28.11: Signaling by Steroid Hormones
- Page ID
- 15119
\( \newcommand{\vecs}[1]{\overset { \scriptstyle \rightharpoonup} {\mathbf{#1}} } \)
\( \newcommand{\vecd}[1]{\overset{-\!-\!\rightharpoonup}{\vphantom{a}\smash {#1}}} \)
\( \newcommand{\id}{\mathrm{id}}\) \( \newcommand{\Span}{\mathrm{span}}\)
( \newcommand{\kernel}{\mathrm{null}\,}\) \( \newcommand{\range}{\mathrm{range}\,}\)
\( \newcommand{\RealPart}{\mathrm{Re}}\) \( \newcommand{\ImaginaryPart}{\mathrm{Im}}\)
\( \newcommand{\Argument}{\mathrm{Arg}}\) \( \newcommand{\norm}[1]{\| #1 \|}\)
\( \newcommand{\inner}[2]{\langle #1, #2 \rangle}\)
\( \newcommand{\Span}{\mathrm{span}}\)
\( \newcommand{\id}{\mathrm{id}}\)
\( \newcommand{\Span}{\mathrm{span}}\)
\( \newcommand{\kernel}{\mathrm{null}\,}\)
\( \newcommand{\range}{\mathrm{range}\,}\)
\( \newcommand{\RealPart}{\mathrm{Re}}\)
\( \newcommand{\ImaginaryPart}{\mathrm{Im}}\)
\( \newcommand{\Argument}{\mathrm{Arg}}\)
\( \newcommand{\norm}[1]{\| #1 \|}\)
\( \newcommand{\inner}[2]{\langle #1, #2 \rangle}\)
\( \newcommand{\Span}{\mathrm{span}}\) \( \newcommand{\AA}{\unicode[.8,0]{x212B}}\)
\( \newcommand{\vectorA}[1]{\vec{#1}} % arrow\)
\( \newcommand{\vectorAt}[1]{\vec{\text{#1}}} % arrow\)
\( \newcommand{\vectorB}[1]{\overset { \scriptstyle \rightharpoonup} {\mathbf{#1}} } \)
\( \newcommand{\vectorC}[1]{\textbf{#1}} \)
\( \newcommand{\vectorD}[1]{\overrightarrow{#1}} \)
\( \newcommand{\vectorDt}[1]{\overrightarrow{\text{#1}}} \)
\( \newcommand{\vectE}[1]{\overset{-\!-\!\rightharpoonup}{\vphantom{a}\smash{\mathbf {#1}}}} \)
\( \newcommand{\vecs}[1]{\overset { \scriptstyle \rightharpoonup} {\mathbf{#1}} } \)
\( \newcommand{\vecd}[1]{\overset{-\!-\!\rightharpoonup}{\vphantom{a}\smash {#1}}} \)
\(\newcommand{\avec}{\mathbf a}\) \(\newcommand{\bvec}{\mathbf b}\) \(\newcommand{\cvec}{\mathbf c}\) \(\newcommand{\dvec}{\mathbf d}\) \(\newcommand{\dtil}{\widetilde{\mathbf d}}\) \(\newcommand{\evec}{\mathbf e}\) \(\newcommand{\fvec}{\mathbf f}\) \(\newcommand{\nvec}{\mathbf n}\) \(\newcommand{\pvec}{\mathbf p}\) \(\newcommand{\qvec}{\mathbf q}\) \(\newcommand{\svec}{\mathbf s}\) \(\newcommand{\tvec}{\mathbf t}\) \(\newcommand{\uvec}{\mathbf u}\) \(\newcommand{\vvec}{\mathbf v}\) \(\newcommand{\wvec}{\mathbf w}\) \(\newcommand{\xvec}{\mathbf x}\) \(\newcommand{\yvec}{\mathbf y}\) \(\newcommand{\zvec}{\mathbf z}\) \(\newcommand{\rvec}{\mathbf r}\) \(\newcommand{\mvec}{\mathbf m}\) \(\newcommand{\zerovec}{\mathbf 0}\) \(\newcommand{\onevec}{\mathbf 1}\) \(\newcommand{\real}{\mathbb R}\) \(\newcommand{\twovec}[2]{\left[\begin{array}{r}#1 \\ #2 \end{array}\right]}\) \(\newcommand{\ctwovec}[2]{\left[\begin{array}{c}#1 \\ #2 \end{array}\right]}\) \(\newcommand{\threevec}[3]{\left[\begin{array}{r}#1 \\ #2 \\ #3 \end{array}\right]}\) \(\newcommand{\cthreevec}[3]{\left[\begin{array}{c}#1 \\ #2 \\ #3 \end{array}\right]}\) \(\newcommand{\fourvec}[4]{\left[\begin{array}{r}#1 \\ #2 \\ #3 \\ #4 \end{array}\right]}\) \(\newcommand{\cfourvec}[4]{\left[\begin{array}{c}#1 \\ #2 \\ #3 \\ #4 \end{array}\right]}\) \(\newcommand{\fivevec}[5]{\left[\begin{array}{r}#1 \\ #2 \\ #3 \\ #4 \\ #5 \\ \end{array}\right]}\) \(\newcommand{\cfivevec}[5]{\left[\begin{array}{c}#1 \\ #2 \\ #3 \\ #4 \\ #5 \\ \end{array}\right]}\) \(\newcommand{\mattwo}[4]{\left[\begin{array}{rr}#1 \amp #2 \\ #3 \amp #4 \\ \end{array}\right]}\) \(\newcommand{\laspan}[1]{\text{Span}\{#1\}}\) \(\newcommand{\bcal}{\cal B}\) \(\newcommand{\ccal}{\cal C}\) \(\newcommand{\scal}{\cal S}\) \(\newcommand{\wcal}{\cal W}\) \(\newcommand{\ecal}{\cal E}\) \(\newcommand{\coords}[2]{\left\{#1\right\}_{#2}}\) \(\newcommand{\gray}[1]{\color{gray}{#1}}\) \(\newcommand{\lgray}[1]{\color{lightgray}{#1}}\) \(\newcommand{\rank}{\operatorname{rank}}\) \(\newcommand{\row}{\text{Row}}\) \(\newcommand{\col}{\text{Col}}\) \(\renewcommand{\row}{\text{Row}}\) \(\newcommand{\nul}{\text{Nul}}\) \(\newcommand{\var}{\text{Var}}\) \(\newcommand{\corr}{\text{corr}}\) \(\newcommand{\len}[1]{\left|#1\right|}\) \(\newcommand{\bbar}{\overline{\bvec}}\) \(\newcommand{\bhat}{\widehat{\bvec}}\) \(\newcommand{\bperp}{\bvec^\perp}\) \(\newcommand{\xhat}{\widehat{\xvec}}\) \(\newcommand{\vhat}{\widehat{\vvec}}\) \(\newcommand{\uhat}{\widehat{\uvec}}\) \(\newcommand{\what}{\widehat{\wvec}}\) \(\newcommand{\Sighat}{\widehat{\Sigma}}\) \(\newcommand{\lt}{<}\) \(\newcommand{\gt}{>}\) \(\newcommand{\amp}{&}\) \(\definecolor{fillinmathshade}{gray}{0.9}\)Introduction
We will now consider signaling by steroid hormones. These hormones are derivatives of cholesterol, which is found in membrane bilayers and lipoproteins. They are mostly nonpolar. Steroid hormones can affect signaling in two major ways:
- through binding to membrane receptors, which when occupied affect signaling through the myriad of ways we discussed throughout this chapter. These effects would be rapid.
- through binding to cytoplasmic receptors after they diffuse into the cell through passive and active means. This signaling is hence similar to retrograde signaling by nitric oxide, which can passively diffuse out of a cell and enter an adjacent cell to effect signaling in that cell. If the steroid primary messenger is in the cell, it most often can enter the nucleus and regulate gene transcription. Binding to cytoplasmic receptors account for most of the biological effects of steroid hormones. Since transcription is involved, the pathways elicit a slower response.
We will briefly discuss the first type of signaling (binding to membrane receptors) mostly by presenting figures which describe their signaling pathways. Then, we will focus on steroid hormone activation of gene transcription.
There are many classes of steroid hormones. These are illustrated in Figure \(\PageIndex{1}\) along with their overall synthetic pathway.
