7.4: Soils
- Page ID
- 31641
\( \newcommand{\vecs}[1]{\overset { \scriptstyle \rightharpoonup} {\mathbf{#1}} } \)
\( \newcommand{\vecd}[1]{\overset{-\!-\!\rightharpoonup}{\vphantom{a}\smash {#1}}} \)
\( \newcommand{\id}{\mathrm{id}}\) \( \newcommand{\Span}{\mathrm{span}}\)
( \newcommand{\kernel}{\mathrm{null}\,}\) \( \newcommand{\range}{\mathrm{range}\,}\)
\( \newcommand{\RealPart}{\mathrm{Re}}\) \( \newcommand{\ImaginaryPart}{\mathrm{Im}}\)
\( \newcommand{\Argument}{\mathrm{Arg}}\) \( \newcommand{\norm}[1]{\| #1 \|}\)
\( \newcommand{\inner}[2]{\langle #1, #2 \rangle}\)
\( \newcommand{\Span}{\mathrm{span}}\)
\( \newcommand{\id}{\mathrm{id}}\)
\( \newcommand{\Span}{\mathrm{span}}\)
\( \newcommand{\kernel}{\mathrm{null}\,}\)
\( \newcommand{\range}{\mathrm{range}\,}\)
\( \newcommand{\RealPart}{\mathrm{Re}}\)
\( \newcommand{\ImaginaryPart}{\mathrm{Im}}\)
\( \newcommand{\Argument}{\mathrm{Arg}}\)
\( \newcommand{\norm}[1]{\| #1 \|}\)
\( \newcommand{\inner}[2]{\langle #1, #2 \rangle}\)
\( \newcommand{\Span}{\mathrm{span}}\) \( \newcommand{\AA}{\unicode[.8,0]{x212B}}\)
\( \newcommand{\vectorA}[1]{\vec{#1}} % arrow\)
\( \newcommand{\vectorAt}[1]{\vec{\text{#1}}} % arrow\)
\( \newcommand{\vectorB}[1]{\overset { \scriptstyle \rightharpoonup} {\mathbf{#1}} } \)
\( \newcommand{\vectorC}[1]{\textbf{#1}} \)
\( \newcommand{\vectorD}[1]{\overrightarrow{#1}} \)
\( \newcommand{\vectorDt}[1]{\overrightarrow{\text{#1}}} \)
\( \newcommand{\vectE}[1]{\overset{-\!-\!\rightharpoonup}{\vphantom{a}\smash{\mathbf {#1}}}} \)
\( \newcommand{\vecs}[1]{\overset { \scriptstyle \rightharpoonup} {\mathbf{#1}} } \)
\( \newcommand{\vecd}[1]{\overset{-\!-\!\rightharpoonup}{\vphantom{a}\smash {#1}}} \)
\(\newcommand{\avec}{\mathbf a}\) \(\newcommand{\bvec}{\mathbf b}\) \(\newcommand{\cvec}{\mathbf c}\) \(\newcommand{\dvec}{\mathbf d}\) \(\newcommand{\dtil}{\widetilde{\mathbf d}}\) \(\newcommand{\evec}{\mathbf e}\) \(\newcommand{\fvec}{\mathbf f}\) \(\newcommand{\nvec}{\mathbf n}\) \(\newcommand{\pvec}{\mathbf p}\) \(\newcommand{\qvec}{\mathbf q}\) \(\newcommand{\svec}{\mathbf s}\) \(\newcommand{\tvec}{\mathbf t}\) \(\newcommand{\uvec}{\mathbf u}\) \(\newcommand{\vvec}{\mathbf v}\) \(\newcommand{\wvec}{\mathbf w}\) \(\newcommand{\xvec}{\mathbf x}\) \(\newcommand{\yvec}{\mathbf y}\) \(\newcommand{\zvec}{\mathbf z}\) \(\newcommand{\rvec}{\mathbf r}\) \(\newcommand{\mvec}{\mathbf m}\) \(\newcommand{\zerovec}{\mathbf 0}\) \(\newcommand{\onevec}{\mathbf 1}\) \(\newcommand{\real}{\mathbb R}\) \(\newcommand{\twovec}[2]{\left[\begin{array}{r}#1 \\ #2 \end{array}\right]}\) \(\newcommand{\ctwovec}[2]{\left[\begin{array}{c}#1 \\ #2 \end{array}\right]}\) \(\newcommand{\threevec}[3]{\left[\begin{array}{r}#1 \\ #2 \\ #3 \end{array}\right]}\) \(\newcommand{\cthreevec}[3]{\left[\begin{array}{c}#1 \\ #2 \\ #3 \end{array}\right]}\) \(\newcommand{\fourvec}[4]{\left[\begin{array}{r}#1 \\ #2 \\ #3 \\ #4 \end{array}\right]}\) \(\newcommand{\cfourvec}[4]{\left[\begin{array}{c}#1 \\ #2 \\ #3 \\ #4 \end{array}\right]}\) \(\newcommand{\fivevec}[5]{\left[\begin{array}{r}#1 \\ #2 \\ #3 \\ #4 \\ #5 \\ \end{array}\right]}\) \(\newcommand{\cfivevec}[5]{\left[\begin{array}{c}#1 \\ #2 \\ #3 \\ #4 \\ #5 \\ \end{array}\right]}\) \(\newcommand{\mattwo}[4]{\left[\begin{array}{rr}#1 \amp #2 \\ #3 \amp #4 \\ \end{array}\right]}\) \(\newcommand{\laspan}[1]{\text{Span}\{#1\}}\) \(\newcommand{\bcal}{\cal B}\) \(\newcommand{\ccal}{\cal C}\) \(\newcommand{\scal}{\cal S}\) \(\newcommand{\wcal}{\cal W}\) \(\newcommand{\ecal}{\cal