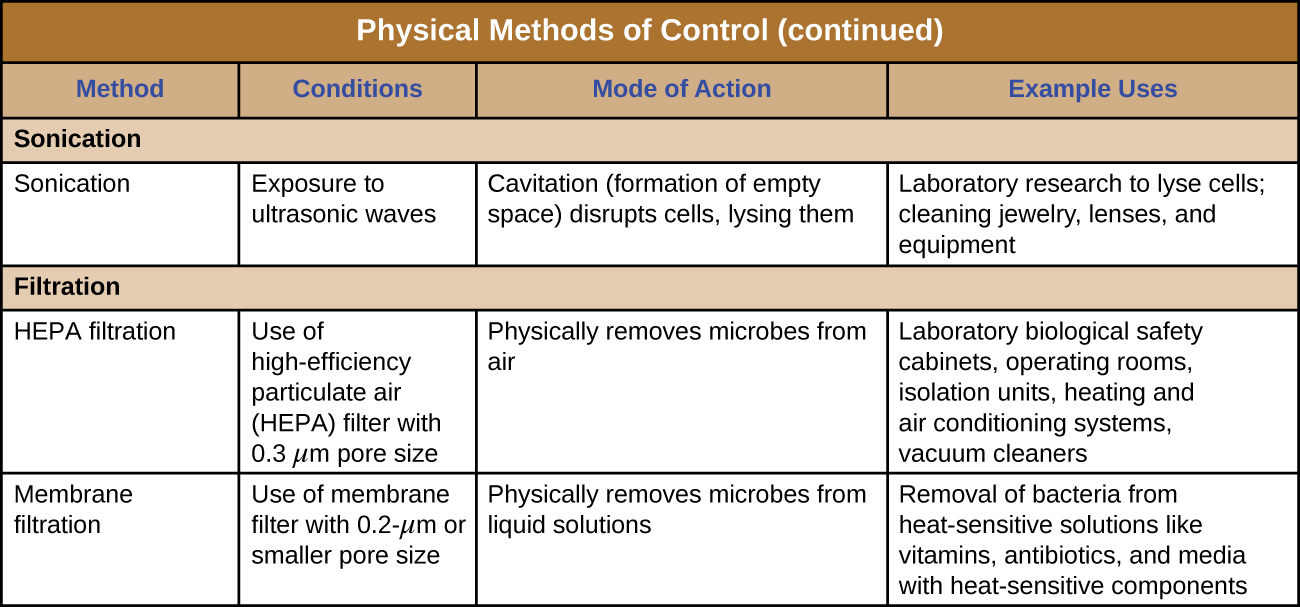
Figure 2: Summary of the physical methods for controlling microbes. These approaches include the following categories: heat, cold, pressure, desiccation, radiation, sonication, and filtration. There are multiple ways for controlling microbes within some of these categories. For example, controlling microbes with cold could involve refrigeration or it could involve freezing.
Chemical Approaches for Controlling Microbial Growth
Table 1: Chemical disinfectants can be used to control microbes. These types of chemicals fall into different chemical categories: phenolics, metals, halogens, alcohols, surfactants, bisbiguanides, alkylating agents, peroxygens, supercritical gases, chemical food preservatives, and natural food preservatives.
