2.2.1: DNA Fingerprinting, RFLPs and Southern Blotting
- Page ID
- 142215
\( \newcommand{\vecs}[1]{\overset { \scriptstyle \rightharpoonup} {\mathbf{#1}} } \)
\( \newcommand{\vecd}[1]{\overset{-\!-\!\rightharpoonup}{\vphantom{a}\smash {#1}}} \)
\( \newcommand{\id}{\mathrm{id}}\) \( \newcommand{\Span}{\mathrm{span}}\)
( \newcommand{\kernel}{\mathrm{null}\,}\) \( \newcommand{\range}{\mathrm{range}\,}\)
\( \newcommand{\RealPart}{\mathrm{Re}}\) \( \newcommand{\ImaginaryPart}{\mathrm{Im}}\)
\( \newcommand{\Argument}{\mathrm{Arg}}\) \( \newcommand{\norm}[1]{\| #1 \|}\)
\( \newcommand{\inner}[2]{\langle #1, #2 \rangle}\)
\( \newcommand{\Span}{\mathrm{span}}\)
\( \newcommand{\id}{\mathrm{id}}\)
\( \newcommand{\Span}{\mathrm{span}}\)
\( \newcommand{\kernel}{\mathrm{null}\,}\)
\( \newcommand{\range}{\mathrm{range}\,}\)
\( \newcommand{\RealPart}{\mathrm{Re}}\)
\( \newcommand{\ImaginaryPart}{\mathrm{Im}}\)
\( \newcommand{\Argument}{\mathrm{Arg}}\)
\( \newcommand{\norm}[1]{\| #1 \|}\)
\( \newcommand{\inner}[2]{\langle #1, #2 \rangle}\)
\( \newcommand{\Span}{\mathrm{span}}\) \( \newcommand{\AA}{\unicode[.8,0]{x212B}}\)
\( \newcommand{\vectorA}[1]{\vec{#1}} % arrow\)
\( \newcommand{\vectorAt}[1]{\vec{\text{#1}}} % arrow\)
\( \newcommand{\vectorB}[1]{\overset { \scriptstyle \rightharpoonup} {\mathbf{#1}} } \)
\( \newcommand{\vectorC}[1]{\textbf{#1}} \)
\( \newcommand{\vectorD}[1]{\overrightarrow{#1}} \)
\( \newcommand{\vectorDt}[1]{\overrightarrow{\text{#1}}} \)
\( \newcommand{\vectE}[1]{\overset{-\!-\!\rightharpoonup}{\vphantom{a}\smash{\mathbf {#1}}}} \)
\( \newcommand{\vecs}[1]{\overset { \scriptstyle \rightharpoonup} {\mathbf{#1}} } \)
\( \newcommand{\vecd}[1]{\overset{-\!-\!\rightharpoonup}{\vphantom{a}\smash {#1}}} \)
\(\newcommand{\avec}{\mathbf a}\) \(\newcommand{\bvec}{\mathbf b}\) \(\newcommand{\cvec}{\mathbf c}\) \(\newcommand{\dvec}{\mathbf d}\) \(\newcommand{\dtil}{\widetilde{\mathbf d}}\) \(\newcommand{\evec}{\mathbf e}\) \(\newcommand{\fvec}{\mathbf f}\) \(\newcommand{\nvec}{\mathbf n}\) \(\newcommand{\pvec}{\mathbf p}\) \(\newcommand{\qvec}{\mathbf q}\) \(\newcommand{\svec}{\mathbf s}\) \(\newcommand{\tvec}{\mathbf t}\) \(\newcommand{\uvec}{\mathbf u}\) \(\newcommand{\vvec}{\mathbf v}\) \(\newcommand{\wvec}{\mathbf w}\) \(\newcommand{\xvec}{\mathbf x}\) \(\newcommand{\yvec}{\mathbf y}\) \(\newcommand{\zvec}{\mathbf z}\) \(\newcommand{\rvec}{\mathbf r}\) \(\newcommand{\mvec}{\mathbf m}\) \(\newcommand{\zerovec}{\mathbf 0}\) \(\newcommand{\onevec}{\mathbf 1}\) \(\newcommand{\real}{\mathbb R}\) \(\newcommand{\twovec}[2]{\left[\begin{array}{r}#1 \\ #2 \end{array}\right]}\) \(\newcommand{\ctwovec}[2]{\left[\begin{array}{c}#1 \\ #2 \end{array}\right]}\) \(\newcommand{\threevec}[3]{\left[\begin{array}{r}#1 \\ #2 \\ #3 \end{array}\right]}\) \(\newcommand{\cthreevec}[3]{\left[\begin{array}{c}#1 \\ #2 \\ #3 \end{array}\right]}\) \(\newcommand{\fourvec}[4]{\left[\begin{array}{r}#1 \\ #2 \\ #3 \\ #4 \end{array}\right]}\) \(\newcommand{\cfourvec}[4]{\left[\begin{array}{c}#1 \\ #2 \\ #3 \\ #4 \end{array}\right]}\) \(\newcommand{\fivevec}[5]{\left[\begin{array}{r}#1 \\ #2 \\ #3 \\ #4 \\ #5 \\ \end{array}\right]}\) \(\newcommand{\cfivevec}[5]{\left[\begin{array}{c}#1 \\ #2 \\ #3 \\ #4 \\ #5 \\ \end{array}\right]}\) \(\newcommand{\mattwo}[4]{\left[\begin{array}{rr}#1 \amp #2 \\ #3 \amp #4 \\ \end{array}\right]}\) \(\newcommand{\laspan}[1]{\text{Span}\{#1\}}\) \(\newcommand{\bcal}{\cal B}\) \(\newcommand{\ccal}{\cal C}\) \(\newcommand{\scal}{\cal S}\) \(\newcommand{\wcal}{\cal W}\) \(\newcommand{\ecal}{\cal E}\) \(\newcommand{\coords}[2]{\left\{#1\right\}_{#2}}\) \(\newcommand{\gray}[1]{\color{gray}{#1}}\) \(\newcommand{\lgray}[1]{\color{lightgray}{#1}}\) \(\newcommand{\rank}{\operatorname{rank}}\) \(\newcommand{\row}{\text{Row}}\) \(\newcommand{\col}{\text{Col}}\) \(\renewcommand{\row}{\text{Row}}\) \(\newcommand{\nul}{\text{Nul}}\) \(\newcommand{\var}{\text{Var}}\) \(\newcommand{\corr}{\text{corr}}\) \(\newcommand{\len}[1]{\left|#1\right|}\) \(\newcommand{\bbar}{\overline{\bvec}}\) \(\newcommand{\bhat}{\widehat{\bvec}}\) \(\newcommand{\bperp}{\bvec^\perp}\) \(\newcommand{\xhat}{\widehat{\xvec}}\) \(\newcommand{\vhat}{\widehat{\vvec}}\) \(\newcommand{\uhat}{\widehat{\uvec}}\) \(\newcommand{\what}{\widehat{\wvec}}\) \(\newcommand{\Sighat}{\widehat{\Sigma}}\) \(\newcommand{\lt}{<}\) \(\newcommand{\gt}{>}\) \(\newcommand{\amp}{&}\) \(\definecolor{fillinmathshade}{gray}{0.9}\)A DNA fingerprint is a characteristic pattern of bands on a DNA gel that results from cutting DNA with a restriction enzyme. Creating DNA fingerprints -- and in particular, DNA fingerprints from a complex mixture of DNA such as a human genome -- requires a fairly sophisticated understanding of several laboratory methods, including restriction digests, gel electrophoresis, and Southern blots.
Creating a DNA Fingerprint
Restriction Enzymes
DNA fingerprints are created by first isolating DNA from an unknown sample to be identified and compared with known samples. If the samples match, it enables identification. The isolated DNA (i.e. DNA that has been removed from cells and other cell components) is mixed with a restriction enzyme to create a fingerprint. The restriction enzyme will cut the DNA in a pattern that will differ from DNA from other sources, unless the identify of the DNA is the same (matching known and unknown samples enables identification).
The DNA fragments produced by the restriction enzyme are separated by size using an approach called gel electrophoresis (see the Gel Electrophoresis section below). The result is a pattern of bands that can be compared with other patterns from known samples. If fingerprints match, it likely means that the DNA originated from the same organism. For paternity testing, half of the fingerprint will originate from the biological mother and half of the fingerprint will originate from the biological father.
