1.37: Viruses and Viral Epidemic Simulation
- Page ID
- 102000
\( \newcommand{\vecs}[1]{\overset { \scriptstyle \rightharpoonup} {\mathbf{#1}} } \)
\( \newcommand{\vecd}[1]{\overset{-\!-\!\rightharpoonup}{\vphantom{a}\smash {#1}}} \)
\( \newcommand{\id}{\mathrm{id}}\) \( \newcommand{\Span}{\mathrm{span}}\)
( \newcommand{\kernel}{\mathrm{null}\,}\) \( \newcommand{\range}{\mathrm{range}\,}\)
\( \newcommand{\RealPart}{\mathrm{Re}}\) \( \newcommand{\ImaginaryPart}{\mathrm{Im}}\)
\( \newcommand{\Argument}{\mathrm{Arg}}\) \( \newcommand{\norm}[1]{\| #1 \|}\)
\( \newcommand{\inner}[2]{\langle #1, #2 \rangle}\)
\( \newcommand{\Span}{\mathrm{span}}\)
\( \newcommand{\id}{\mathrm{id}}\)
\( \newcommand{\Span}{\mathrm{span}}\)
\( \newcommand{\kernel}{\mathrm{null}\,}\)
\( \newcommand{\range}{\mathrm{range}\,}\)
\( \newcommand{\RealPart}{\mathrm{Re}}\)
\( \newcommand{\ImaginaryPart}{\mathrm{Im}}\)
\( \newcommand{\Argument}{\mathrm{Arg}}\)
\( \newcommand{\norm}[1]{\| #1 \|}\)
\( \newcommand{\inner}[2]{\langle #1, #2 \rangle}\)
\( \newcommand{\Span}{\mathrm{span}}\) \( \newcommand{\AA}{\unicode[.8,0]{x212B}}\)
\( \newcommand{\vectorA}[1]{\vec{#1}} % arrow\)
\( \newcommand{\vectorAt}[1]{\vec{\text{#1}}} % arrow\)
\( \newcommand{\vectorB}[1]{\overset { \scriptstyle \rightharpoonup} {\mathbf{#1}} } \)
\( \newcommand{\vectorC}[1]{\textbf{#1}} \)
\( \newcommand{\vectorD}[1]{\overrightarrow{#1}} \)
\( \newcommand{\vectorDt}[1]{\overrightarrow{\text{#1}}} \)
\( \newcommand{\vectE}[1]{\overset{-\!-\!\rightharpoonup}{\vphantom{a}\smash{\mathbf {#1}}}} \)
\( \newcommand{\vecs}[1]{\overset { \scriptstyle \rightharpoonup} {\mathbf{#1}} } \)
\( \newcommand{\vecd}[1]{\overset{-\!-\!\rightharpoonup}{\vphantom{a}\smash {#1}}} \)
\(\newcommand{\avec}{\mathbf a}\) \(\newcommand{\bvec}{\mathbf b}\) \(\newcommand{\cvec}{\mathbf c}\) \(\newcommand{\dvec}{\mathbf d}\) \(\newcommand{\dtil}{\widetilde{\mathbf d}}\) \(\newcommand{\evec}{\mathbf e}\) \(\newcommand{\fvec}{\mathbf f}\) \(\newcommand{\nvec}{\mathbf n}\) \(\newcommand{\pvec}{\mathbf p}\) \(\newcommand{\qvec}{\mathbf q}\) \(\newcommand{\svec}{\mathbf s}\) \(\newcommand{\tvec}{\mathbf t}\) \(\newcommand{\uvec}{\mathbf u}\) \(\newcommand{\vvec}{\mathbf v}\) \(\newcommand{\wvec}{\mathbf w}\) \(\newcommand{\xvec}{\mathbf x}\) \(\newcommand{\yvec}{\mathbf y}\) \(\newcommand{\zvec}{\mathbf z}\) \(\newcommand{\rvec}{\mathbf r}\) \(\newcommand{\mvec}{\mathbf m}\) \(\newcommand{\zerovec}{\mathbf 0}\) \(\newcommand{\onevec}{\mathbf 1}\) \(\newcommand{\real}{\mathbb R}\) \(\newcommand{\twovec}[2]{\left[\begin{array}{r}#1 \\ #2 \end{array}\right]}\) \(\newcommand{\ctwovec}[2]{\left[\begin{array}{c}#1 \\ #2 \end{array}\right]}\) \(\newcommand{\threevec}[3]{\left[\begin{array}{r}#1 \\ #2 \\ #3 \end{array}\right]}\) \(\newcommand{\cthreevec}[3]{\left[\begin{array}{c}#1 \\ #2 \\ #3 \end{array}\right]}\) \(\newcommand{\fourvec}[4]{\left[\begin{array}{r}#1 \\ #2 \\ #3 \\ #4 \end{array}\right]}\) \(\newcommand{\cfourvec}[4]{\left[\begin{array}{c}#1 \\ #2 \\ #3 \\ #4 \end{array}\right]}\) \(\newcommand{\fivevec}[5]{\left[\begin{array}{r}#1 \\ #2 \\ #3 \\ #4 \\ #5 \\ \end{array}\right]}\) \(\newcommand{\cfivevec}[5]{\left[\begin{array}{c}#1 \\ #2 \\ #3 \\ #4 \\ #5 \\ \end{array}\right]}\) \(\newcommand{\mattwo}[4]{\left[\begin{array}{rr}#1 \amp #2 \\ #3 \amp #4 \\ \end{array}\right]}\) \(\newcommand{\laspan}[1]{\text{Span}\{#1\}}\) \(\newcommand{\bcal}{\cal B}\) \(\newcommand{\ccal}{\cal C}\) \(\newcommand{\scal}{\cal S}\) \(\newcommand{\wcal}{\cal W}\) \(\newcommand{\ecal}{\cal E}\) \(\newcommand{\coords}[2]{\left\{#1\right\}_{#2}}\) \(\newcommand{\gray}[1]{\color{gray}{#1}}\) \(\newcommand{\lgray}[1]{\color{lightgray}{#1}}\) \(\newcommand{\rank}{\operatorname{rank}}\) \(\newcommand{\row}{\text{Row}}\) \(\newcommand{\col}{\text{Col}}\) \(\renewcommand{\row}{\text{Row}}\) \(\newcommand{\nul}{\text{Nul}}\) \(\newcommand{\var}{\text{Var}}\) \(\newcommand{\corr}{\text{corr}}\) \(\newcommand{\len}[1]{\left|#1\right|}\) \(\newcommand{\bbar}{\overline{\bvec}}\) \(\newcommand{\bhat}{\widehat{\bvec}}\) \(\newcommand{\bperp}{\bvec^\perp}\) \(\newcommand{\xhat}{\widehat{\xvec}}\) \(\newcommand{\vhat}{\widehat{\vvec}}\) \(\newcommand{\uhat}{\widehat{\uvec}}\) \(\newcommand{\what}{\widehat{\wvec}}\) \(\newcommand{\Sighat}{\widehat{\Sigma}}\) \(\newcommand{\lt}{<}\) \(\newcommand{\gt}{>}\) \(\newcommand{\amp}{&}\) \(\definecolor{fillinmathshade}{gray}{0.9}\)- Name the following virus types and virus structures: helical capsid, icosahedral capsid, enveloped virus, complex virus, nucleic acid (DNA or RNA)/viral genome, capsid, protein, spike proteins, envelope, sheath, tail fibers
- Tell that viruses are not cells, but are particles that are almost always smaller than cells.
- Participate in viral epidemic simulation.
- Use data from viral epidemic simulation to determine the original carrier of the "virus."
- Calculate incidence rate and prevalence rate.