Figure \(\PageIndex{1}\): Diagram of the pathways of human steroidogenesis. WikiJournal of Medicine 1 (1). DOI:10.15347/wjm/2014.005. ISSN 20018762.
The overall families include progestogens and estrogens (female sex hormones), androgens (male sex hormones), glucocorticoids (like cortisol which affects immune and metabolic systems), and mineral corticoids (which affect salt/water balance).
We will mostly use the estrogens in the section as a prototypical example because of their involvement in breast cancer and epidermal growth factor receptors.
Steroid hormone signaling through binding to membrane receptors
Most of this subsection is taken from and adapted from the following source: Masi et al. Cells 2021, 10(11), 2999; https://doi.org/10.3390/cells10112999. Creative Commons Attribution (CC BY) license (https://creativecommons.org/licenses/by/4.0/).
Most people think of steroid signaling, it is through their nuclear effects on gene transcription (the predominant signaling effect). Signaling at the cell membrane is an emerging area of interest. We will present steroid signaling at the cell nucleus in four figures with their associated captions. We present these lesser-known effects of steroid signaling first since they utilize many of the pathways we have already studied. The figures will also give you a short review of some canonical pathways, which is always good in a field so complicated as signal transduction. The figures focus on the signaling effects in cancer.
Figure \(\PageIndex{2}\) shows membrane signaling through androgen receptors.
Figure \(\PageIndex{3}\) shows membrane signaling through estrogen receptors
A tamoxifen (a selective estrogen receptor modulator used in breast cancer therapy) binding site has been found in NavMs voltage-gated sodium channels.
Figure \(\PageIndex{4}\) show membrane signaling through membrane progesterone receptors
Figure \(\PageIndex{5}\) shows membrane-associated progesterone receptor effects from progesterone signaling.
Steroid hormone signaling through binding to cytoplasmic receptors and activation of gene transcription
Now we will explore the major effects of steroid hormones on signaling, in which steroids enter the cell, bind to cytoplasmic receptors, and then translocate into the nucleus where they regulate gene expression. The naming of steroid receptors can be confusing since it is important to differentiate steroid receptors that are resident in the cell membrane and those that move to the nucleus. An added complexity arises from the factor that some nuclear receptors can be covalently modified with a fatty acid (palmitoylated) and targeted to the cell membrane. Estrogen receptors targeted to the membrane can then act independently of their nuclear transcriptional activity.
We will primarily focus on estrogen effects in breast cancer (most diagnosed cancer) that are mediated through the nuclear steroid receptors, which belong to the nuclear receptor superfamily. Tamoxifen, a commonly used drug in the treatment of breast cancer is an estrogen receptor antagonist (also called an estrogen receptor modulator—SERM).
Molecular Function of Steroid Receptors—Common Features
Steroid receptors (SR) consist of four main domains, the C-terminal ligand-binding domain (LBD), the DNA-binding domain (DBD), the hinge region, and amino-terminal domain (NTD). Each SR contains also two motifs called activation function 1 and 2 (AF1 and AF2) within NTD and LBD, respectively, and are crucial for the regulation of gene transcription. Two zinc fingers are located in the DBD. Figure \(\PageIndex{6}\):
The domains are often labeled A-F. The N-terminal domain (NTD) is also called the A/B domain. It can also bind DNA and can weakly activate transcription in the absence of hormones. The C domain is the DNA binding domain (DBD) containing Zn fingers, which bind to the steroid response element in promoters of key genes. The D domain is the hinge domain, and The E domain binds hormones (like estrogen) and protein regulators and when bound can activate gene transcription. The last domain (F) varies in length and its function is not completely clear.
The specific DNA structure for the estrogen receptor from Pfam is shown in Figure \(\PageIndex{7}\).

The green is the N-terminal Oest_recep domain (NTD, A/B). The red zf-C4 is the DNA binding domain which has two Zn fingers that bind DNA (DBD, C). The blue Hormone_Receptor is the ligand (estrogen) binding domain (LBD, domain E). The yellow is the C-terminal domain (F).