E}\) \(\newcommand{\coords}[2]{\left\{#1\right\}_{#2}}\) \(\newcommand{\gray}[1]{\color{gray}{#1}}\) \(\newcommand{\lgray}[1]{\color{lightgray}{#1}}\) \(\newcommand{\rank}{\operatorname{rank}}\) \(\newcommand{\row}{\text{Row}}\) \(\newcommand{\col}{\text{Col}}\) \(\renewcommand{\row}{\text{Row}}\) \(\newcommand{\nul}{\text{Nul}}\) \(\newcommand{\var}{\text{Var}}\) \(\newcommand{\corr}{\text{corr}}\) \(\newcommand{\len}[1]{\left|#1\right|}\) \(\newcommand{\bbar}{\overline{\bvec}}\) \(\newcommand{\bhat}{\widehat{\bvec}}\) \(\newcommand{\bperp}{\bvec^\perp}\) \(\newcommand{\xhat}{\widehat{\xvec}}\) \(\newcommand{\vhat}{\widehat{\vvec}}\) \(\newcommand{\uhat}{\widehat{\uvec}}\) \(\newcommand{\what}{\widehat{\wvec}}\) \(\newcommand{\Sighat}{\widehat{\Sigma}}\) \(\newcommand{\lt}{<}\) \(\newcommand{\gt}{>}\) \(\newcommand{\amp}{&}\) \(\definecolor{fillinmathshade}{gray}{0.9}\)Soil is the outer loose layer that covers the surface of Earth. Soil quality is a major determinant, along with climate, of plant distribution and growth. Soil quality depends not only on the chemical composition of the soil, but also climate, topography, and organisms living in the soil. In agriculture, the history of the soil, such as the cultivating practices and previous crops, modify the characteristics and fertility of that soil.
Importance of Soil
Soil is important to our society primarily because it provides the foundation of agriculture and forestry. Soil plays a key role in plant growth. Beneficial aspects to plants include providing physical support, water, heat, nutrients, and oxygen. Mineral nutrients from the soil can dissolve in water and then become available to plants. Through their roots, plants absorb water and minerals (e.g., nitrates, phosphates, potassium, copper, zinc). With these and carbon dioxide acquired during photosynthesis, plants produce carbohydrates, proteins, lipids, nucleic acids, and the vitamins, on which consumers depend.
Soil plays a role in nearly all biogeochemical cycles on the Earth’s surface. Global cycling of key elements such as carbon (C), nitrogen (N), phosphorous (P), and sulfur (S) all pass through soil. In the hydrologic (water) cycle, soil helps to mediate infiltration (percolating) from the surface into the groundwater. Microorganisms living in soil can also be important components of biogeochemical cycles through the action of decomposition and other processes such as nitrogen fixation.
Several elements are considered essential for plant growth. Carbon (C), hydrogen (H), and oxygen (O) are required in large quantities, but they are not absorbed as mineral nutrients from the soil. Plants obtain carbon from carbon dioxide in the atmosphere and hydrogen from the water absorbed by the roots. Oxygen atoms come from carbon dioxide acquired during and gaseous oxygen in the atmosphere (acquire through aerobic cellular respiration) as well as water. Of the mineral nutrients absorbed from the soil, macronutrients, including nitrogen (N), potassium (K), calcium (Ca), magnesium (Mg), phosphorus (P), sulfur (S), and silicon (Si) are needed by plants in significant quantities. Micronutrients are essential elements that are needed only in small quantities, but can still be limiting to plant growth since these nutrients are not so abundant in nature. Micronutrients include chlorine (Cl), iron (Fe), boron (B), manganese (Mn), sodium (Na), zinc (Zn), copper (Cu), nickel (Ni), and molybdenum (Mo). There are some other elements that tend to aid plant growth but are not absolutely essential.
Although many aspects of soil are beneficial to plants, excessively high levels of trace metals (either naturally occurring or added by humans) or applied herbicides can be toxic to some plants (figure \(\PageIndex{a}\)).