- Define, use, and recognize and name examples of the following: epidemiology, etiology, morbidity, morbidity rate, prevalence, incidence, mortality, sporadic diseases, endemic diseases, epidemic diseases, pandemic diseases, causative agent, reservoirs, passive carriers, active carriers, asymptomatic carriers, direct contact transmission, droplet transmission, indirect contact transmission, vehicle transmission, mechanical transmission, mechanical vector, quarantine, nosocomial infections, healthcare-associated infections
Introduction to Viruses
Viruses are noncellular parasitic entities that cannot be classified within any living kingdom. They can infect organisms as diverse as bacteria, plants, and animals. In fact, viruses exist in a sort of netherworld between a living organism and a nonliving entity. Living things grow, metabolize, and reproduce. In contrast, viruses are not cellular, do not have a metabolism or grow, and cannot divide by cell division. Viruses can copy, or replicate themselves; however, they are entirely dependent on resources derived from their host cells to produce progeny viruses—which are assembled in their mature form. No one knows exactly when or how viruses evolved or from what ancestral source because viruses have not left a fossil record. Some virologists contend that modern viruses are a mosaic of bits and pieces of nucleic acids picked up from various sources along their respective evolutionary paths.
Viruses are diverse entities: They vary in structure, methods of replication, and the hosts they infect. Nearly all forms of life—from prokaryotic bacteria and archaeans, to eukaryotes such as plants, animals, and fungi—have viruses that infect them. While most biological diversity can be understood through evolutionary history (such as how species have adapted to changing environmental conditions and how different species are related to one another through common descent), much about virus origins and evolution remains unknown.

Figure 1: Electron micrograph of the SARS virus (red) attached to an infected cell (green). Note how very small virus particles are and they are not cellular.
Viruses were first discovered after the development of a porcelain filter—the Chamberland-Pasteur filter—that could remove all bacteria visible in the microscope from any liquid sample. In 1886, Adolph Meyer demonstrated that a disease of tobacco plants—tobacco mosaic disease—could be transferred from a diseased plant to a healthy one via liquid plant extracts. In 1892, Dmitri Ivanowski showed that this disease could be transmitted in this way even after the Chamberland-Pasteur filter had removed all viable bacteria from the extract. Still, it was many years before it was proved that these “filterable” infectious agents were not simply very small bacteria but were a new type of very small, disease-causing particle.
Virus Structures
Most virions, or single virus particles, are very small, about 20 to 250 nanometers in diameter. However, some recently discovered viruses from amoebae range up to 1000 nm in diameter. With the exception of large virions, like the poxvirus and other large DNA viruses, viruses cannot be seen with a light microscope. It was not until the development of the electron microscope in the late 1930s that scientists got their first good view of the structure of the tobacco mosaic virus, discussed above, and other viruses. The surface structure of virions can be observed by both scanning and transmission electron microscopy, whereas the internal structures of the virus can only be observed in images from a transmission electron microscope. The use of electron microscopy and other technologies has allowed for the discovery of many viruses of all types of living organisms.
*viruses in this interactive tool include: measles virus, hiv, phage, influenza virus, hepatitis virus, and rhinovirus
Viruses are noncellular, meaning they are biological entities that do not have a cellular structure. They therefore lack most of the components of cells, such as organelles, ribosomes, and the plasma membrane. A virion consists of a nucleic acid core (DNA or RNA), an outer protein coating or capsid, and sometimes an outer envelope made of protein and phospholipid membranes derived from the host cell. Viruses may also contain additional proteins, such as enzymes, within the capsid or attached to the viral genome. The most obvious difference between members of different viral families is the variation in their morphology, which is quite diverse. An interesting feature of viral complexity is that the complexity of the host does not necessarily correlate with the complexity of the virion. In fact, some of the most complex virion structures are found in the bacteriophages—viruses that infect the simplest living organisms, bacteria.
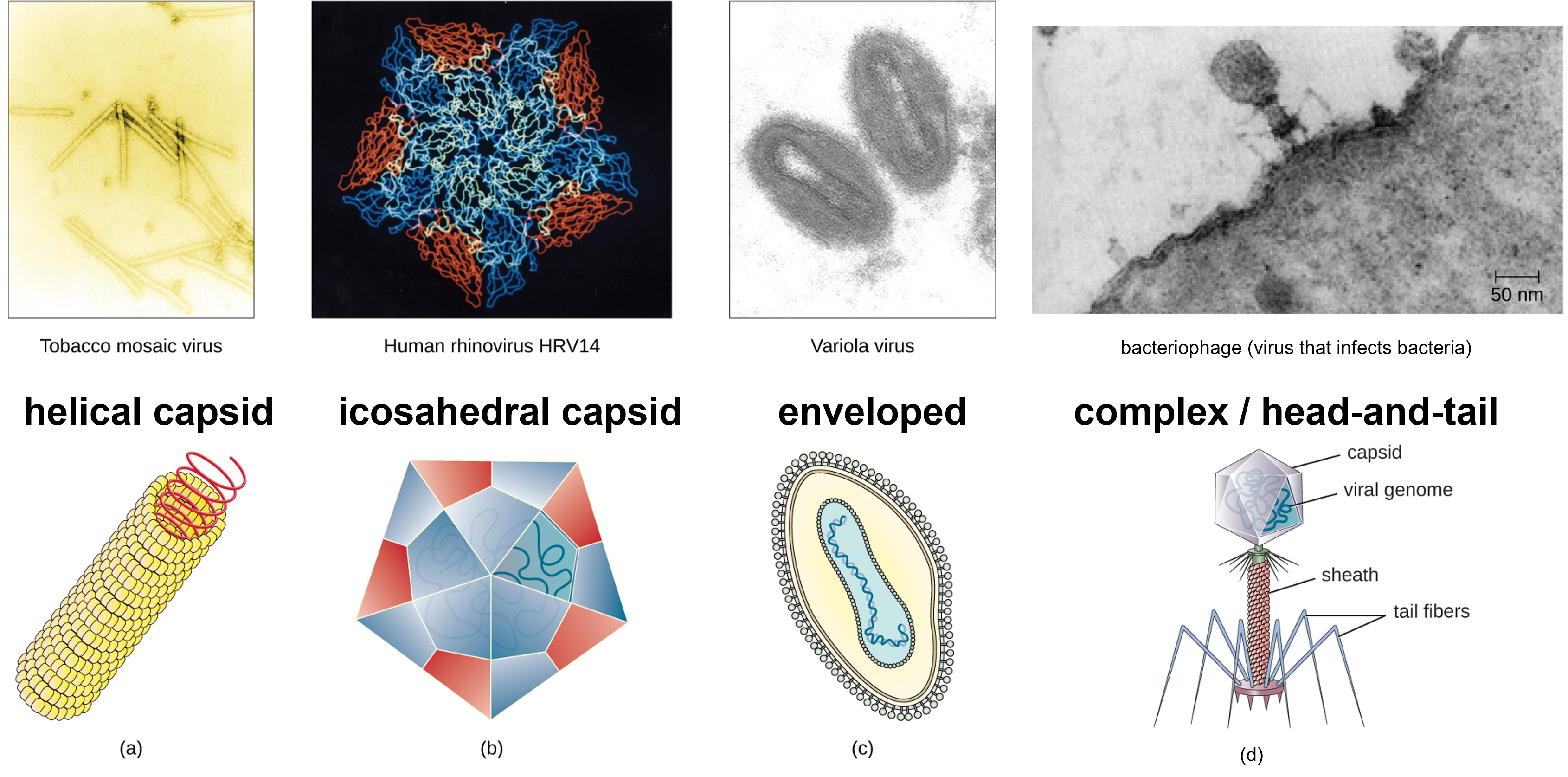
Figure 2: Viruses have diverse shapes. (a) Helical viruses have protein capsids form a hollow tube containing the viral genetic molecule(s). (b) Icosahedral viruses is a three-dimensional hollow structure formed by 20 faces (icosahedron) with the viral genetic material inside. (c) Enveloped viruses are surrounding by a lipid membrane that arose from the plasma membrane of a host cell. (d) Complex or head-and-tail viruses have a head-like structure formed by the capsid with genetic material inside, and a sheath or tail-like structure. (credit a “micrograph”: modification of work by USDA ARS; credit b “micrograph”: modification of work by U.S. Department of Energy) (credit d: modification of work by U.S. Department of Energy, Office of Science, LBL, PBD)
Viruses come in many shapes and sizes, but these features are consistent for each viral family. As we have seen, all virions have a nucleic acid genome covered by a protective capsid. The proteins of the capsid are encoded in the viral genome, and are called capsomeres. Some viral capsids are simple helices or polyhedral “spheres,” whereas others are quite complex in structure.