These two genes for the estrogen receptor encoding ERα and ERβ. The transcriptionally active form is a dimer that forms on the binding of estrogens. The dimer then translocates to the nucleus and activates transcription at ERE sites. The ERα dimer promotes estrogen-dependent growth while the ERβ dimer inhibits it. Heterodimers can form which seem to reduce the proliferative effects of ERα. Both ERα and ERβ can be expressed in
Figure \(\PageIndex{8}\) shows an interactive iCn3D model of the human estrogen receptor computational model (P03372, AlphaFold)
.png?revision=1&size=bestfit&width=327&height=343)
- red spacefill: N-terminal Met
- green backbone trace: N-terminal domain disordered, which can bind DNA
- magenta backbone trace: DNA binding domain with Zn fingers. The Cys side chains of the Zn fingers are shown in sticks, CPK colors, and labeled.
- blue: Hormone_Receptor is the ligand (estrogen) binding domain (LBD, domain E)
- yellow: C-terminal tail (domain)
- Cyan spacefill: C terminal Val
There are no full-length crystal structures of ER dimer. Most available structures are for the estrogen-binding domain. It's really useful to see the full predicted structure to see how all the domains are connected, but perhaps more interestingly, the extended regions of disordered structures, which you should imagine adopting specific structures on interaction with key signaling partners.
Figure \(\PageIndex{9}\) shows an interactive iCn3D model of the ligand binding domain of human estrogen receptor ERα bound to the antagonist tamoxifen (3ERT).
V2.png?revision=1&size=bestfit&width=395&height=264)
The ligand binding domains (one for each of the ERα monomers) are shown in magenta and cyan. The antagonist Tamoxifen, one bound in each of the ligand binding domains, is shown in sticks, CPK color. The amino acids comprising the binding site for tamoxifen, are shown in stick, and CPK colors and labeled in the magenta subunit. The CPK-colored spheres show the binding site on the ligand binding domain for other binding proteins called corepressors or coactivators (not shown, discussed below).
Figure \(\PageIndex{10}\) shows the structures of estrogens and selective estrogen receptor modulators (SERMs).
The iCn3D model for the human estrogen receptor ERα bound to the antagonist tamoxifen (3ERT) showed binding sites for other proteins called coactivators or corepressors. The ER-estrogen complex, after binding to DNA, can also bind a protein coactivator, which activates transcription. Likewise binding of a corepressor to the DNA-bound complex inhibits transcriptional activity. Tamoxifen binding to the ERα monomer leads to dimerization and DNA binding. The DNA-bound dimer can then bind either a corepressor (the usual case for tamoxifen binding to ER in the breast), leading to inhibition of DNA transcription (i.e. tamoxifen antagonizes ER transcriptional function), or a coactivator which stimulates gene transcription.
A cartoon diagram illustrating the role of ER coactivators and corepressors is shown in Figure \(\PageIndex{11}\).
When a SERM binds to the estrogen receptor, the receptor adopts a unique conformation that allows dimerization and interaction with estrogen response elements (EREs) of the target genes. The unique conformational change induced by the binding of the SERM may result in a distinct pattern of cofactor recruitment.
Before steroid binding, most steroid receptors are found in the cytoplasm bound to heat shock proteins like Hsp90. Phosphorylation of the Hsp:SR complex leads to dissociation of the Hsp, followed by dimerization and translocation into the nucleus. In some cases, hormone binding occurs in the nucleus.
Figure \(\PageIndex{12}\) shows an interactive iCn3D model of the estrogen receptor DNA-binding domain bound to DNA (1HCQ).
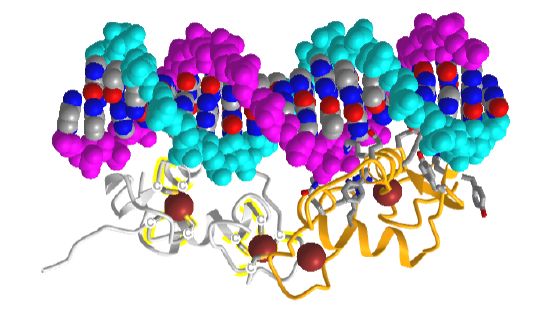
The backbones of the dsDNA are shown in spacefill cyan and magenta. The DNA bases are shown in CPK colors. The two chains of the DNA binding domain of the estrogen receptor are shown in gray and gold. Zn2+ ions are shown as brown spheres. The coordinating Cys side chains in the gray DNA binding domain are shown in sticks, CPK colors, and labeled "C". The amino acid side chains from the gold DNA binding domain that interact with DNA are shown in sticks CPK colors.