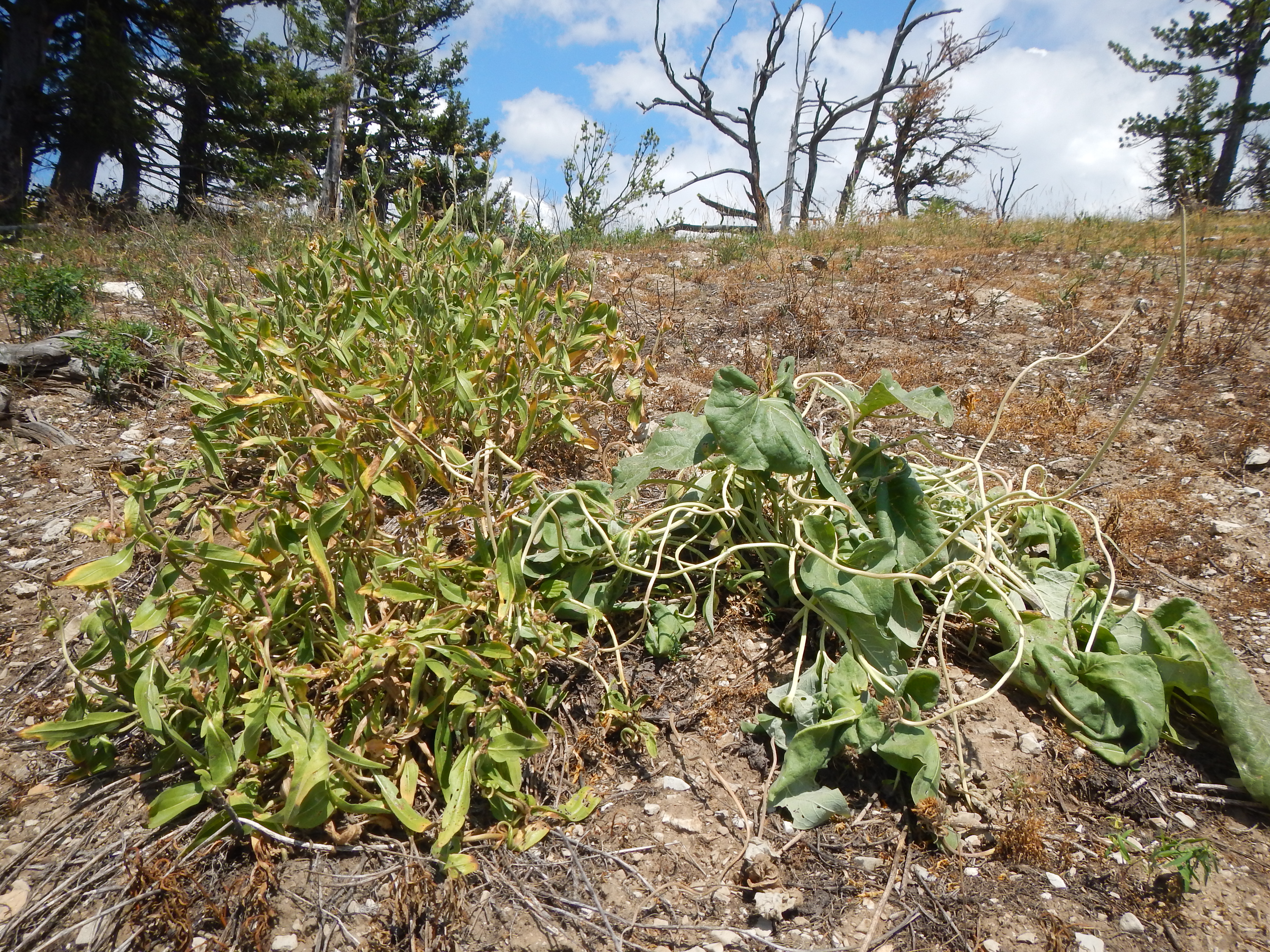
Micronutrients and macronutrients are desirable in particular concentrations and can be detrimental to plant growth when concentrations in soil solution are either too low (limiting) or too high (toxicity). Mineral nutrients are useful to plants only if they are in an extractable form in soil solutions, such as a dissolved ion rather than in solid mineral. Many nutrients move through the soil and into the root system as a result of concentration gradients, moving by diffusion from high to low concentrations. However, some nutrients are selectively absorbed by the root membranes, enabling concentrations to become higher inside the plant than in the soil.
An important factor affecting soil fertility is soil pH (the negative log of the hydrogen ion concentration). Soil pH is a measure of the acidity or alkalinity of the soil solution. On the pH scale (0 to 14) a value of seven represents a neutral solution; a value less than seven represents an acidic solution and a value greater than seven represents an alkaline solution (figure \(\PageIndex{b}\)). Soil pH affects the health of microorganisms in the soil and controls the availability of nutrients in the soil solution. Strongly acidic soils (less than 5.5) hinder the growth of bacteria that decompose organic matter in the soil. This results in a buildup of organic matter that has yet to be decomposed, which leaves important nutrients such as nitrogen in forms that are unusable by plants. Soil pH also affects the solubility of nutrient-bearing minerals. This is important because the nutrients must be dissolved in solution for plants to assimilate them through their roots. Most minerals are more soluble in slightly acidic soils than in neutral or slightly alkaline soils. Strongly acid soils (pH four to five), though, can result in high concentrations of aluminum, iron and manganese in the soil solution, which may inhibit the growth of some plants.
