4.2: Linkage and Recombination
- Page ID
- 142554
\( \newcommand{\vecs}[1]{\overset { \scriptstyle \rightharpoonup} {\mathbf{#1}} } \)
\( \newcommand{\vecd}[1]{\overset{-\!-\!\rightharpoonup}{\vphantom{a}\smash {#1}}} \)
\( \newcommand{\id}{\mathrm{id}}\) \( \newcommand{\Span}{\mathrm{span}}\)
( \newcommand{\kernel}{\mathrm{null}\,}\) \( \newcommand{\range}{\mathrm{range}\,}\)
\( \newcommand{\RealPart}{\mathrm{Re}}\) \( \newcommand{\ImaginaryPart}{\mathrm{Im}}\)
\( \newcommand{\Argument}{\mathrm{Arg}}\) \( \newcommand{\norm}[1]{\| #1 \|}\)
\( \newcommand{\inner}[2]{\langle #1, #2 \rangle}\)
\( \newcommand{\Span}{\mathrm{span}}\)
\( \newcommand{\id}{\mathrm{id}}\)
\( \newcommand{\Span}{\mathrm{span}}\)
\( \newcommand{\kernel}{\mathrm{null}\,}\)
\( \newcommand{\range}{\mathrm{range}\,}\)
\( \newcommand{\RealPart}{\mathrm{Re}}\)
\( \newcommand{\ImaginaryPart}{\mathrm{Im}}\)
\( \newcommand{\Argument}{\mathrm{Arg}}\)
\( \newcommand{\norm}[1]{\| #1 \|}\)
\( \newcommand{\inner}[2]{\langle #1, #2 \rangle}\)
\( \newcommand{\Span}{\mathrm{span}}\) \( \newcommand{\AA}{\unicode[.8,0]{x212B}}\)
\( \newcommand{\vectorA}[1]{\vec{#1}} % arrow\)
\( \newcommand{\vectorAt}[1]{\vec{\text{#1}}} % arrow\)
\( \newcommand{\vectorB}[1]{\overset { \scriptstyle \rightharpoonup} {\mathbf{#1}} } \)
\( \newcommand{\vectorC}[1]{\textbf{#1}} \)
\( \newcommand{\vectorD}[1]{\overrightarrow{#1}} \)
\( \newcommand{\vectorDt}[1]{\overrightarrow{\text{#1}}} \)
\( \newcommand{\vectE}[1]{\overset{-\!-\!\rightharpoonup}{\vphantom{a}\smash{\mathbf {#1}}}} \)
\( \newcommand{\vecs}[1]{\overset { \scriptstyle \rightharpoonup} {\mathbf{#1}} } \)
\( \newcommand{\vecd}[1]{\overset{-\!-\!\rightharpoonup}{\vphantom{a}\smash {#1}}} \)
\(\newcommand{\avec}{\mathbf a}\) \(\newcommand{\bvec}{\mathbf b}\) \(\newcommand{\cvec}{\mathbf c}\) \(\newcommand{\dvec}{\mathbf d}\) \(\newcommand{\dtil}{\widetilde{\mathbf d}}\) \(\newcommand{\evec}{\mathbf e}\) \(\newcommand{\fvec}{\mathbf f}\) \(\newcommand{\nvec}{\mathbf n}\) \(\newcommand{\pvec}{\mathbf p}\) \(\newcommand{\qvec}{\mathbf q}\) \(\newcommand{\svec}{\mathbf s}\) \(\newcommand{\tvec}{\mathbf t}\) \(\newcommand{\uvec}{\mathbf u}\) \(\newcommand{\vvec}{\mathbf v}\) \(\newcommand{\wvec}{\mathbf w}\) \(\newcommand{\xvec}{\mathbf x}\) \(\newcommand{\yvec}{\mathbf y}\) \(\newcommand{\zvec}{\mathbf z}\) \(\newcommand{\rvec}{\mathbf r}\) \(\newcommand{\mvec}{\mathbf m}\) \(\newcommand{\zerovec}{\mathbf 0}\) \(\newcommand{\onevec}{\mathbf 1}\) \(\newcommand{\real}{\mathbb R}\) \(\newcommand{\twovec}[2]{\left[\begin{array}{r}#1 \\ #2 \end{array}\right]}\) \(\newcommand{\ctwovec}[2]{\left[\begin{array}{c}#1 \\ #2 \end{array}\right]}\) \(\newcommand{\threevec}[3]{\left[\begin{array}{r}#1 \\ #2 \\ #3 \end{array}\right]}\) \(\newcommand{\cthreevec}[3]{\left[\begin{array}{c}#1 \\ #2 \\ #3 \end{array}\right]}\) \(\newcommand{\fourvec}[4]{\left[\begin{array}{r}#1 \\ #2 \\ #3 \\ #4 \end{array}\right]}\) \(\newcommand{\cfourvec}[4]{\left[\begin{array}{c}#1 \\ #2 \\ #3 \\ #4 \end{array}\right]}\) \(\newcommand{\fivevec}[5]{\left[\begin{array}{r}#1 \\ #2 \\ #3 \\ #4 \\ #5 \\ \end{array}\right]}\) \(\newcommand{\cfivevec}[5]{\left[\begin{array}{c}#1 \\ #2 \\ #3 \\ #4 \\ #5 \\ \end{array}\right]}\) \(\newcommand{\mattwo}[4]{\left[\begin{array}{rr}#1 \amp #2 \\ #3 \amp #4 \\ \end{array}\right]}\) \(\newcommand{\laspan}[1]{\text{Span}\{#1\}}\) \(\newcommand{\bcal}{\cal B}\) \(\newcommand{\ccal}{\cal C}\) \(\newcommand{\scal}{\cal S}\) \(\newcommand{\wcal}{\cal W}\) \(\newcommand{\ecal}{\cal E}\) \(\newcommand{\coords}[2]{\left\{#1\right\}_{#2}}\) \(\newcommand{\gray}[1]{\color{gray}{#1}}\) \(\newcommand{\lgray}[1]{\color{lightgray}{#1}}\) \(\newcommand{\rank}{\operatorname{rank}}\) \(\newcommand{\row}{\text{Row}}\) \(\newcommand{\col}{\text{Col}}\) \(\renewcommand{\row}{\text{Row}}\) \(\newcommand{\nul}{\text{Nul}}\) \(\newcommand{\var}{\text{Var}}\) \(\newcommand{\corr}{\text{corr}}\) \(\newcommand{\len}[1]{\left|#1\right|}\) \(\newcommand{\bbar}{\overline{\bvec}}\) \(\newcommand{\bhat}{\widehat{\bvec}}\) \(\newcommand{\bperp}{\bvec^\perp}\) \(\newcommand{\xhat}{\widehat{\xvec}}\) \(\newcommand{\vhat}{\widehat{\vvec}}\) \(\newcommand{\uhat}{\widehat{\uvec}}\) \(\newcommand{\what}{\widehat{\wvec}}\) \(\newcommand{\Sighat}{\widehat{\Sigma}}\) \(\newcommand{\lt}{<}\) \(\newcommand{\gt}{>}\) \(\newcommand{\amp}{&}\) \(\definecolor{fillinmathshade}{gray}{0.9}\)Recall that the loci Mendel observed behaved independently of each other; for example, the segregation of seed color alleles was independent from the segregation of alleles for seed shape. This observation was the basis for his Second Law (Independent Assortment), and contributed greatly to our understanding of heredity. However, further research showed that Mendel’s Second Law did not apply to every pair of genes that could be studied. In fact, we now know that alleles of loci that are located close together on the same chromosome tend to be inherited together. This phenomenon is called linkage, and is a major exception to Mendel’s Second Law of Independent Assortment.
The term “recombination” is used in several different contexts in genetics. In reference to heredity, recombination is defined as any process that results in gametes with combinations of alleles that were not present in the gametes of a previous generation (see Figure \(\PageIndex{2}\)). Interchromosomal recombination occurs either through independent assortment of alleles whose loci are on different chromosomes. Intrachromosomal recombination occurs through crossovers between loci on the same chromosomes (as described below). It is important to remember that in both of these cases, recombination is a process that occurs during meiosis (mitotic recombination may also occur in some species, but it is relatively rare). If meiosis results in recombination, the products are said to have a recombinant genotype. On the other hand, if no recombination occurs during meiosis, the products have their original combinations and are said to have a non-recombinant, or parental genotype. Recombination is important because it contributes to the genetic variation that may be observed between individuals within a population and acted upon by selection to produce evolution.

