9.4: Microevolution
- Page ID
- 16770
\( \newcommand{\vecs}[1]{\overset { \scriptstyle \rightharpoonup} {\mathbf{#1}} } \)
\( \newcommand{\vecd}[1]{\overset{-\!-\!\rightharpoonup}{\vphantom{a}\smash {#1}}} \)
\( \newcommand{\id}{\mathrm{id}}\) \( \newcommand{\Span}{\mathrm{span}}\)
( \newcommand{\kernel}{\mathrm{null}\,}\) \( \newcommand{\range}{\mathrm{range}\,}\)
\( \newcommand{\RealPart}{\mathrm{Re}}\) \( \newcommand{\ImaginaryPart}{\mathrm{Im}}\)
\( \newcommand{\Argument}{\mathrm{Arg}}\) \( \newcommand{\norm}[1]{\| #1 \|}\)
\( \newcommand{\inner}[2]{\langle #1, #2 \rangle}\)
\( \newcommand{\Span}{\mathrm{span}}\)
\( \newcommand{\id}{\mathrm{id}}\)
\( \newcommand{\Span}{\mathrm{span}}\)
\( \newcommand{\kernel}{\mathrm{null}\,}\)
\( \newcommand{\range}{\mathrm{range}\,}\)
\( \newcommand{\RealPart}{\mathrm{Re}}\)
\( \newcommand{\ImaginaryPart}{\mathrm{Im}}\)
\( \newcommand{\Argument}{\mathrm{Arg}}\)
\( \newcommand{\norm}[1]{\| #1 \|}\)
\( \newcommand{\inner}[2]{\langle #1, #2 \rangle}\)
\( \newcommand{\Span}{\mathrm{span}}\) \( \newcommand{\AA}{\unicode[.8,0]{x212B}}\)
\( \newcommand{\vectorA}[1]{\vec{#1}} % arrow\)
\( \newcommand{\vectorAt}[1]{\vec{\text{#1}}} % arrow\)
\( \newcommand{\vectorB}[1]{\overset { \scriptstyle \rightharpoonup} {\mathbf{#1}} } \)
\( \newcommand{\vectorC}[1]{\textbf{#1}} \)
\( \newcommand{\vectorD}[1]{\overrightarrow{#1}} \)
\( \newcommand{\vectorDt}[1]{\overrightarrow{\text{#1}}} \)
\( \newcommand{\vectE}[1]{\overset{-\!-\!\rightharpoonup}{\vphantom{a}\smash{\mathbf {#1}}}} \)
\( \newcommand{\vecs}[1]{\overset { \scriptstyle \rightharpoonup} {\mathbf{#1}} } \)
\( \newcommand{\vecd}[1]{\overset{-\!-\!\rightharpoonup}{\vphantom{a}\smash {#1}}} \)
\(\newcommand{\avec}{\mathbf a}\) \(\newcommand{\bvec}{\mathbf b}\) \(\newcommand{\cvec}{\mathbf c}\) \(\newcommand{\dvec}{\mathbf d}\) \(\newcommand{\dtil}{\widetilde{\mathbf d}}\) \(\newcommand{\evec}{\mathbf e}\) \(\newcommand{\fvec}{\mathbf f}\) \(\newcommand{\nvec}{\mathbf n}\) \(\newcommand{\pvec}{\mathbf p}\) \(\newcommand{\qvec}{\mathbf q}\) \(\newcommand{\svec}{\mathbf s}\) \(\newcommand{\tvec}{\mathbf t}\) \(\newcommand{\uvec}{\mathbf u}\) \(\newcommand{\vvec}{\mathbf v}\) \(\newcommand{\wvec}{\mathbf w}\) \(\newcommand{\xvec}{\mathbf x}\) \(\newcommand{\yvec}{\mathbf y}\) \(\newcommand{\zvec}{\mathbf z}\) \(\newcommand{\rvec}{\mathbf r}\) \(\newcommand{\mvec}{\mathbf m}\) \(\newcommand{\zerovec}{\mathbf 0}\) \(\newcommand{\onevec}{\mathbf 1}\) \(\newcommand{\real}{\mathbb R}\) \(\newcommand{\twovec}[2]{\left[\begin{array}{r}#1 \\ #2 \end{array}\right]}\) \(\newcommand{\ctwovec}[2]{\left[\begin{array}{c}#1 \\ #2 \end{array}\right]}\) \(\newcommand{\threevec}[3]{\left[\begin{array}{r}#1 \\ #2 \\ #3 \end{array}\right]}\) \(\newcommand{\cthreevec}[3]{\left[\begin{array}{c}#1 \\ #2 \\ #3 \end{array}\right]}\) \(\newcommand{\fourvec}[4]{\left[\begin{array}{r}#1 \\ #2 \\ #3 \\ #4 \end{array}\right]}\) \(\newcommand{\cfourvec}[4]{\left[\begin{array}{c}#1 \\ #2 \\ #3 \\ #4 \end{array}\right]}\) \(\newcommand{\fivevec}[5]{\left[\begin{array}{r}#1 \\ #2 \\ #3 \\ #4 \\ #5 \\ \end{array}\right]}\) \(\newcommand{\cfivevec}[5]{\left[\begin{array}{c}#1 \\ #2 \\ #3 \\ #4 \\ #5 \\ \end{array}\right]}\) \(\newcommand{\mattwo}[4]{\left[\begin{array}{rr}#1 \amp #2 \\ #3 \amp #4 \\ \end{array}\right]}\) \(\newcommand{\laspan}[1]{\text{Span}\{#1\}}\) \(\newcommand{\bcal}{\cal B}\) \(\newcommand{\ccal}{\cal C}\) \(\newcommand{\scal}{\cal S}\) \(\newcommand{\wcal}{\cal W}\) \(\newcommand{\ecal}{\cal