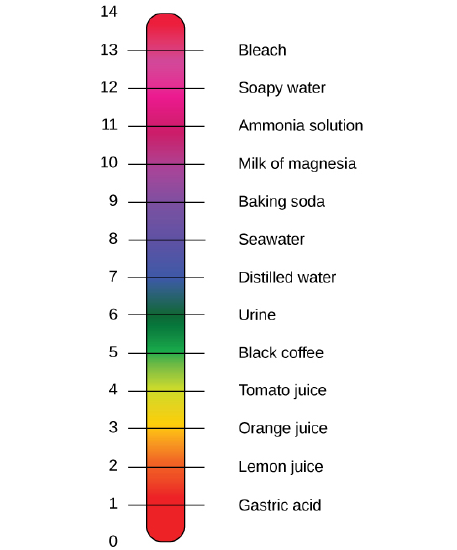
Several factors determine soil pH. Organic material in soil decreases pH to an extent, but it also acts as a buffer, limiting changes in pH. Climate is also important, with high amounts of rainfall increasing leaching and lowering pH. Some types of parent material, such as those high in silicon, decrease pH, while others, such as limestone increase pH.
Soil Composition
Soil consists of organic matter (about 5%), inorganic mineral matter (40-45% of soil volume), water (about 25%) and air (about 25%). The amount of each of the four major components of soil depends on the amount of vegetation, soil compaction, and water present in the soil.
The organic material consists of dead organisms in various stages of decomposition. It is dark-colored because it contains humus, partially decayed matter containing organic acids. Humus enriches the soil with nutrients, gives the soil a loose texture that holds water, and allows air to diffuse through it. Oxygen is important for plant roots and many inhabitants of the soil. The organic component of soil serves as a cementing agent, returns nutrients to the plant, allows soil to store moisture, makes soil tillable for farming, and provides energy for soil microorganisms. Most soil microorganisms—bacteria, algae, or fungi—are dormant in dry soil, but become active once moisture is available.
The inorganic material of soil consists of rock, slowly broken down into smaller particles that vary in size. Soil particles that are 100 μm to 2 mm in diameter are sand. (A micrometer, μm, 10-6 m, or a millionth of a meter.) Soil particles between 2 and 100 μm are called silt, and even smaller particles, less than 2 μm in diameter, are called clay.
Soil should ideally contain 50 percent solid material and 50 percent pore space (figure \(\PageIndex{c}\)). Pore space refers to the gaps in between soil particles. The larger the soil particles, the larger the pore spaces. Water can quickly pass through large pore spaces, so soils high in sand drain easily. Smaller soil particles have more surface area relative to volume and produce narrow pore spaces. Water clings to these surfaces, and soils high in clay thus retain water. (Clay is also negatively charged, which attracts water.) About one-half of the pore space should contain water, and the other half should contain air.
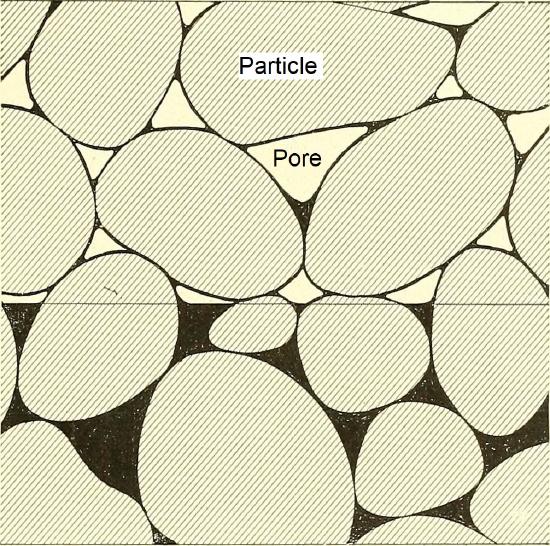
Soil texture is based on percentages of sand, silt, and clay (figure \(\PageIndex{d}\)). Soils that have a high percentage of one particle size are named after that particle (a clay soil has a high percentage of clay). Other soils have a mixture of two particle sizes and very little of the third size. For example, silty clay has roughly 50% clay and 50% silt while sandy clay has 50-60% sand and 35-50% clay. Some soils have no dominant particle size and contain a mixture of sand, silt, and humus. These soils are called loams, and they are optimal for agriculture. A medium loam has roughly 40% sand, 40% silt, and 20% clay. Larger particles (sand) facilitate drainage, and small particles (clay) facilitate water retention, so loam soils both have good drainage and can remain moist. Soils that deviate slightly from a medium loam include loamy sand, sandy loam, sandy clay loam, clay loam, silty clay loam, and silty loam.
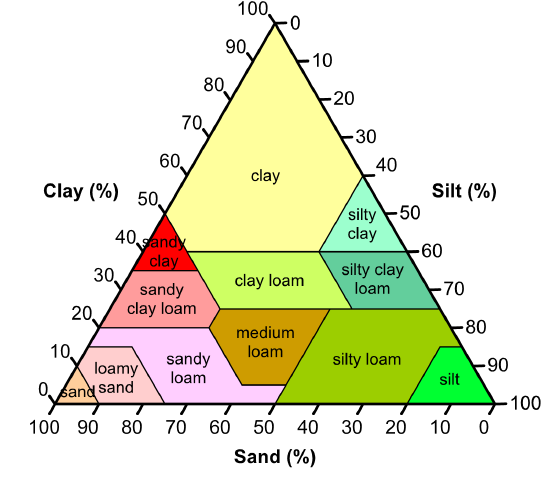
Organic Versus Mineral Soils
Soils can be divided into two groups based on how they form. Organic soils are those that are formed from sedimentation and often contain more than 30% organic matter. They form when organic matter, such as leaf litter, is deposited more quickly than it can be decomposed (figure \(\PageIndex{e}\)). Mineral soils are formed from the weathering of rocks, typically contain no more than 30% organic matter, and are primarily composed of inorganic material. Weathering occurs when biological, physical, and chemical processes, such as erosion, leaching, or high temperatures, break down rocks.