Restriction enzymes are found in some bacteria and have been isolated to use for a variety of biotechnologies such as DNA fingerprinting. These enzymes cut DNA at a characteristic recognition site. Recognition sites are different for each restriction enzyme. Typically, recognition sites are palindromic, that is they read the same backwards and forwards. Ordinary words that are palindromic include "mom," "dad," "wow," and "racecar." With DNA, a palindrome is based on reading one DNA strand 5' to 3' and comparing it with its complement DNA strand as read 5' to 3'. For example:
5'-GAATTC-3'
3'-CTTAAG-5'
Notice that the complementary DNA strands above, if reading from the 5' end, have the same sequence: 5'-GAATTC-3'. This example is a recognition sequence from the restriction enzyme known as EcoRI. The "Eco" part of the enzyme name comes from the fact that this enzyme originates from Escherichia coli (E. coli). The rest of the restriction enzyme name comes from the strain of the organism (in this case the R strain) and the order in which the enzyme was discovered (in this case it is the first restriction enzyme discovered from the R strain of E. coli - I is the Roman numeral for 1).
In the example of EcoRI, this enzyme cuts the DNA between the "G" and the "A" on the 5' side. As a result, both the top and bottom DNA strands are cut and only held together by the few hydrogen bonds between the 5'-AATT-3' on both strands. Because of this (and that hydrogen bonds are rather weak attractions), the two DNA strands fully separate leaving the 5'-AATT-3' overhangs on both broken strands. These overhangs are called sticky ends.
Restriction enzymes will identify every location on a DNA molecule with the recognition sequence and cut the DNA there. This means the a restriction enzyme will likely make multiple cuts in the DNA. This will produce DNA fragments of different numbers of fragments with different sizes based on the base sequence of the DNA. The fragment sizes and number of fragments produces the DNA fingerprint used for identification purposes in DNA fingerprinting.
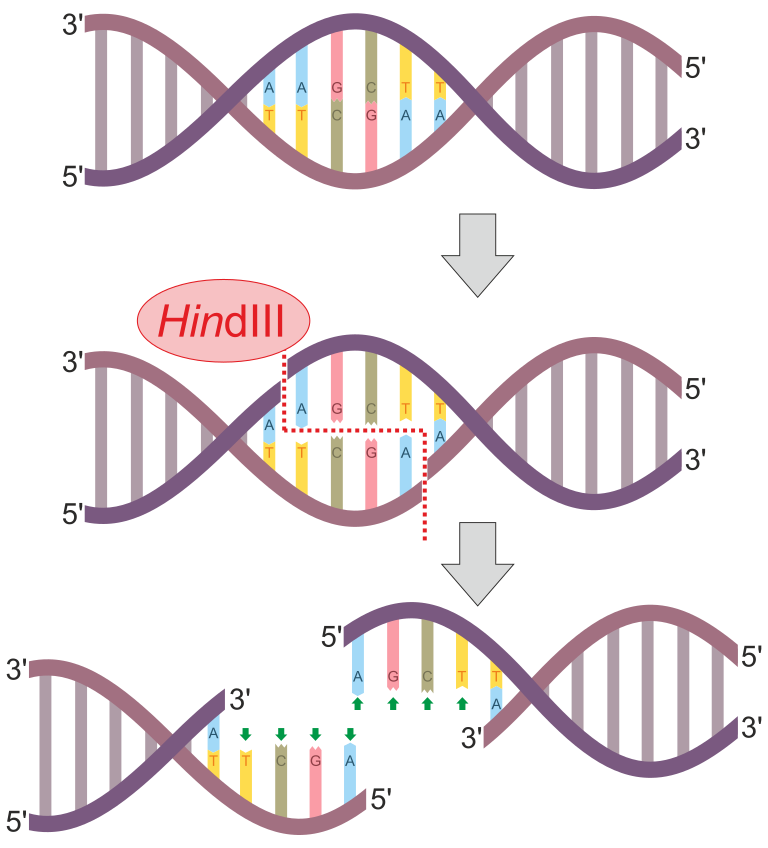
Figure 1: HindIII is an example of a restriction enzyme. This enzyme has the recognition sequence of 5'-AAGCTT-3'. Notice that this sequence is also a palindrome since its complementary strand will also have the 5'-AAGCTT-3' sequence (just antiparallel). This enzyme scans the entire DNA molecule for this specific sequence. When this sequence is found, it will cut between the "A" and "A" bases. This happens on both DNA strands to produce sticky ends (overhangs). In this case, the overhangs have the sequence 5'-AGCT-3'.