E}\) \(\newcommand{\coords}[2]{\left\{#1\right\}_{#2}}\) \(\newcommand{\gray}[1]{\color{gray}{#1}}\) \(\newcommand{\lgray}[1]{\color{lightgray}{#1}}\) \(\newcommand{\rank}{\operatorname{rank}}\) \(\newcommand{\row}{\text{Row}}\) \(\newcommand{\col}{\text{Col}}\) \(\renewcommand{\row}{\text{Row}}\) \(\newcommand{\nul}{\text{Nul}}\) \(\newcommand{\var}{\text{Var}}\) \(\newcommand{\corr}{\text{corr}}\) \(\newcommand{\len}[1]{\left|#1\right|}\) \(\newcommand{\bbar}{\overline{\bvec}}\) \(\newcommand{\bhat}{\widehat{\bvec}}\) \(\newcommand{\bperp}{\bvec^\perp}\) \(\newcommand{\xhat}{\widehat{\xvec}}\) \(\newcommand{\vhat}{\widehat{\vvec}}\) \(\newcommand{\uhat}{\widehat{\uvec}}\) \(\newcommand{\what}{\widehat{\wvec}}\) \(\newcommand{\Sighat}{\widehat{\Sigma}}\) \(\newcommand{\lt}{<}\) \(\newcommand{\gt}{>}\) \(\newcommand{\amp}{&}\) \(\definecolor{fillinmathshade}{gray}{0.9}\)Except for their plastic lunch coolers, you might think that this picture of children on their way to school came from the 1800s. In fact, the picture is a photograph that was taken in 2006. The children are part of a religious community called the Amish, whose founders first came to the U.S. in the 1700s. Amish people shun modern conveniences such as electricity and automobiles. Their lives are more similar to the lives of their founders than to those of most other people in the U.S. today. As you will learn when you read this concept, the Amish are an example of one way in which populations may evolve.
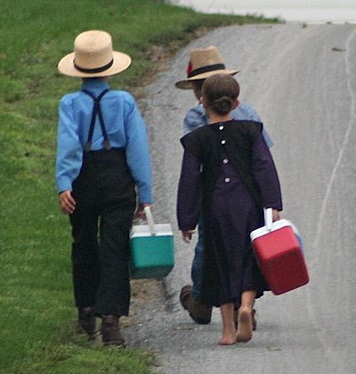
Genes in Populations
Individuals do not evolve because their genes do not change over time. Instead, evolution occurs at the level of the population. A population consists of organisms of the same species that live in the same area. In terms of evolution, the population is assumed to be a relatively closed group. This means that most mating takes place within the population. Evolutionary change that occurs over relatively short periods of time within populations is called microevolution. The science that focuses on evolution within populations is population genetics. It is a combination of evolutionary theory and Mendelian genetics.
The Gene Pool
The genetic makeup of an individual is the individual’s genotype. A population consists of many individuals and therefore many genotypes. All the genotypes together make up the population’s gene pool. The gene pool consists of all the genes of all the members of the population. For each gene, the gene pool includes all the different alleles of the gene that exist in the population. An allele is referred to as a version of a gene. For a given gene, the population is characterized by the frequency of the different alleles in the gene pool. Allele frequency is how often an allele occurs in a gene pool relative to the other alleles for the same gene.
Forces of Evolution
The factors that cause allele frequencies to change are called the forces of evolution. There are four such forces: mutation, gene flow, genetic drift, and natural selection.
Genetic Drift
Genetic drift is a random change in allele frequencies that occurs in a small population. When a small number of parents produce just a few offspring, allele frequencies in the offspring may differ, just by chance, from allele frequencies in the parents. This is like tossing a coin. If you toss a coin just a few times, you may, by chance, get more or less than the expected 50 percent heads and 50 percent tails. Due to such chance variations in small populations, allele frequencies drift over time.