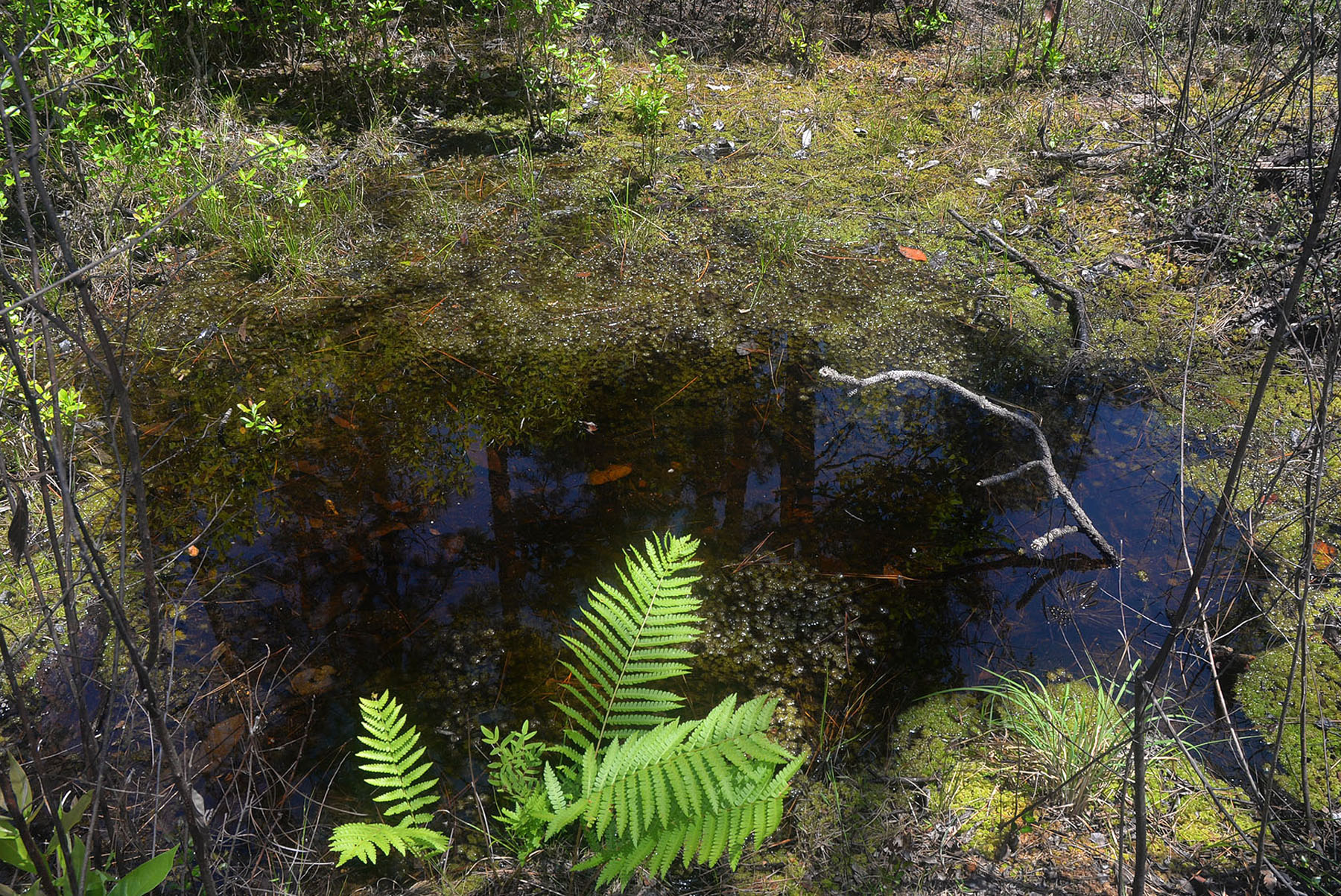
Soil Horizons
Soil distribution is not uniform because its formation results in the production of layers; together, the vertical section of a soil is called the soil profile. Within the soil profile, soil scientists define zones called horizons. A horizon is a soil layer with distinct physical and chemical properties that differ from those of other layers.
The soil profile has four distinct layers: 1) O horizon; 2) A horizon; 3) B horizon and 4) C horizon (figure \(\PageIndex{f}\)-g). Upper horizons (labeled as the A and O horizons) are richer in organic material and so are important in plant growth, while deeper layers (such as the B and C horizons) retain more of the original features of the bedrock below. Some soils may have additional layers (like the E horizon, figure \(\PageIndex{f}\)), or lack one of these layers. The thickness of the layers is also variable, and depends on the factors that influence soil formation. In general, immature soils may have O, A, and C horizons, whereas mature soils may display all of these, plus additional layers.