Chemical Disinfectants | ||
---|---|---|
Chemical | Mode of Action | Example Uses |
Phenolics | ||
Cresols o-Phenylphenol Hexachlorophene Triclosan |
Denature proteins and disrupt membranes | Disinfectant in Lysol Prevent contamination of crops (citrus) Antibacterial soap pHisoHex for handwashing in hospitals |
Metals | ||
Mercury Silver Copper Nickel Zinc |
Bind to proteins and inhibit enzyme activity | Topical antiseptic Treatment of wounds and burns Prevention of eye infections in newborns Antibacterial in catheters and bandages Mouthwash Algicide for pools and fish tanks Containers for long-term water storage |
Halogens | ||
Iodine Chlorine Fluorine |
Oxidation and destabilization of cellular macromolecules | Topical antiseptic Hand scrub for medical personnel Water disinfectant Water treatment plants Household bleach Food processing Prevention of dental carries |
Alcohols | ||
Ethanol Isopropanol |
Denature proteins and disrupt membranes | Disinfectant Antiseptic |
Surfactants | ||
Quaternary ammonium salts | Lowers surface tension of water to help with washing away of microbes, and disruption of cell membranes | Soaps and detergent Disinfectant Antiseptic Mouthwash |
Bisbiguanides | ||
Chlorhexidine Alexidine |
Disruption of cell membranes | Oral rinse Hand scrub for medical personnel |
Alkylating Agents | ||
Formaldehyde Glutaraldehyde o-Phthalaldehyde Ethylene oxide β-Propionolactone |
Inactivation of enzymes and nucleic acid | Disinfectant Tissue specimen storage Embalming Sterilization of medical equipment Vaccine component for sterility |
Peroxygens | ||
Hydrogen peroxide Peracetic acid Benzoyl peroxide Carbamide peroxide Ozone gas |
Oxidation and destabilization of cellular macromolecules | Antiseptic Disinfectant Acne medication Toothpaste ingredient |
Supercritical Gases | ||
Carbon dioxide | Penetrates cells, forms carbonic acid, lowers intracellular pH | Food preservation Disinfection of medical devices Disinfection of transplant tissues |
Chemical Food Preservatives | ||
Sorbic acid Benzoic acid Propionic acid Potassium sorbate Sodium benzoate Calcium propionate Sulfur dioxide Nitrites |
Decrease pH and inhibit enzymatic function | Preservation of food products |
Natural Food Preservatives | ||
Nisin Natamycin |
Inhibition of cell wall synthesis (Nisin) | Preservation of dairy products, meats, and beverages |
t-test Statistical Analysis
The t-test is a statistical analysis that enables scientists to objectively determine, based on their data and not their opinions, whether the results from one experimental treatment are statistically different from another experimental treatment. For example, in this experiment, we will be able to determine if the soap-cleaned surfaces produced a statistically significantly different number of microbial colonies on petri plates than the uncleaned surfaces. If the t-test indicates that there is not a statistical difference, regardless of what the means (i.e. averages) are an how different those means may appear, they are statistically considered not different from each other.
To determine if a pair of experimental treatments are statistically different from each other, consider the means (i.e. averages) and the t-test ranges:
- If the mean of one treatment falls into the t-test range of the other treatment, and the mean of the other treatment falls into the t-test range of the first treatment, these results indicate that these treatments are not statistically significantly different from each other.
- If the mean of one treatment falls outside of the t-test range of the other treatment, and the mean of the other treatment falls outside of the t-test range of the first treatment, these results indicate these treatments are statistically significantly different from each other.
uncleaned surface | soap cleaned surface | |
---|---|---|
mean number of colonies on the petri plate | 98 | 12 |
t-test range | 54 - 142 | 0 - 24 |
Solution
To determine if the microbial load on the uncleaned surface is statistically significantly different than the soap cleaned surface as the following questions:
- Does the mean of the uncleaned surface fall into the t-test range of the soap cleaned surface? Answer No. 98 does not fall between 0 and 24.
- Does the mean of the soap cleaned surface fall into the t-test range of the uncleaned surface? Answer: No. 12 does not fall between 54 and 142.
- Check with the bullet points above this example. According to those, since the means do not fit into the t-test ranges of the other experimental groups, they are statistically significantly different.
Conclusion Statement (Option 1)
The soap cleaned surface had statistically significantly lower microbial load than the uncleaned surface.
Conclusion Statement (Option 2)
The uncleaned surface had a statistically significantly higher microbial load than the soap cleaned surface.
disinfectant cleaned surface | soap cleaned surface | |
---|---|---|
mean number of colonies on the petri plate | 9 | 12 |
t-test range | 2 - 16 | 0 - 24 |
Solution
To determine if the microbial load on the disinfectant cleaned surface is statistically significantly different than the soap cleaned surface as the following questions:
- Does the mean of the disinfectant cleaned surface fall into the t-test range of the soap cleaned surface? Answer Yes. 9 falls between 0 and 24.
- Does the mean of the soap cleaned surface fall into the t-test range of the disinfectant cleaned surface? Answer: Yes. 12 falls between 2 and 16.