There are two special conditions under which genetic drift occurs. They are called the bottleneck effect and founder effect.
- The bottleneck effect occurs when a population suddenly gets much smaller. This might happen because of a natural disaster such as a forest fire or disease epidemic. By chance, allele frequencies of the survivors may be different from those of the original population.
- The founder effect occurs when a few individuals start or found a new population. By chance, allele frequencies of the founders may be different from allele frequencies of the population they left. An example of the founder effect occurred in the Amish, as described in figure \(\PageIndex{2}\).
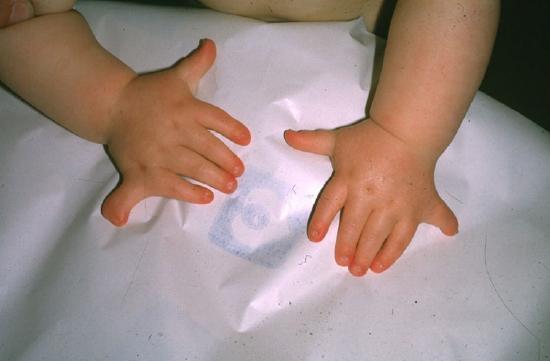
Mutation
Mutation creates new genetic variation in a gene pool. It is how all new alleles first arise. In sexually reproducing species, the mutations that matter for evolution are those that occur in gametes. Only these mutations can be passed to offspring. For any given gene, the chance of a mutation occurring in a given gamete is very low. Thus, mutations alone do not have much effect on allele frequencies. However, mutations provide the genetic variation needed for other forces of evolution to act.
Gene Flow
Gene flow occurs when individuals move into or out of a population. If the rate of migration is high, this can have a significant effect on allele frequencies. Allele frequencies may change in the population the migrants leave as well as in the population the migrants enter. An example of gene flow occurred during the Vietnam War in the 1960s and 1970s. Many young American servicemen had children with Vietnamese women. Most of the servicemen returned to the United States after the war. However, they left copies of their genes behind in their offspring. In this way, they changed the allele frequencies in the Vietnamese gene pool. Do you think the gene pool of the U.S. was also affected? Why or why not?
Natural Selection
Natural selection occurs when there are differences in fitness among members of a population. As a result, some individuals pass more genes to the next generation than do other members of the population. This causes allele frequencies to change over time. The example of sickle cell anemia, which is shown in the following table and described below, shows how natural selection can keep even a harmful allele in a gene pool.
Genotype | Phenotype | Fitness |
---|---|---|
AA | 100% normal hemoglobin | Somewhat reduced fitness because of no resistance to malaria |
AS | Enough normal hemoglobin to prevent sickle-cell anemia | Highest fitness because of resistance to malaria |
SS | 100% abnormal hemoglobin, causing sickle-cell anemia | Greatly reduced fitness because of sickle-cell anemia |
The allele (S) for sickle cell anemia is a harmful, autosomal recessive allele. It is caused by a mutation in the normal allele (A) for hemoglobin (the oxygen-carrying protein on red blood cells). Malaria is a deadly tropical disease that is common in many African populations. Heterozygotes (AS) with the sickle cell allele are resistant to malaria. Therefore, they are more likely to survive and reproduce. This keeps the S allele in the gene pool.
The sickle cell example shows that fitness depends on phenotypes and also on the environment. What do you think might happen if malaria were to be eliminated in an African population with a relatively high frequency of the S allele? How might the fitness of the different genotypes change? How might this affect the frequency of the S allele?
The sickle cell trait is controlled by a single gene. Natural selection for polygenic traits, which are controlled by multiple genes, is more complex, although it is less complicated if you consider just phenotypes for polygenic traits rather than genotypes. There are three major ways that natural selection can affect the distribution of phenotypes for a polygenic trait. The three ways are shown in the graphs in Figure \(\PageIndex{3}\).
- Disruptive selection occurs when phenotypes in the middle of the range are selected against. This results in two overlapping phenotypes, one at each end of the distribution. An example is a sexual dimorphism. This refers to differences between the phenotypes of males and females of the same species. In humans, for example, males and females have different average heights and body shapes.
- Stabilizing selection occurs when phenotypes at both extremes of the phenotypic distribution are selected against. This narrows the range of variation. An example is human birth weight. Babies that are very large or very small at birth are less likely to survive, and this keeps birth weight within a relatively narrow range.
- Directional selection occurs when one of two extreme phenotypes is selected for. This shifts the distribution toward that extreme. This is the type of natural selection that the Grants observed in the beak size of Galápagos finches. Larger beaks were selected for during drought, so beak size increased over time.