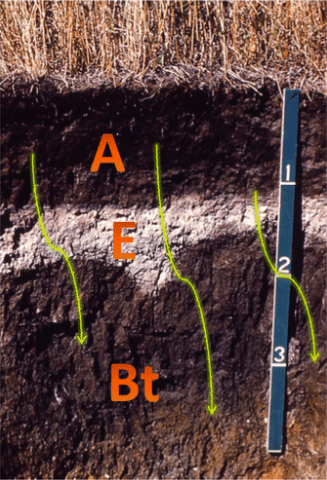
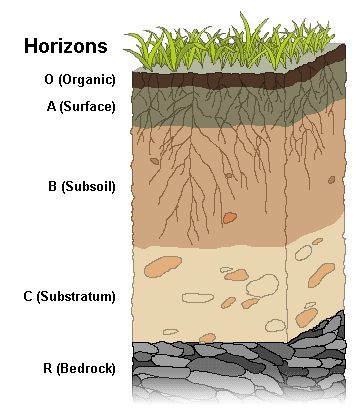
O horizon
The very top of the O horizon consists of partially decayed organic debris like leaves. This horizon is usually dark in color because of humus.
A horizon
The A horizon (topsoil) consists of a mixture of organic material with inorganic products of weathering, and it is therefore the beginning of true mineral soil. In this area, rainwater percolates through the soil and carries materials from the surface. The A horizon may be only 5 cm (2 in.), or it may over a meter. For instance, river deltas like the Mississippi River delta have deep layers of topsoil. Microbial processes occur in the top soil, and this horizon supports plant growth. Many organisms, such as earthworms and insects live among the plant roots in this horizon.
B horizon
The B horizon (subsoil) consists of small particles that have moved downward, resulting in a dense layer in the soil. In some soils, the B horizon contains nodules or a layer of calcium carbonate. The subsoil is usually lighter in color than topsoil and often contains an accumulation of minerals.
C horizon
The C horizon (soil base), includes the parent material, the organic and inorganic substances from which soils form. Weathering parent material represents the first steps in the chemical breakdown of rock into soil. Often the weathered parent material is underlain by the parent material itself, although in some places it has been carried from another location by wind, water, or glaciers. Beneath the C horizon lies bedrock. The chemical nature of the parent material, whether granite, limestone, or sandstone, for example, has a great influence on the fertility of the soil derived from it.
Factors Affecting Soil Formation and Composition
The fundamental factors that affect soil genesis can be categorized into five elements: climate, organisms, topography, parent material, and time. One could say that the relief, climate, and organisms dictate the local soil environment and act together to cause weathering and mixing of the soil parent material over time.
Climate
The role of climate in soil development includes aspects of temperature and precipitation. Soils in very cold areas with permafrost conditions (such as the arctic tundra) tend to be shallow and weakly developed due to the short growing season. In warm, tropical climates, soils tend to be thicker (but lacking in organic matter), with extensive leaching and mineral alteration. In such climates, organic matter decomposition and chemical weathering occur at an accelerated rate. The presence of moisture and nutrients from weathering will also promote biological activity: a key component of a quality soil. See the Biomes chapter for more details about the effect of climate on soils.
Ancient soils, sometimes buried and preserved in the subsurface, are referred to as paleosols (figure \(\PageIndex{h}\)) and reflect past climatic and environmental conditions.
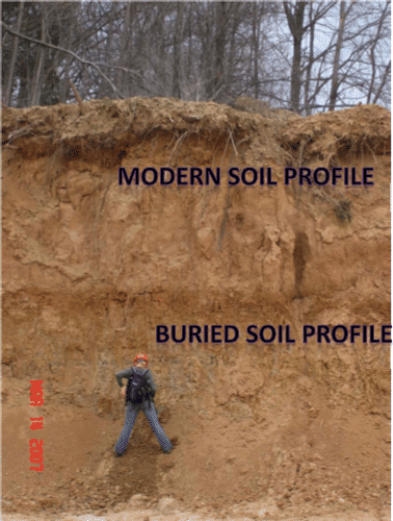
Organisms
The presence of living organisms in the soil (soil biota) greatly affects soil formation and structure. A diversity of animals are found in the soil such as nematodes, spiders, insects, centipedes, millipedes, pillbugs, slugs, and earthworms (figure \(\PageIndex{i}\)). The soil also contains microorganisms like bacteria, archaea, fungi, and "protists". Animals and microorganisms can produce pores and crevices, and plant roots can penetrate into crevices to produce more fragmentation. Additionally, leaves and other material that fall from plants decompose and contribute to soil composition. Microorganisms not only decompose organic matter, but contribute to other processes in nutrient cycles, such as nitrogen fixation.