In general, viruses structures can be classified as: helical, icosahedral, enveloped, and complex, also known as head-and-tail. Helical capsids are long and cylindrical. Many plant viruses are helical, including TMV. Icosahedral viruses have shapes that are roughly spherical, such as those of poliovirus or herpesviruses. Enveloped viruses have membranes derived from the host cell that surrounds the capsids. Animal viruses, such as HIV, are frequently enveloped. Head-and-tail viruses infect bacteria and have a head that is similar to icosahedral viruses and a tail shaped like helical viruses.
Viral Reproduction
All viruses depend on cells for reproduction and metabolic processes. By themselves, viruses do not encode for all of the enzymes necessary for viral replication. But within a host cell, a virus can commandeer cellular machinery to produce more viral particles. Bacteriophages replicate only in the cytoplasm, since prokaryotic cells do not have a nucleus or organelles. In eukaryotic cells, most DNA viruses can replicate inside the nucleus, with an exception observed in the large DNA viruses, such as the poxviruses, that can replicate in the cytoplasm. With a few exceptions, RNA viruses that infect animal cells replicate in the cytoplasm. An important exception that will be highlighted later is Influenza virus.
The life cycle of bacteriophages has been a good model for understanding how viruses affect the cells they infect, since similar processes have been observed for eukaryotic viruses, which can cause immediate death of the cell or establish a latent or chronic infection.
The Lytic Cycle
During the lytic cycle of virulent phage, the bacteriophage takes over the cell, reproduces new phages, and destroys the cell. T-even phage is a good example of a well-characterized class of virulent phages. There are five stages in the bacteriophage lytic cycle. Attachment is the first stage in the infection process in which the phage interacts with specific bacterial surface receptors (e.g., lipopolysaccharides and OmpC protein on host surfaces). Most phages have a narrow host range and may infect one species of bacteria or one strain within a species. This unique recognition can be exploited for targeted treatment of bacterial infection by phage therapy or for phage typing to identify unique bacterial subspecies or strains. The second stage of infection is entry or penetration. This occurs through contraction of the tail sheath, which acts like a hypodermic needle to inject the viral genome through the cell wall and membrane. The phage head and remaining components remain outside the bacteria.

Figure 3: A virulent phage shows only the lytic cycle pictured here. In the lytic cycle, the phage replicates and lyses the host cell. 1. Attachment: the phage attaches to the surface of the host. 2. Penetration: the viral DNA enters the host cell. 3. Biosynthesis: phage DNA replicates and phage proteins are made. 4. Maturation: New phage particles are assembled. 5. Lysis: The cell lyses, releasing the newly made phages.
The third stage of infection is biosynthesis of new viral components. After entering the host cell, the virus synthesizes virus-encoded endonucleases to degrade the bacterial chromosome. It then hijacks the host cell to replicate, transcribe, and translate the necessary viral components (capsomeres, sheath, base plates, tail fibers, and viral enzymes) for the assembly of new viruses. Polymerase genes are usually expressed early in the cycle, while capsid and tail proteins are expressed later. During the maturation phase, new virions are created. To liberate free phages, the bacterial cell wall is disrupted by phage proteins such as holin or lysozyme. The final stage is release. Mature viruses burst out of the host cell in a process called lysis and the progeny viruses are liberated into the environment to infect new cells.
The Lysogenic Cycle
In a lysogenic cycle, the phage genome also enters the cell through attachment and penetration. A prime example of a phage with this type of life cycle is the lambda phage. During the lysogenic cycle, instead of killing the host, the phage genome integrates into the bacterial chromosome and becomes part of the host. The integrated phage genome is called a prophage. A bacterial host with a prophage is called a lysogen. The process in which a bacterium is infected by a temperate phage is called lysogeny. It is typical of temperate phages to be latent or inactive within the cell. As the bacterium replicates its chromosome, it also replicates the phage’s DNA and passes it on to new daughter cells during reproduction. The presence of the phage may alter the phenotype of the bacterium, since it can bring in extra genes (e.g., toxin genes that can increase bacterial virulence). This change in the host phenotype is called lysogenic conversion or phage conversion. Some bacteria, such as Vibrio cholerae and Clostridium botulinum, are less virulent in the absence of the prophage. The phages infecting these bacteria carry the toxin genes in their genome and enhance the virulence of the host when the toxin genes are expressed. In the case of V. cholera, phage encoded toxin can cause severe diarrhea; in C. botulinum, the toxin can cause paralysis. During lysogeny, the prophage will persist in the host chromosome until induction, which results in the excision of the viral genome from the host chromosome. After induction has occurred the temperate phage can proceed through a lytic cycle and then undergo lysogeny in a newly infected cell.
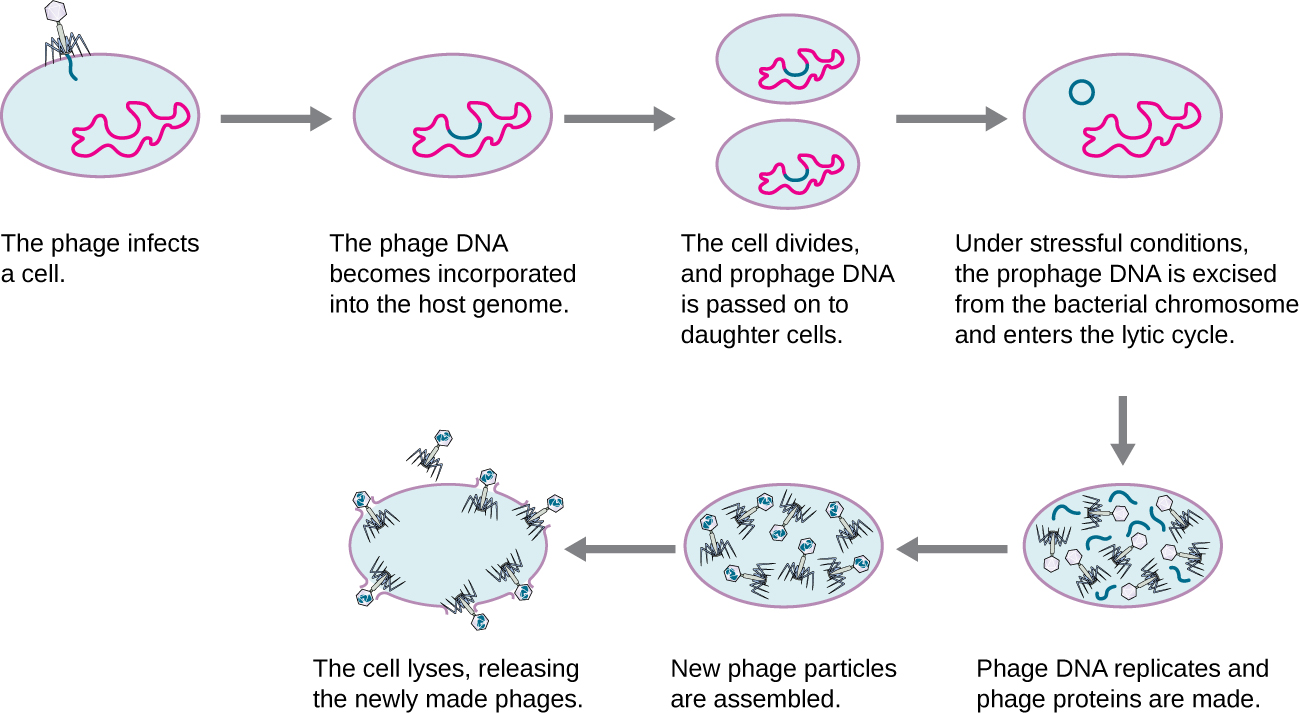
Figure 4: A temperate bacteriophage has both lytic and lysogenic cycles. In the lysogenic cycle, phage DNA is incorporated into the host genome, forming a prophage, which is passed on to subsequent generations of cells. Environmental stressors such as starvation or exposure to toxic chemicals may cause the prophage to be excised and enter the lytic cycle.