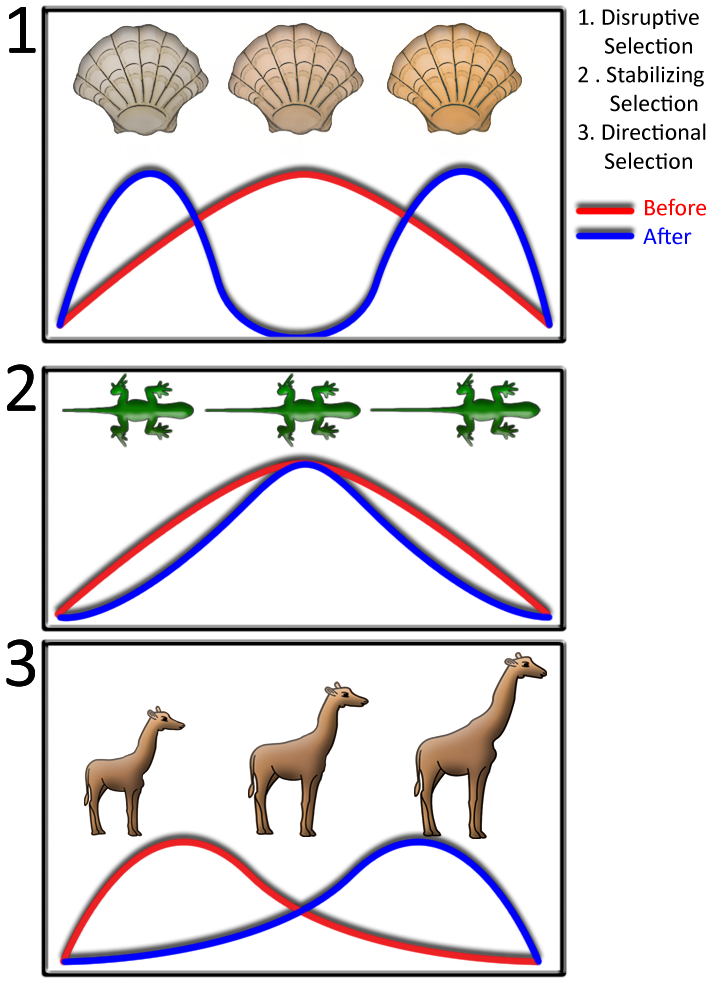
Recently reported research may help solve one of the most important and long-lasting mysteries of human biology. The mystery is why people with the AS genotype for sickle cell hemoglobin are protected from malaria. As you read above, their sickle cell hemoglobin gives them higher fitness in malaria areas than normal homozygotes (AA) who have only normal hemoglobin.
The malaria parasite and its mosquito vector were discovered in the late 1800s. The genetic basis of sickle cell hemoglobin anemia and the resistance to malaria it confers were discovered around 1950. Since then, scientists have assumed, and some evidence has suggested, that the few sickle-shaped red blood cells of heterozygotes make them less hospitable hosts for the malaria parasite than the completely normal red blood cells of AA homozygotes. This seems like a reasonable hypothesis, but is it the correct one? The new research suggests a different hypothesis.
Working with genetically engineered mice as model organisms, researchers in Portugal discovered that an enzyme that produces the gas carbon monoxide is expressed at much higher levels in the presence of sickle cell hemoglobin than normal hemoglobin. Furthermore, the gas seems to protect the infected host from developing the lesions and symptoms of malaria, even though it does not seem to interfere with the life cycle of the malaria parasite in red blood cells. These findings may lead to new therapies for treating malaria, which is still one of the most serious public health problems in the world. The findings may also shed light on other abnormal hemoglobin variants that are known to protect against malaria.
Review
- Why are populations, rather than individuals, the units of evolution?
- What is a gene pool?
- List and define the four forces of evolution.
- Why is mutation needed for evolution to occur, even though it usually has little effect on allele frequencies?
- What is the founder effect? Give an example.
- Identify three types of natural selection for polygenic traits.
- Explain why genetic drift is most likely to occur in a very small population.
- In some species, females prefer to mate with males that have certain genetically determined characteristics, such as bright coloration or a large, showy tail. How will this alter allele frequency in a population?
- Which of the following may cause genetic drift?
- A natural disaster
- A large population where members mate with each other and also with new migrants that come into the population.
- An island with no birds that becomes populated by a small number of a species of bird.
- Both A and C
- True or False. Allele frequencies can change within an organism.
- True or False. Most populations on Earth are in Hardy-Weinberg equilibrium.
- True or False. Genotype frequency can change if there is migration into or out of the population.
Explore More
Attributions
- Amish on their way to school by Gladjoboy, licensed CC BY 2.0 via Wikimedia Commons
- Polydactyly by Baujat G, Le Merrer M. CC BY 2.0 via Wikimedia Commons
- Selection type chart, by Azcolvin429, CC BY-SA 3.0; via Wikimedia.org
- Text adapted from Human Biology by CK-12 licensed CC BY-NC 3.0