There are two types of ways that steroid hormones activate gene transcription, a direct and an indirect method
Direct (classical): The DNA binding domain (containing the Zn fingers binds) of the dimer bind to the target hormone response element (HRE) or for steroids the steroid response element (SRE) sequences in the promoter site of specific genes under steroid hormone control. As seen in the iCn3D above, one of the two Zn fingers on each of the hormone receptors binds to the target site in the major groove of DNA. The other Zn fingers are involved in the dimerization of the hormone receptor. The SRE contains two 6-base pair repeats separated by 3 base pairs. The DNA sequence shown in the iCn3D above is CCAPGGTCA. The general consensus sequence for steroid hormones is 5′-GGTACAnnnTGTTCT-3′. The ER binds to 5′-GGTCAnnnTGACC-3′. Note that the complementary strand sequence is 5'-GGTCAnnTGACC so the sequence is a palindrome (the complementary strand has the same sequence going in the opposite direction. After binding the hormone:receptor dimer to DNA, coregulators bind. These modify histones and remodel the DNA to facilitate or inhibit transcription.
Indirect: In this method, the steroid receptors bridge other DNA-bound transcription factors without the steroid hormone binding to its response element.
The direct and indirect methods for steroid hormone effects on transcription are shown in Figure \(\PageIndex{13}\).
(1) Translation of a SR and binding of Hsp70. (2) Hsp70 to Hsp90 transition. (3) Ligand binding, Hsp90 dissociation, and dimerization. (4) Nuclear translocation. (5) Transcriptional action: induction (5a, 5c) or inhibition (5b, 5d) of target gene expression, performed either in the classical mechanism involving SRE-binding (5a, 5b) or by tethering other TFs (5c, 5d). (6) Ligand dissociation followed by disassembly of the transcriptional complex and SR binding to a molecular chaperone. (7) Rebinding of the ligand. (8) Ubiquitination. (9) Proteasomal degradation. SR—steroid receptor, SH—steroid hormone, Hsp 70—heat shock protein 70, Hsp90—heat shock protein 90, SRE—steroid response element, CoA—coactivators, CoR—corepressors, HAT—histone acetyltransferase, HDAC—histone deacetylase, TF—transcription factor, TFRE—transcription factor response element, Ub—ubiquitin. Although histone acetyltransferases (HATs) and histone deacetylases (HDACs) are classified as coregulators, here they are shown separately to emphasize their role. Illustration created using elements from Servier Medical Art https://smart.servier.com/, reproduced under Creative Commons Attribution 3.0 Unported License https://creativecommons.org/licenses/by/3.0/.
Hormone dissociation leads to steroid hormone dissociation from DNA by chaperone proteins. The Hsp90-SR complex can reenter the cycle. The steroid hormone receptor can be targeted by proteolysis by the proteasome by ubiquitination in either the nucleus or the cytoplasm.
Modulation of ER function by phosphorylation
No pathways stand in isolation, so it should be no surprise the estrogen receptor (again used as an example) is regulated by post-translational modification, especially by phosphorylation. Phosphorylation can be ligand dependent or independent. Multiple kinases are involved in the phosphorylation of the N-terminal region. An especially important one occurs at Ser 118 at a cyclin-dependent kinase (involved in cell cycling) when the receptor is bound to estradiol. Ser 118 also gets phosphorylation through epidermal growth factor signaling by MAPK. This may increase cell proliferation in breast cancers even in the absence of estrogen. Figure \(\PageIndex{14}\) shows the activation of ER by phosphorylation through growth factor and cytokine signaling pathways.
Figure \(\PageIndex{14}\): Activation of ER by phosphorylation induced by growth factor and cytokine signaling pathways. Siersbæk et al ,Genes Dev. 2018 Sep 1; 32(17-18): 1141–1154. doi:10.1101/gad.316646.118. Creative Commons License (Attribution-NonCommercial 4.0 International), as described at http://creativecommons.org/licenses/by-nc/4.0/.