Epidemiology
In the United States and other developed nations, public health is a key function of government. A healthy citizenry is more productive, content, and prosperous; high rates of death and disease, on the other hand, can severely hamper economic productivity and foster social and political instability. The burden of disease makes it difficult for citizens to work consistently, maintain employment, and accumulate wealth to better their lives and support a growing economy.
Epidemiology is the science that underlies public health by examining the incidence, spread, transmission, and control of diseases in society. Epidemiology studies how disease originates and spreads throughout a population, with the goal of preventing outbreaks and containing them when they do occur. Over the past two centuries, discoveries in epidemiology have led to public health policies that have transformed life in developed nations, leading to the eradication (or near eradication) of many diseases (e.g. polio, smallpox, measles) that were once causes of great human suffering and premature death. However, the work of epidemiologists is far from finished. Numerous diseases continue to plague humanity, and new diseases are always emerging. Moreover, in the developing world, lack of infrastructure continues to pose many challenges to efforts to contain disease.
The field of epidemiology concerns the geographical distribution and timing of infectious disease occurrences and how they are transmitted and maintained in nature, with the goal of recognizing and controlling outbreaks. The science of epidemiology includes etiology (the study of the causes of disease) and investigation of disease transmission (mechanisms by which a disease is spread).
Analyzing Disease in a Population
Epidemiological analyses are always carried out with reference to a population, which is the group of individuals that are at risk for the disease or condition. The population can be defined geographically, but if only a portion of the individuals in that area are susceptible, additional criteria may be required. Susceptible individuals may be defined by particular behaviors, such as intravenous drug use, owning particular pets, or membership in an institution, such as a college. Being able to define the population is important because most measures of interest in epidemiology are made with reference to the size of the population.
The state of being diseased is called morbidity. Morbidity in a population can be expressed in a few different ways. Morbidity or total morbidity is expressed in numbers of individuals without reference to the size of the population. The morbidity rate can be expressed as the number of diseased individuals out of a standard number of individuals in the population, such as 100,000, or as a percent of the population.
There are two aspects of morbidity that are relevant to an epidemiologist: a disease’s prevalence and its incidence. Prevalence is the number, or proportion, of individuals with a particular illness in a given population at a point in time. For example, the Centers for Disease Control and Prevention (CDC) estimated that in 2012, there were about 1.2 million people 13 years and older with an active human immunodeficiency virus (HIV) infection. Expressed as a proportion, or rate, this is a prevalence of 467 infected persons per 100,000 in the population. On the other hand, incidence is the number or proportion of new cases in a period of time. For the same year and population, the CDC estimates that there were 43,165 newly diagnosed cases of HIV infection, which is an incidence of 13.7 new cases per 100,000 in the population. The relationship between incidence and prevalence can be seen in Figure 5. For a chronic disease like HIV infection, prevalence will generally be higher than incidence because it represents the cumulative number of new cases over many years minus the number of cases that are no longer active (e.g., because the patient died or was cured).
In addition to morbidity rates, the incidence and prevalence of mortality (death) may also be reported. A mortality rate can be expressed as the percentage of the population that has died from a disease or as the number of deaths per 100,000 persons (or other suitable standard number).

Figure 5: This graph compares the incidence of HIV (the number of new cases reported each year) with the prevalence (the total number of cases each year). Prevalence and incidence can also be expressed as a rate or proportion for a given population.
Patterns of Incidence
Diseases that are seen only occasionally, and usually without geographic concentration, are called sporadic diseases. Examples of sporadic diseases include tetanus, rabies, and plague. In the United States, Clostridium tetani, the bacterium that causes tetanus, is ubiquitous in the soil environment, but incidences of infection occur only rarely and in scattered locations because most individuals are vaccinated, clean wounds appropriately, or are only rarely in a situation that would cause infection. Likewise in the United States there are a few scattered cases of plague each year, usually contracted from rodents in rural areas in the western states.
Diseases that are constantly present (often at a low level) in a population within a particular geographic region are called endemic diseases. For example, malaria is endemic to some regions of Brazil, but is not endemic to the United States.
Diseases for which a larger than expected number of cases occurs in a short time within a geographic region are called epidemic diseases. Influenza is a good example of a commonly epidemic disease. Incidence patterns of influenza tend to rise each winter in the northern hemisphere. These seasonal increases are expected, so it would not be accurate to say that influenza is epidemic every winter; however, some winters have an usually large number of seasonal influenza cases in particular regions, and such situations would qualify as epidemics (Figure 6 and Figure 7).
An epidemic disease signals the breakdown of an equilibrium in disease frequency, often resulting from some change in environmental conditions or in the population. In the case of influenza, the disruption can be due to antigenic shift or drift (see Virulence Factors of Bacterial and Viral Pathogens), which allows influenza virus strains to circumvent the acquired immunity of their human hosts.
An epidemic that occurs on a worldwide scale is called a pandemic disease. For example, HIV/AIDS is a pandemic disease and novel influenza virus strains often become pandemic.