As an example of interchromosomal recombination, consider loci on two different chromosomes as shown in Figure \(\PageIndex{2}\). We know that if these loci are on different chromosomes, there are no physical connections between them, so they are unlinked and will segregate independently as did Mendel’s traits. The segregation depends on the relative orientation of each pair of chromosomes at metaphase. Since the orientation is random and independent of other chromosomes, each of the arrangements (and their meiotic products) is equally possible for two unlinked loci as shown in Figure \(\PageIndex{2}\). More precisely, there is a 50% probability for recombinant genotypes, and a 50% probability for parental genotypes within the gametes produced by a meiocyte with unlinked loci. Indeed, if we examined all of the gametes that could be produced by this individual (which are the products of multiple independent meioses), we would note that approximately 50% of the gametes would be recombinant, and 50% would be parental. Recombination frequency (RF) is simply the number of recombinant gametes, divided by the total number of gametes. A frequency of approximately 50% recombination is therefore a defining characteristic of unlinked loci. Thus the greatest recombinant frequency expected is ~50%.
Having considered unlinked loci above, let us turn to the opposite situation, in which two loci are so close together on a chromosome that the parental combinations of alleles always segregate together (Figure \(\PageIndex{3}\)). This is because during meiosis they are so close that there are no crossover events between the two loci and the alleles at the two loci are physically attached on the same chromatid and so they always segregate together into the same gamete. In this case, no recombinants will be present following meiosis, and the recombination frequency will be 0%. This is complete (or absolute) linkage and is rare, as the loci must be so close together that crossovers are never detected between them.
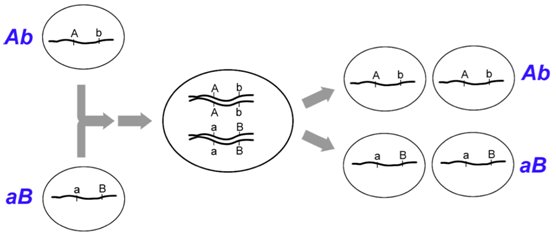
Thus far, we have only considered situations with either no linkage (50% recombination) or complete linkage (0% recombination). It is also possible to obtain recombination frequencies between 0% and 50%, which is a situation we call incomplete (or partial) linkage. Incomplete linkage occurs when two loci are located on the same chromosome but the loci are far enough apart so that crossovers occur between them during some, but not all, meioses. Genes that are on the same chromosome are said to be syntenic regardless of whether they are completely or incompletely linked. All linked genes are syntenic, but not all syntenic genes are linked, as we will learn later.
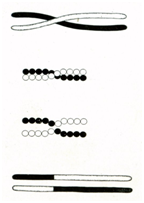
Crossovers occur during prophase I of meiosis, when pairs of homologous chromosomes have aligned with each other in a process called synapsis. Crossing over begins with the breakage of DNA of a pair of non-sister chromatids. The breaks occur at corresponding positions on two non-sister chromatids, and then the ends of non-sister chromatids are connected to each other resulting in a reciprocal exchange of double-stranded DNA (Figure \(\PageIndex{4}\)). Generally every pair of chromosomes has at least one (and often more) crossovers during meioses (Figure \(\PageIndex{5}\)).
Figure \(\PageIndex{5}\): A crossover between two linked loci can generate recombinant genotypes (AB, ab), from the chromatids involved in the crossover. Remember that multiple, independent meioses occur in each organism, so this particular pattern of recombination will not be observed among all the meioses from this individual. (Original-Deyholos-CC:AN)
Because the location of crossovers is essentially random along the chromosome, the greater the distance between two loci, the more likely a crossover will occur between them. Furthermore, loci that are on the same chromosome, but are sufficiently far apart from each other, will on average have multiple crossovers between them and they will behave as though they are completely unlinked. A recombination frequency of 50% is therefore the maximum recombination frequency that can be observed, and is indicative of loci that are either on separate chromosomes, or are located very far apart on the same chromosome.