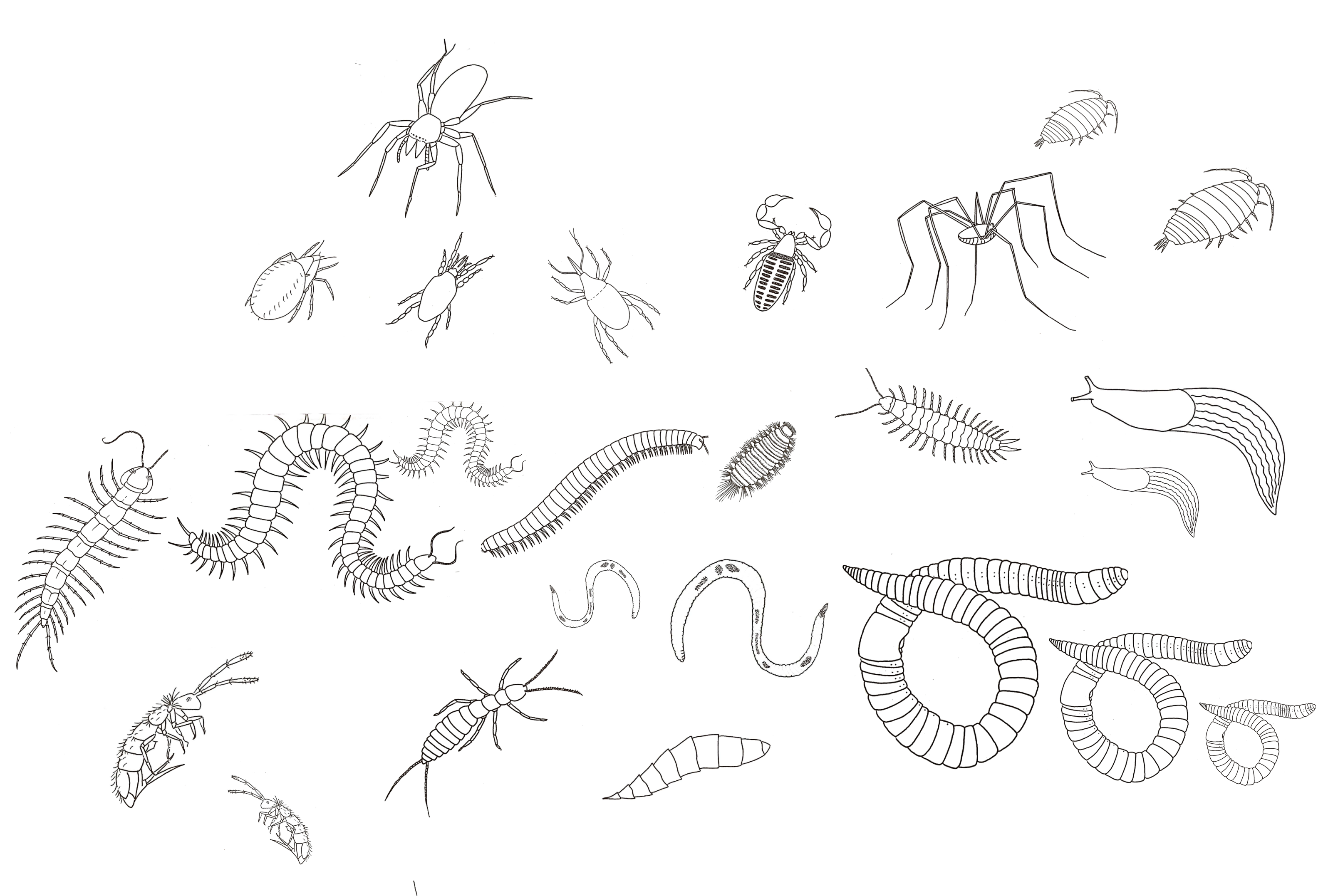
Parent Material
Mineral soils form directly from the weathering of bedrock, the solid rock that lies beneath the soil, and therefore, they have a similar composition to the original rock. Other soils form in materials that came from elsewhere, such as sand and glacial drift. Materials located in the depth of the soil are relatively unchanged compared with the deposited material. Sediments in rivers may have different characteristics, depending on whether the stream moves quickly or slowly. A fast-moving river could have sediments of rocks and sand, whereas a slow-moving river could have fine-textured material, such as clay.
The type of parent material may also affect the rapidity of soil development. Parent materials that are highly weatherable (such as volcanic ash) will transform more quickly into highly developed soils, whereas parent materials that are quartz-rich, for example, will take longer to develop. Parent materials also provide nutrients to plants and can affect soil internal drainage.
Topography
Regional surface features (familiarly called “the lay of the land”) can have a major influence on the characteristics and fertility of a soil. Topography affects water runoff, which strips away parent material and affects plant growth. Soils on steep slopes are more prone to erosion and may be thinner than soils that are on relatively level ground. Infiltration, the percolating of water through the soil, is limited in steep soils.
The local topography can have important microclimatic effects. In the northern hemisphere, south-facing slopes are exposed to more direct sunlight angles and are thus warmer and drier than north-facing slopes. The cooler, moister north-facing slopes have a more dynamic plant community and thicker soils because extensive root systems stabilize the soil and reduce erosion (figure \(\PageIndex{j}\)).