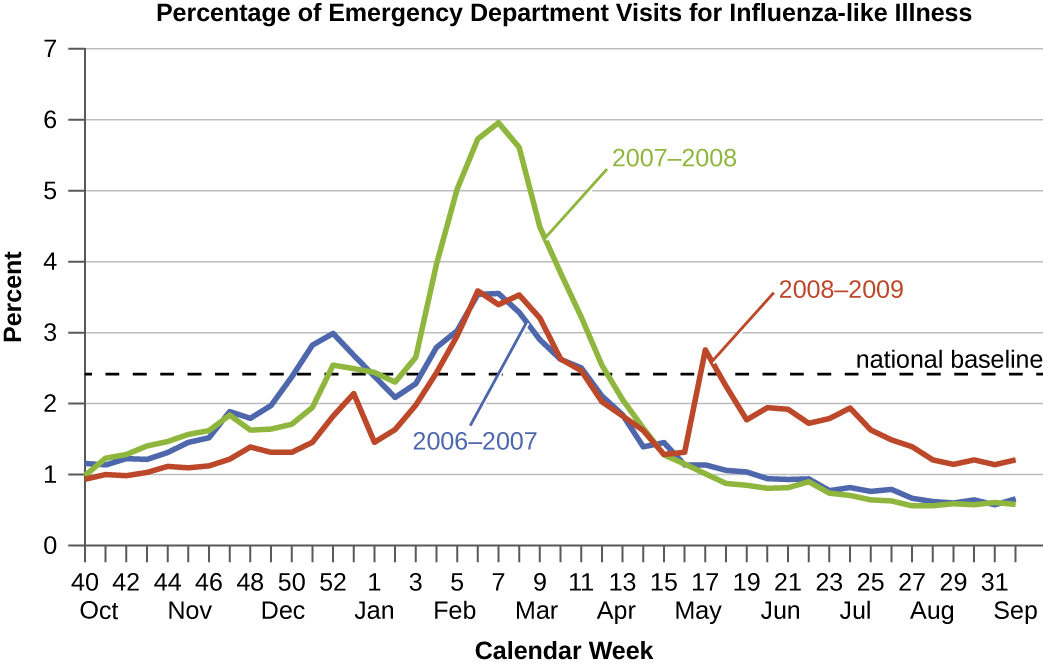
Figure 6: The 2007–2008 influenza season in the United States saw much higher than normal numbers of visits to emergency departments for influenza-like symptoms as compared to the previous and the following years. (credit: modification of work by Centers for Disease Control and Prevention)
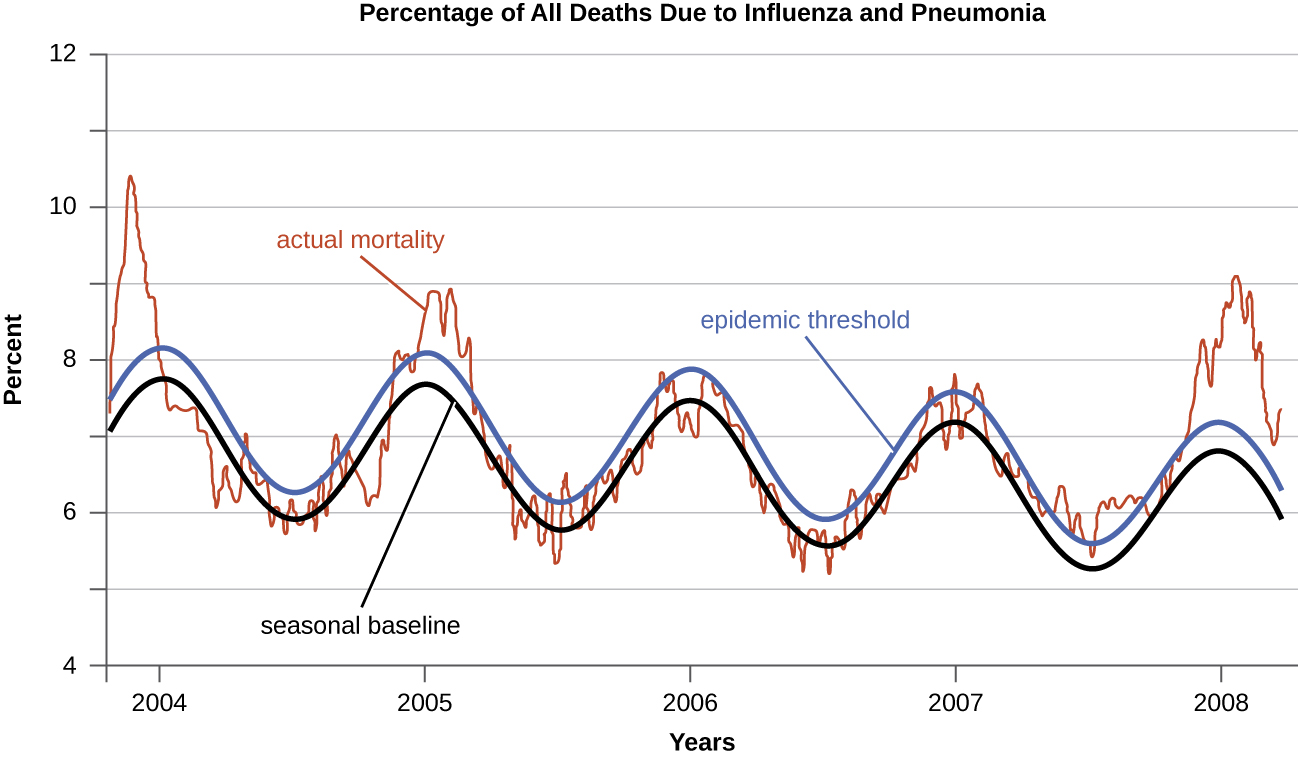
Figure 7: The seasonal epidemic threshold (blue curve) is set by the CDC-based data from the previous five years. When actual mortality rates exceed this threshold, a disease is considered to be epidemic. As this graph shows, pneumonia- and influenza-related mortality saw pronounced epidemics during the winters of 2003–2004, 2005, and 2008. (credit: modification of work by Centers for Disease Control and Prevention)
Etiology
When studying an epidemic, an epidemiologist’s first task is to determinate the cause of the disease, called the etiologic agent or causative agent. Connecting a disease to a specific pathogen can be challenging because of the extra effort typically required to demonstrate direct causation as opposed to a simple association. It is not enough to observe an association between a disease and a suspected pathogen; controlled experiments are needed to eliminate other possible causes. In addition, pathogens are typically difficult to detect when there is no immediate clue as to what is causing the outbreak. Signs and symptoms of disease are also commonly nonspecific, meaning that many different agents can give rise to the same set of signs and symptoms. This complicates diagnosis even when a causative agent is familiar to scientists.
Robert Koch was the first scientist to specifically demonstrate the causative agent of a disease (anthrax) in the late 1800s. Koch developed four criteria, now known as Koch’s postulates, which had to be met in order to positively link a disease with a pathogenic microbe. Without Koch’s postulates, the Golden Age of Microbiology would not have occurred. Between 1876 and 1905, many common diseases were linked with their etiologic agents, including cholera, diphtheria, gonorrhea, meningitis, plague, syphilis, tetanus, and tuberculosis. Today, we use the molecular Koch’s postulates, a variation of Koch’s original postulates that can be used to establish a link between the disease state and virulence traits unique to a pathogenic strain of a microbe.
Koch’s Postulates
- The suspected pathogen must be found in every case of disease and not be found in healthy individuals.
- The suspected pathogen can be isolated and grown in pure culture.
- A healthy test subject infected with the suspected pathogen must develop the same signs and symptoms of disease as seen in postulate 1.
- The pathogen must be re-isolated from the new host and must be identical to the pathogen from postulate 2.
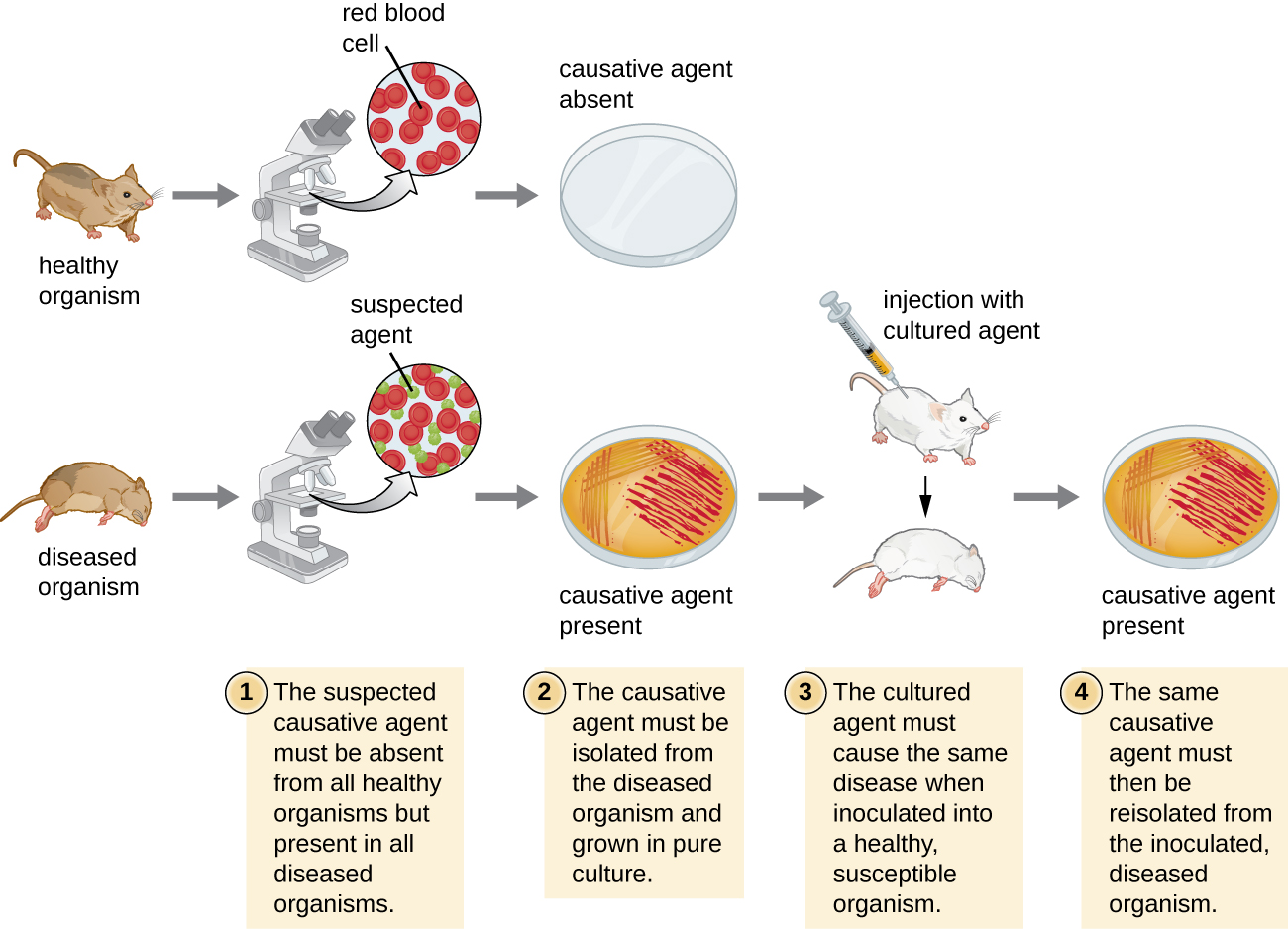
Figure 8: The steps for confirming that a pathogen is the cause of a particular disease using Koch’s postulates. 1. The suspected causative agent must be absent from all healthy organisms but present in all diseased organisms. 2. The causative agent must be isolated from the diseased organism and grown in pure culture. 3. The cultured agent must cause the same disease when inoculated into a healthy, susceptible organisms. 4. The same causative agent must then be reisolated from the inoculated, diseased organism.