DNA Fingerprinting and Restriction Fragment Length Polymorphisms (RFLPs) Analysis
Restriction enzyme recognition sites are short (only a few nucleotides long), sequence-specific palindromes, and may be found throughout the genome. Thus, differences in DNA sequences in the genomes of individuals will lead to differences in distribution of restriction enzyme recognition sites that can be visualized as distinct fingerprints using a technique called gel electrophoresis (see the seciton on Gel Electrophoresis below). When a polymorphism results in the change of the length of a restriction fragment on a gel, we say it is a restriction fragment length polymorphism (RFLP).
RFLP analysis has many practical applications in both medicine and forensic science. For example, epidemiologists use RFLP analysis to track and identify the source of specific microorganisms implicated in outbreaks of food poisoning or certain infectious diseases. RFLP analysis can also be used on human DNA to determine inheritance patterns of chromosomes with variant genes, including those associated with heritable diseases or to establish paternity.
Forensic scientists use RFLP analysis as a form of DNA fingerprinting, which is useful for analyzing DNA obtained from crime scenes, suspects, and victims. DNA samples are collected, the numbers of copies of the sample DNA molecules are increased using PCR, and then subjected to restriction enzyme digestion and agarose gel electrophoresis to generate specific banding patterns. By comparing the banding patterns of samples collected from the crime scene against those collected from suspects or victims, investigators can definitively determine whether DNA evidence collected at the scene was left behind by suspects or victims.

Figure 2: RFLP analysis can be used to differentiate DNA sequences. In this example, a normal chromosome is digested into two fragments, whereas digestion of a mutated chromosome produces only one fragment. The small red arrows pointing to the two different chromosome segments show the locations of the restriction enzyme recognition sites. After digestion and agarose gel electrophoresis, the banding patterns reflect the change by showing the loss of two shorter bands and the gain of a longer band. Each band produced by gel electrophoresis is a DNA fragment of different size. (credit: modification of work by National Center for Biotechnology Information)
Gel Electrophoresis
After digesting DNA with a restriction enzyme, how do we separate them to create a DNA fingerprint? The most common method is agarose gel electrophoresis. Gel electrophoresis methods are commonly used to separate biological molecules based on size and biochemical characteristics, such as charge and polarity. Agarose gel electrophoresis is widely used to separate DNA (or RNA) of varying sizes that may be generated by restriction enzyme digestion (such as DNA fingerprinting / RFLP analysis) or by other means, such as the PCR.
DNA molecules have an overall negative charge. This is due to negative charges on the phosphate groups of its nucleotides. As a result, DNA can be pulled toward a positive charge. This is how gel electrophoresis pulls DNA through an agarose gel.
Due to its negatively charged backbone, DNA is strongly attracted to a positive electrode. In agarose gel electrophoresis, the gel is oriented horizontally in a buffer solution. Samples are loaded into sample wells on the side of the gel closest to the negative electrode, then drawn through the molecular sieve of the agarose matrix toward the positive electrode. The agarose matrix impedes the movement of larger molecules through the gel, whereas smaller molecules pass through more readily. Thus, the distance of migration is inversely correlated to the size of the DNA fragment, with smaller fragments traveling a longer distance through the gel. Sizes of DNA fragments within a sample can be estimated by comparison to fragments of known size in a DNA ladder also run on the same gel.
Small DNA fragments travel farther through the electrophoresis gel than larger DNA fragments.
You can think about this as being analagous to rocks in a river. Large boulders do not move very far, even if the current is swift, but small pebbles are capable of moving great distances in river's current. Similarly, large DNA cannot move well through the gel and will remain closer to the wells and smaller DNA can move easily through the gel and will move farther away from the wells.