- Check with the bullet points above this example. According to those, since the means fall into the t-test ranges of the other experimental groups, they are not statistically significantly different.
Conclusion Statement (Option 1)
The soap cleaned surface did not have a statistically significantly different microbial load than the disinfectant cleaned surface.
Conclusion Statement (Option 2)
The disinfectant cleaned surface did not have a statistically significantly different microbial load than the soap cleaned surface.
Laboratory Instructions
Purpose
The goal of this experiment is to swab various surfaces for microbes and to compare the number of microorganisms that grow on a Petri plate from the same surface in three different conditions:
- The surface as it is, untreated
- The surface that has been cleaned with soap
- The surface that has been cleaned with disinfectant
Each person will choose a surface. Work as a group so that the surfaces you choose will be diverse and different. Surfaces may be anything that it will be safe to use soap and disinfectant on. Examples include:
- Door handles
- Pens/pencils
- Cell phones (being mindful of not getting sensitive parts wet when cleaning)
- The floor
- A shoe
- A lab bench
- Lab equipment
- A sink in the lab or the bathroom
- Other parts of the bathroom
- A computer mouse (being mindful of not getting sensitive parts wet when cleaning)
- Keys
- Anything else you can think of
Instructions
- Choose a surface you would like to examine for microbes.
- Label three petri plates with:
- Your name
- Your group number
- The sample being swabbed
- Label one of each as:
- uncleaned
- soap cleaned
- disinfectant cleaned
- Dip a sterile cotton swab into dH2O and then swab the surface of interest and streak across the Petri plate labeled "uncleaned."
- Clean a separate spot of that same surface with soap and wipe dry. Dip a sterile cotton swab into dH2O and then swab the surface you cleaned with soap and streak across the Petri plate labeled "soap cleaned."
- Clean a separate spot of that surface with disinfectant and wipe dry. Dip a sterile cotton swab into dH2O and then swab the surface you cleaned with disinfectant and streak the Petri plate labeled "disinfectant cleaned."
- Invert all plates and place in the class bin (these will be put in the incubator until next class).
Results & Questions
- The surface I am examining in this experiment is…
- I predict the following will have the most bacterial growth (circle one):
uncleaned soap cleaned disinfectant cleaned
- I predict the following will have the least bacterial growth (circle one):
uncleaned soap cleaned disinfectant cleaned
- Record your individual results (colony number) in the table below:
uncleaned |
soap cleaned |
disinfectant cleaned |
---|---|---|
|
|
- Record class results (average colony number) in the table below:
|
uncleaned |
soap cleaned |
disinfectant cleaned |
---|---|---|---|
mean (i.e. average) colony number |
|
|
|
t-test range |
|
|
|
- Discuss your results.
- Which treatment produced the most microbial growth?
- Which treatment produced the least microbial growth?
- See questions 2. and 3. above. Were your predictions for the control of bacterial growth correct? Explain your answer.
- What type of control of microbes did we employ in this laboratory – physical or chemical?
- Use your individual data to create a bar graph (you may use a graphing software or graph paper – handwritten graphs should be done neatly with a ruler). Your bar graph should have 3 bars, one for each Petri plate. The y-axis should be the number of colonies. Remember to label all axes and give your graph a title.
- Use your class data to create a bar graph (you may use a graphing software or graph paper – handwritten graphs should be done neatly with a ruler). Your bar graph should have 3 bars, one for each Petri plate. The y-axis should be the average number of colonies. Remember to label all axes and give your graph a title. Standard error should be shown as error bars above and below the average colony numbers on each bar of the bar graph.
- How to analyze data using t-test:
- Interpret results of the t-test analysis. Give a proper conclusion statement for these results indicating that you conducted a statistical analysis (i.e. use the phrase "statistically signigicant" or statistically significantly").
Graph Paper
Attributions
- Chapter Image: Hand disinfectant machine.jpg by Santeri Viinamäki is licensed under CC BY 4.0
- Lightblue empty grid.svg by Pieter Kuiper is in the public domain
- Microbiology by OpenStax is licensed under CC BY 4.0