Spread of Pathogens
Understanding how infectious pathogens spread is critical to preventing infectious disease. Many pathogens require a living host to survive, while others may be able to persist in a dormant state outside of a living host. But having infected one host, all pathogens must also have a mechanism of transfer from one host to another or they will die when their host dies. Pathogens often have elaborate adaptations to exploit host biology, behavior, and ecology to live in and move between hosts. Hosts have evolved defenses against pathogens, but because their rates of evolution are typically slower than their pathogens (because their generation times are longer), hosts are usually at an evolutionary disadvantage. This section will explore where pathogens survive—both inside and outside hosts—and some of the many ways they move from one host to another.
Reservoirs and Carriers
For pathogens to persist over long periods of time they require reservoirs where they normally reside. Reservoirs can be living organisms or nonliving locations. Nonliving reservoirs can include soil and water in the environment. These may naturally harbor the organism because it may grow in that environment. These environments may also become contaminated with pathogens in human feces, pathogens shed by intermediate hosts, or pathogens contained in the remains of intermediate hosts.
Pathogens may have mechanisms of dormancy or resilience that allow them to survive (but typically not to reproduce) for varying periods of time in nonliving environments. For example, Clostridium tetani survives in the soil and in the presence of oxygen as a resistant endospore. Although many viruses are soon destroyed once in contact with air, water, or other non-physiological conditions, certain types are capable of persisting outside of a living cell for varying amounts of time. For example, a study that looked at the ability of influenza viruses to infect a cell culture after varying amounts of time on a banknote showed survival times from 48 hours to 17 days, depending on how they were deposited on the banknote. On the other hand, cold-causing rhinoviruses are somewhat fragile, typically surviving less than a day outside of physiological fluids.
A human acting as a reservoir of a pathogen may or may not be capable of transmitting the pathogen, depending on the stage of infection and the pathogen. To help prevent the spread of disease among school children, the CDC has developed guidelines based on the risk of transmission during the course of the disease. For example, children with chickenpox are considered contagious for five days from the start of the rash, whereas children with most gastrointestinal illnesses should be kept home for 24 hours after the symptoms disappear.
An individual capable of transmitting a pathogen without displaying symptoms is referred to as a carrier. A passive carrier is contaminated with the pathogen and can mechanically transmit it to another host; however, a passive carrier is not infected. For example, a health-care professional who fails to wash their hands after seeing a patient harboring an infectious agent could become a passive carrier, transmitting the pathogen to another patient who becomes infected.
By contrast, an active carrier is an infected individual who can transmit the disease to others. An active carrier may or may not exhibit signs or symptoms of infection. For example, active carriers may transmit the disease during the incubation period (before they show signs and symptoms) or the period of convalescence (after symptoms have subsided). Active carriers who do not present signs or symptoms of disease despite infection are called asymptomatic carriers. Pathogens such as hepatitis B virus, herpes simplex virus, and HIV are frequently transmitted by asymptomatic carriers.
Mary Mallon, better known as Typhoid Mary, is a famous historical example of an asymptomatic carrier. An Irish immigrant, Mallon worked as a cook for households in and around New York City between 1900 and 1915. In each household, the residents developed typhoid fever (caused by Salmonella typhi) a few weeks after Mallon started working. Later investigations determined that Mallon was responsible for at least 122 cases of typhoid fever, five of which were fatal. See Eye on Ethics: Typhoid Mary for more about the Mallon case.
A pathogen may have more than one living reservoir. In zoonotic diseases, animals act as reservoirs of human disease and transmit the infectious agent to humans through direct or indirect contact. In some cases, the disease also affects the animal, but in other cases the animal is asymptomatic.
In parasitic infections, the parasite’s preferred host is called the definitive host. In parasites with complex life cycles, the definitive host is the host in which the parasite reaches sexual maturity. Some parasites may also infect one or more intermediate hosts in which the parasite goes through several immature life cycle stages or reproduces asexually.
Transmission
Regardless of the reservoir, transmission must occur for an infection to spread. First, transmission from the reservoir to the individual must occur. Then, the individual must transmit the infectious agent to other susceptible individuals, either directly or indirectly. Pathogenic microorganisms employ diverse transmission mechanisms.
Contact Transmission
Contact transmission includes direct contact or indirect contact. Person-to-person transmission is a form of direct contact transmission. Here the agent is transmitted by physical contact between two individuals (Figure 9) through actions such as touching, kissing, sexual intercourse, or droplet sprays. Direct contact can be categorized as vertical, horizontal, or droplet transmission. Vertical direct contact transmission occurs when pathogens are transmitted from mother to child during pregnancy, birth, or breastfeeding. Other kinds of direct contact transmission are called horizontal direct contact transmission. Often, contact between mucous membranes is required for entry of the pathogen into the new host, although skin-to-skin contact can lead to mucous membrane contact if the new host subsequently touches a mucous membrane. Contact transmission may also be site-specific; for example, some diseases can be transmitted by sexual contact but not by other forms of contact.
When an individual coughs or sneezes, small droplets of mucus that may contain pathogens are ejected. This leads to direct droplet transmission, which refers to droplet transmission of a pathogen to a new host over distances of one meter or less. A wide variety of diseases are transmitted by droplets, including influenza and many forms of pneumonia. Transmission over distances greater than one meter is called airborne transmission.