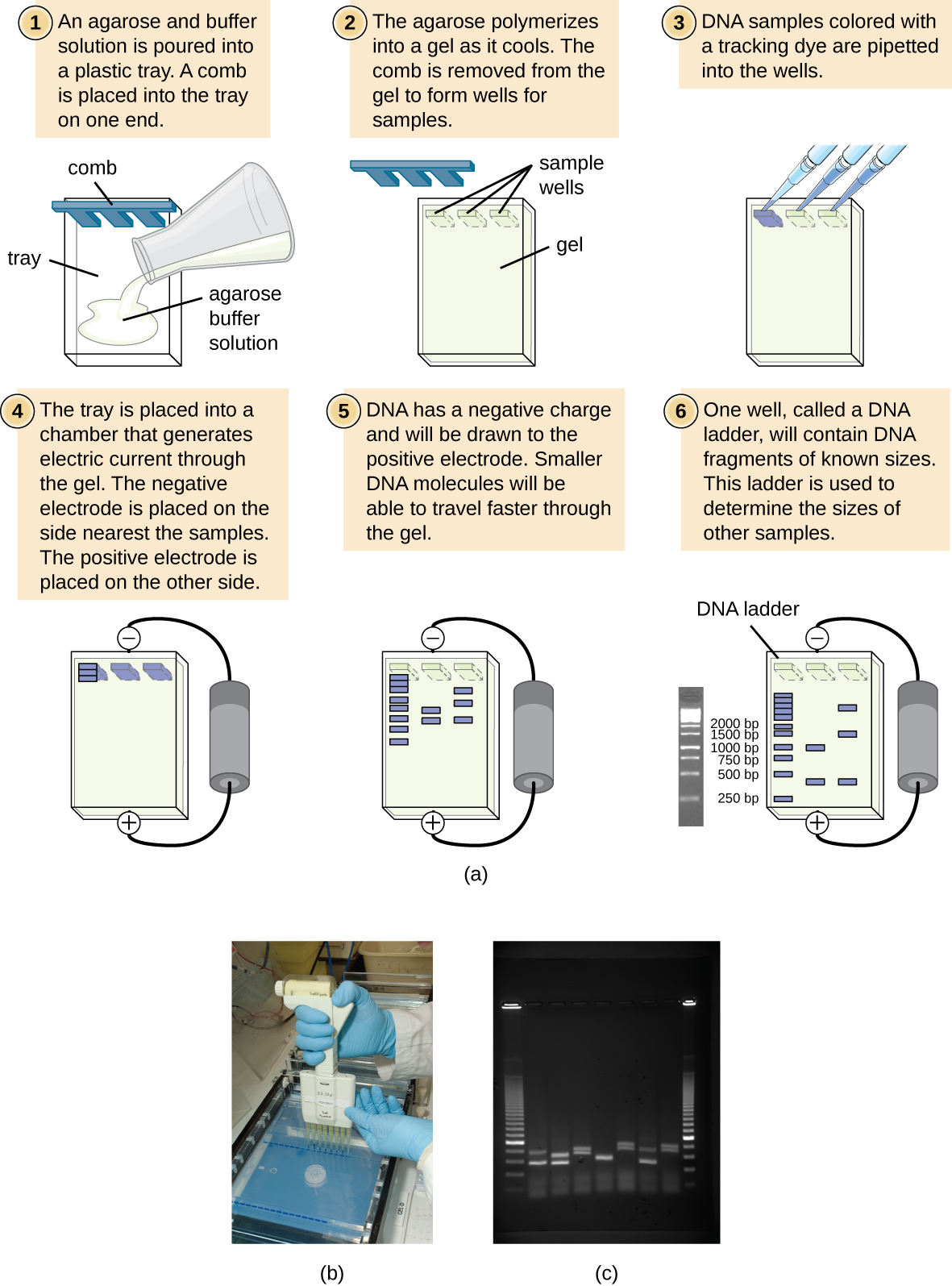
Figure 3: (a) The process of agarose gel electrophoresis. (b) A researcher loading samples into a gel. (c) This photograph shows a completed electrophoresis run on an agarose gel. The DNA ladder is located in lanes 1 and 9. Seven samples are located in lanes 2 through 8. The gel was stained with ethidium bromide and photographed under ultraviolet light. (credit a: modification of work by Magnus Manske; credit b: modification of work by U.S. Department of Agriculture; credit c: modification of work by James Jacob)
Nucleic Acid Probes
When making a DNA fingerprint from a small piece of DNA such as a plasmid, a restriction digest and followed by agarose gel electrophoresis is all you need. However, what if you wanted to do so with a complicated mixture of DNA such as a human genome? Running a digested sample of human DNA on an agarose gel results in a "smear" because the restriction enzyme cuts SO many times and produces SO many bands that they become indistinguishable. In order to make an interpretable DNA fingerprint, we need a way of identifying specific bands in the gel with specific sequences.