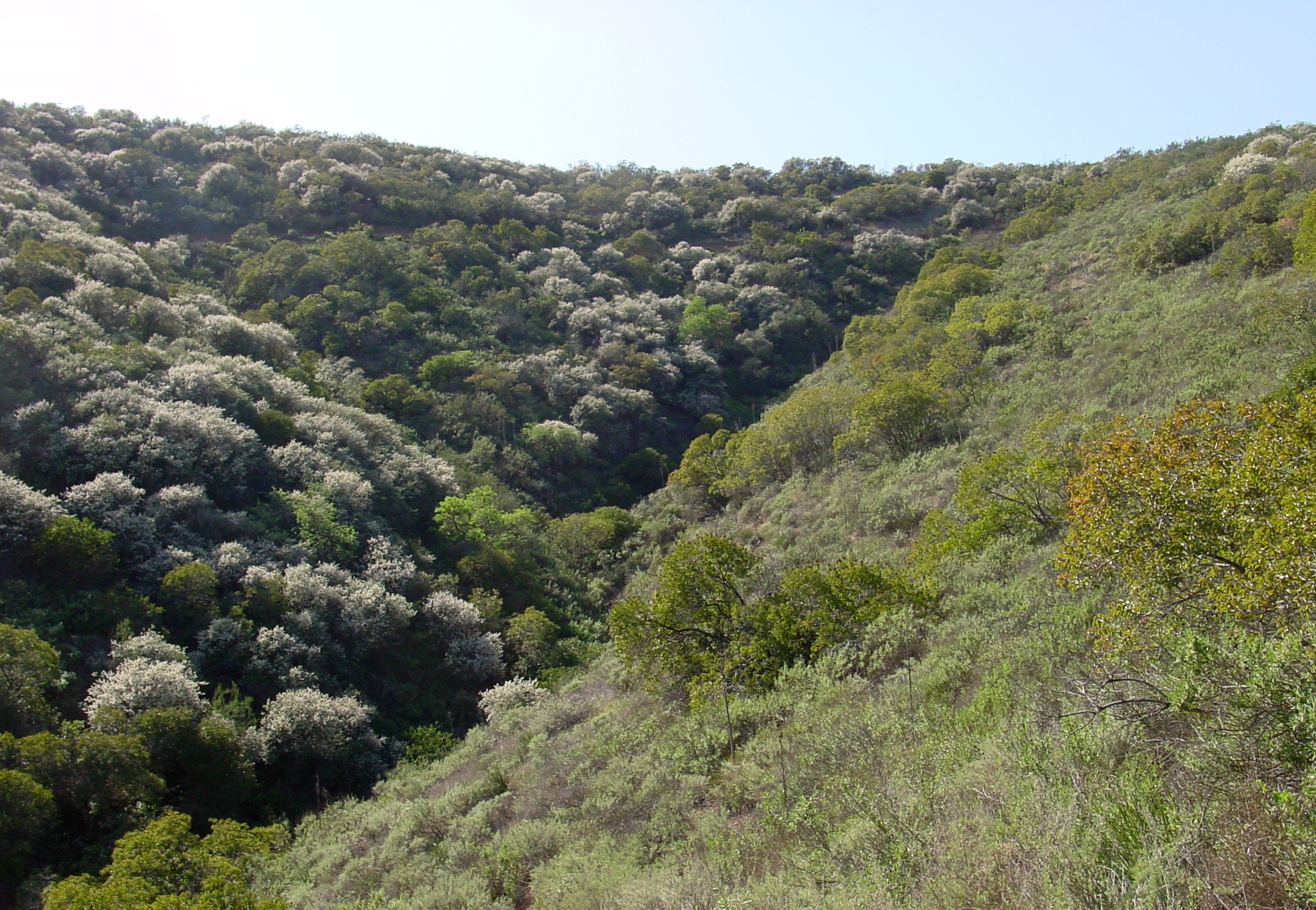
Time
Time is an important factor in soil formation because soils develop over long periods. Soil formation is a dynamic process. Materials are deposited over time, decompose, and transform into other materials that can be used by living organisms or deposited onto the surface of the soil.
In general, soil profiles tend to become thicker (deeper), more developed, and more altered over time. However, the rate of change is greater for soils in youthful stages of development. The degree of soil alteration and deepening slows with time and at some point, after tens or hundreds of thousands of years, may approach an equilibrium condition where erosion and deepening (removals and additions) become balanced. Young soils (< 10,000 years old) are strongly influenced by parent material and typically develop horizons and character rapidly. Over time, as weathering processes deepen, mix, and alter the soil, the parent material becomes less recognizable as chemical, physical, and biological processes take effect. Moderate age soils (roughly 10,000 to 500,000 years old) are slowing in profile development and deepening, and may begin to approach equilibrium conditions. Old soils (>500,000 years old) have generally reached their limit as far as soil horizonation and physical structure, but may continue to alter chemically or mineralogically.
Soil development is not always continual. Geologic events such as landslides, glacier advance, or the rising of shorelines can rapidly bury soils. Erosion in rivers and shorelines can cause removal or truncation of soils, and wind or flooding slowly deposit sediment that adds to the soil. Animals can mix the soil and sometimes cause soil regression, a reversal or "bump in the road" for the normal path of development, and this increases development over time.
Soil Taxonomy
Soils are classified into one of 12 soil orders based on soil horizons, how they form, and their chemical compositions. For example, Mollisols (figure \(\PageIndex{f}\)), which are found in temperate grasslands, have a thick topsoil rich in organic content. Aridisols, on the other hand, are dry soils that contain calcium carbonate and are found in deserts. Each soil order is further divided into suborders. See USDA's The Twelve Orders of Soil Taxonomy and The Twelve Soil Orders from the University of Idaho for more details.
Attributions
Modified by Melissa Ha from the following sources:
- The Soil from General Biology by OpenStax (CC-BY)
- Soil Profiles and Processes and Soil-Plant Interactions from Environmental Biology by Matthew R. Fisher (licensed under CC-BY)
- Soil from Biology by John W. Kimball (licensed under CC-BY)