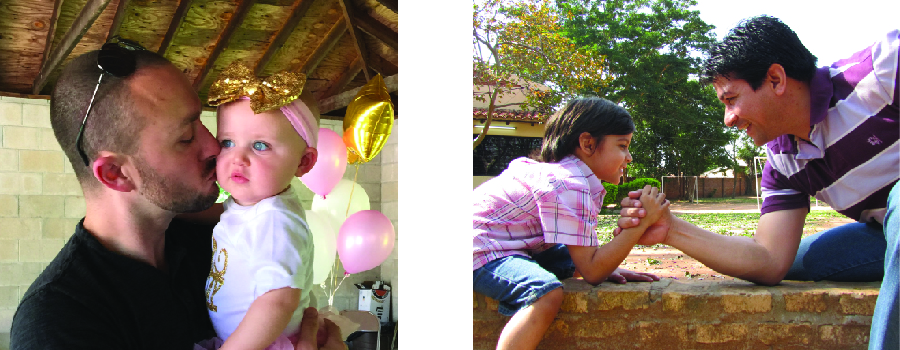
Figure 9: Direct contact transmission of pathogens can occur through physical contact. Many pathogens require contact with a mucous membrane to enter the body, but the host may transfer the pathogen from another point of contact (e.g., hand) to a mucous membrane (e.g., mouth or eye). (credit left: modification of work by Lisa Doehnert)
Indirect Contact Transmission
Indirect contact transmission involves inanimate objects called fomites that become contaminated by pathogens from an infected individual or reservoir (Figure 10). For example, an individual with the common cold may sneeze, causing droplets to land on a fomite such as a tablecloth or carpet, or the individual may wipe her nose and then transfer mucus to a fomite such as a doorknob or towel. Transmission occurs indirectly when a new susceptible host later touches the fomite and transfers the contaminated material to a susceptible portal of entry. Fomites can also include objects used in clinical settings that are not properly sterilized, such as syringes, needles, catheters, and surgical equipment. Pathogens transmitted indirectly via such fomites are a major cause of healthcare-associated infections (see Controlling Microbial Growth).

Figure 10: Fomites are nonliving objects that facilitate the indirect transmission of pathogens. Contaminated doorknobs, towels, and syringes are all common examples of fomites. (credit left: modification of work by Kate Ter Haar; credit middle: modification of work by Vernon Swanepoel; credit right: modification of work by “Zaldylmg”/Flickr)
Vehicle Transmission
The term vehicle transmission refers to the transmission of pathogens through vehicles such as water, food, and air. Water contamination through poor sanitation methods leads to waterborne transmission of disease. Waterborne disease remains a serious problem in many regions throughout the world. The World Health Organization (WHO) estimates that contaminated drinking water is responsible for more than 500,000 deaths each year. Similarly, food contaminated through poor handling or storage can lead to foodborne transmission of disease (Figure 11).
Dust and fine particles known as aerosols, which can float in the air, can carry pathogens and facilitate the airborne transmission of disease. For example, dust particles are the dominant mode of transmission of hantavirus to humans. Hantavirus is found in mouse feces, urine, and saliva, but when these substances dry, they can disintegrate into fine particles that can become airborne when disturbed; inhalation of these particles can lead to a serious and sometimes fatal respiratory infection.
Although droplet transmission over short distances is considered contact transmission as discussed above, longer distance transmission of droplets through the air is considered vehicle transmission. Unlike larger particles that drop quickly out of the air column, fine mucus droplets produced by coughs or sneezes can remain suspended for long periods of time, traveling considerable distances. In certain conditions, droplets desiccate quickly to produce a droplet nucleus that is capable of transmitting pathogens; air temperature and humidity can have an impact on effectiveness of airborne transmission.
Tuberculosis is often transmitted via airborne transmission when the causative agent, Mycobacterium tuberculosis, is released in small particles with coughs. Because tuberculosis requires as few as 10 microbes to initiate a new infection, patients with tuberculosis must be treated in rooms equipped with special ventilation, and anyone entering the room should wear a mask.

Figure 11: Food is an important vehicle of transmission for pathogens, especially of the gastrointestinal and upper respiratory systems. Notice the glass shield above the food trays, designed to prevent pathogens ejected in coughs and sneezes from entering the food. (credit: Fort George G. Meade Public Affairs Office)
Vector Transmission
Diseases can also be transmitted by a mechanical or biological vector, an animal (typically an arthropod) that carries the disease from one host to another. Mechanical transmission is facilitated by a mechanical vector, an animal that carries a pathogen from one host to another without being infected itself. For example, a fly may land on fecal matter and later transmit bacteria from the feces to food that it lands on; a human eating the food may then become infected by the bacteria, resulting in a case of diarrhea or dysentery (Figure 12).
Biological transmission occurs when the pathogen reproduces within a biological vector that transmits the pathogen from one host to another (Figure 12). Arthropods are the main vectors responsible for biological transmission (Figure 13). Most arthropod vectors transmit the pathogen by biting the host, creating a wound that serves as a portal of entry. The pathogen may go through part of its reproductive cycle in the gut or salivary glands of the arthropod to facilitate its transmission through the bite. For example, hemipterans (called “kissing bugs” or “assassin bugs”) transmit Chagas disease to humans by defecating when they bite, after which the human scratches or rubs the infected feces into a mucous membrane or break in the skin.
Biological insect vectors include mosquitoes, which transmit malaria and other diseases, and lice, which transmit typhus. Other arthropod vectors can include arachnids, primarily ticks, which transmit Lyme disease and other diseases, and mites, which transmit scrub typhus and rickettsial pox. Biological transmission, because it involves survival and reproduction within a parasitized vector, complicates the biology of the pathogen and its transmission. There are also important non-arthropod vectors of disease, including mammals and birds. Various species of mammals can transmit rabies to humans, usually by means of a bite that transmits the rabies virus. Chickens and other domestic poultry can transmit avian influenza to humans through direct or indirect contact with avian influenza virus A shed in the birds’ saliva, mucous, and feces.

Figure 12: (a) A mechanical vector carries a pathogen on its body from one host to another, not as an infection. 1. Fly picks up pathogen from fecal matter and carries it on its body. 2. Fly transfers pathogen to food. 3. Person eats contaminated food and then gets sick. (b) A biological vector carries a pathogen from one host to another after becoming infected itself. 1. Infected mosquito bites uninfected person. 2. Infection spreads through body and into red blood cells. 3. Second mosquito bites infected person. Mosquito may now transmit infection to another person.

Figure 13: (credit “Black fly”, “Tick”, “Tsetse fly”: modification of work by USDA; credit: “Flea”: modification of work by Centers for Disease Control and Prevention; credit: “Louse”, “Mosquito”, “Sand fly”: modification of work by James Gathany, Centers for Disease Control and Prevention; credit “Kissing bug”: modification of work by Glenn Seplak; credit “Mite”: modification of work by Michael Wunderli)
Quarantining
Individuals suspected or known to have been exposed to certain contagious pathogens may be quarantined, or isolated to prevent transmission of the disease to others. Hospitals and other health-care facilities generally set up special wards to isolate patients with particularly hazardous diseases such as tuberculosis or Ebola (Figure 16.15). Depending on the setting, these wards may be equipped with special air-handling methods, and personnel may implement special protocols to limit the risk of transmission, such as personal protective equipment or the use of chemical disinfectant sprays upon entry and exit of medical personnel.
The duration of the quarantine depends on factors such as the incubation period of the disease and the evidence suggestive of an infection. The patient may be released if signs and symptoms fail to materialize when expected or if preventive treatment can be administered in order to limit the risk of transmission. If the infection is confirmed, the patient may be compelled to remain in isolation until the disease is no longer considered contagious.
In the United States, public health authorities may only quarantine patients for certain diseases, such as cholera, diphtheria, infectious tuberculosis, and strains of influenza capable of causing a pandemic. Individuals entering the United States or moving between states may be quarantined by the CDC if they are suspected of having been exposed to one of these diseases. Although the CDC routinely monitors entry points to the United States for crew or passengers displaying illness, quarantine is rarely implemented.
Healthcare-Associated (Nosocomial) Infections
Hospitals, retirement homes, and prisons attract the attention of epidemiologists because these settings are associated with increased incidence of certain diseases. Higher rates of transmission may be caused by characteristics of the environment itself, characteristics of the population, or both. Consequently, special efforts must be taken to limit the risks of infection in these settings.
Infections acquired in health-care facilities, including hospitals, are called nosocomial infections or healthcare-associated infections (HAI). HAIs are often connected with surgery or other invasive procedures that provide the pathogen with access to the portal of infection. For an infection to be classified as an HAI, the patient must have been admitted to the health-care facility for a reason other than the infection. In these settings, patients suffering from primary disease are often afflicted with compromised immunity and are more susceptible to secondary infection and opportunistic pathogens.
In 2011, more than 720,000 HAIs occurred in hospitals in the United States, according to the CDC. About 22% of these HAIs occurred at a surgical site, and cases of pneumonia accounted for another 22%; urinary tract infections accounted for an additional 13%, and primary bloodstream infections 10%. Such HAIs often occur when pathogens are introduced to patients’ bodies through contaminated surgical or medical equipment, such as catheters and respiratory ventilators. Health-care facilities seek to limit nosocomial infections through training and hygiene protocols such as those described in Control of Microbial Growth.
Laboratory Instructions
Viral Epidemic Scenario
One of the most rapid ways for a pathogen to spread is when people are asymptomatic carriers or when people are in the incubation period before symptoms occur. Since these people are not experiencing symptoms, they are unaware that they are carrying a pathogen and able to spread that pathogen. This is particularly true when the incubation period is lengthy (length of the incubation period is different for different pathogens).
You are among a population who is at risk of catching a virus at the start of an epidemic. Everyone in the population begins either as an uninfected person, except one person who is an asymptomatic carrier of the virus. No one knows who the infected person is including that person.
This virus passes when two people are in close proximity and the carrier exhales respiratory droplets into the air. The uninfected person inhales respiratory droplets from the nearby carrier and infection then occurs.
As you go about normal day to day life in this scenario, you will come into contact with different individuals in the classroom each "day." Each "day" you will interact with one classmate and breath each others respiratory droplets. You will not know until the end whether you were the original infected person, or if you become infected and transmit the infection to others.
Epidemic Day 1
A well plate will be used to track fluid samples from each participant.
- Choose/be assigned a vial number.
- Collect the vial with your number and a transfer pipet.
- Write your vial number down in the Results & Questions section below.
- Put 5 drops from your vial into the well on the well plate corresponding with your vial number and epidemic day 1.
Epidemic Day 2 (Transfer #1)
- Names will be randomly chosen using a group randomizer.
- Find the person you are paired with and write their vial number in the table for epidemic day 2 in the Results & Questions section below.
- Use your transfer pipet to collect fluid from your vial. Your partner will do the same.
- Drop 5 drops from your transfer pipet into your partner's vial (this represents you transferring your respiratory droplets to your partner).
- Your partner will drop 5 drops from their transfer pipet to your vial (this represents your partner transferring their respiratory droplets to you).
- Put any liquid remaining in the transfer pipet back into your own vial.
- Close your vial securely and turn the vial upside-down 10 times to mix the vial well.
- Collect liquid from your vial with the transfer pipet.
- Put 5 drops from your vial into the well on the well plate corresponding with your vial number and epidemic day 2.
- Put any liquid remaining in the transfer pipet back into your own vial.
Epidemic Day 3 (Transfer #2)
- Names will be randomly chosen using a group randomizer.
- Find the person you are paired with and write their vial number in the table for epidemic day 3 in the Results & Questions section below.
- Use your transfer pipet to collect fluid from your vial. Your partner will do the same.
- Drop 5 drops from your transfer pipet into your partner's vial (this represents you transferring your respiratory droplets to your partner).
- Your partner will drop 5 drops from their transfer pipet to your vial (this represents your partner transferring their respiratory droplets to you).
- Put any liquid remaining in the transfer pipet back into your own vial.
- Close your vial securely and turn the vial upside-down 10 times to mix the vial well.
- Collect liquid from your vial with the transfer pipet.
- Put 5 drops from your vial into the well on the well plate corresponding with your vial number and epidemic day 3.
- Put any liquid remaining in the transfer pipet back into your own vial.
Epidemic Day 4 (Transfer #3)
- Names will be randomly chosen using a group randomizer.
- Find the person you are paired with and write their vial number in the table for epidemic day 4 in the Results & Questions section below.
- Use your transfer pipet to collect fluid from your vial. Your partner will do the same.
- Drop 5 drops from your transfer pipet into your partner's vial (this represents you transferring your respiratory droplets to your partner).
- Your partner will drop 5 drops from their transfer pipet to your vial (this represents your partner transferring their respiratory droplets to you).
- Put any liquid remaining in the transfer pipet back into your own vial.
- Close your vial securely and turn the vial upside-down 10 times to mix the vial well.
- Collect liquid from your vial with the transfer pipet.
- Put 5 drops from your vial into the well on the well plate corresponding with your vial number and epidemic day 4.
- Put any liquid remaining in the transfer pipet back into your own vial.
Revealing the Viral Infections & Spread of Virus in the Population
- After epidemic day 4, your instructor will place a single drop of a solution into each person's vial. If the vial turns red, you are "infected" with the virus.
- If you are infected, fill out the table on the board to indicate your vial number and the vial numbers you exchanged "respiratory drops" with.
- Your instructor will also use the well plate to determine the number of "infected" people in the population each day if the epidemic. They will share this information with the class so you can calculate the prevalence rates and incidence rates.
Results & Questions
My vial number is: _______
Epidemic Day | Vial # Respiratory Drops Exchanged With |
---|---|
2 | |
3 | |
4 |
Vial # | Mark with 'X' if infected on Epidemic Day 4 | Vials that Exchanged Respiratory Droplets with this Vial |
---|---|---|
1 | ||
2 | ||
3 | ||
4 | ||
5 | ||
6 | ||
7 | ||
8 | ||
9 | ||
10 | ||
11 | ||
12 | ||
13 | ||
14 | ||
15 | ||
16 | ||
17 | ||
18 | ||
19 | ||
20 | ||
21 | ||
22 | ||
23 | ||
24 | ||
25 | ||
26 |
- Fill out the table above for each of the epidemic days.
- Fill out the table above to indicate which of the vials were positive for the virus and which vials the virus-positive vials exchanged respiratory droplets with.
- Put your epidemiologist hat on. Use the information above to determine which vial was the original one that was infected. Which vial contained virus on epidemic day 1? How can you tell?
- How many chances did people in this scenario have to become "infected?"
- At the beginning of this viral epidemic scenario, only one person was "infected" with the virus. How many people were "infected" with the virus by epidemic day 4?
- Explain why there were more people who were "infected" at the end of the scenario than the number of fluid exchanges.
- What type or types of transmission occurred in this scenario (direct contact transmission, droplet transmission, indirect contact transmission, vehicle transmission, mechanical transmission)
- When a person is asymptomatic, are they aware that they are spreading a pathogen?
- Calculate the prevalence rate and incidence rate of the virus in the population for each epidemic day.
-
Epidemic Day Prevalence Rate Incidence Rate 1 2 3 4
-
- Define the following terms:
- epidemiology:
- etiology:
- morbidity:
- morbidity rate:
- prevalence:
- incidence:
- mortality:
- sporadic diseases:
- endemic diseases:
- epidemic diseases:
- pandemic diseases:
- causative agent:
- reservoirs:
- passive carriers:
- active carriers:
- asymptomatic carriers:
- direct contact transmission:
- droplet transmission:
- indirect contact transmission:
- vehicle transmission:
- mechanical transmission:
- mechanical vector:
- quarantine:
- nosocomial infections:
- healthcare-associated infections:
Attributions
- Chapter Image: Covid-19 SP - Santo Andre's hospital at peak of pandemic 02.jpg by Gustavo Basso is licensed under CC BY-SA 4.0
- Apoplast and symplast pathways.svg by Jackacon, vectorised by Smartse is in the public domain
- Biology 2e by OpenStax is licensed under CC BY 4.0
- Microbiology by OpenStax is licensed under CC BY 4.0
- SARS Virus Particles (43093982224).jpg by NIAID is in the public domain
- TMV Structure.png by Graham Colm is licsensed under CC BY-SA 3.0