To see how we do so, recall that a single strand of DNA will preferentially bind to a piece of DNA whose base sequence is complementary. Thus, if a researcher knows a portion of the DNA sequence of a piece of DNA they would like to identify, they can design a DNA probe, a single-stranded DNA fragment that is complementary to part of the gene of interest and different from other DNA sequences in the sample, that will stick to it. The DNA probe may be synthesized chemically by commercial laboratories, or it may be created by cloning, isolating, and denaturing a DNA fragment from a living organism. In either case, the DNA probe must be labeled with a molecular tag or beacon, such as a radioactive phosphorus atom (as is used for autoradiography) or a fluorescent dye (as is used in fluorescent in situ hybridization, or FISH), so that the probe and the DNA it binds to can be seen (Figure \(\PageIndex{1}\)). The DNA sample being probed must also be denatured to make it single-stranded so that the single-stranded DNA probe can anneal to the single-stranded DNA sample at locations where their sequences are complementary.
Figure \(\PageIndex{1}\): DNA probes can be used to confirm the presence of a suspected pathogen in patient samples. This diagram illustrates how a DNA probe can be used to search for a gene of interest associated with the suspected pathogen.
Putting it all together: Southern Blotting
Unfortunately, you typically can't probe nucleic-acid samples within a gel because as the DNA probe soaks into a gel, the sample nucleic acids within the gel diffuse out. Instead, blotting techniques are commonly used to transfer nucleic acids to a thin, positively charged membrane made of nitrocellulose or nylon. In the Southern blot technique, developed by Sir Edwin Southern in 1975, DNA fragments within a sample are first separated by agarose gel electrophoresis and then transferred to a membrane through capillary action (Figure \(\PageIndex{4}\)). The DNA fragments that bind to the surface of the membrane are then exposed to a specific single-stranded DNA probe labeled with a radioactive or fluorescent molecular beacon to aid in detection. Southern blots may be used to detect the presence of certain DNA sequences in a given DNA sample. Once the target DNA within the membrane is visualized, researchers can cut out the portion of the membrane containing the fragment to recover the DNA fragment of interest.
Figure \(\PageIndex{4}\): In the Southern blot technique, DNA fragments are first separated by agarose gel electrophoresis, then transferred by capillary action to a nylon membrane, which is then soaked with a DNA probe tagged with a molecular beacon for easy visualization.
Variations of the Southern blot—the dot blot, slot blot, and the spot blot—do not involve electrophoresis, but instead concentrate DNA from a sample into a small location on a membrane. After hybridization with a DNA probe, the signal intensity detected is measured, allowing the researcher to estimate the amount of target DNA present within the sample.
A colony blot is another variation of the Southern blot in which colonies representing different clones in a genomic library are transferred to a membrane by pressing the membrane onto the culture plate. The cells on the membrane are lysed and the membrane can then be probed to determine which colonies within a genomic library harbor the target gene. Because the colonies on the plate are still growing, the cells of interest can be isolated from the plate.
In the northern blot, another variation of the Southern blot, RNA (not DNA) is immobilized on the membrane and probed. Northern blots are typically used to detect the amount of mRNA made through gene expression within a tissue or organism sample.
Using Southern Blots to determine RFLP genotypes
Remember that a restriction-length fragment polymorphism is a polymorphism that causes a change in the length of a restriction fragment. If a researcher knows the sequence of DNA near the restriction site, they can design a DNA probe to interrogate just that site:
Sample A only reveals one band after processing because this person is homologous for the same allele. Sample B is heterozygous and reveals three bands.
Credit: Retama (CC-BY-SA 4.0)
And remember, RFLPs are inherited with a Mendelian pattern just like any other gene. Thus, RFLPs represent inheritable markers and can reveal relationships between different individuals. A pedigree can illustrate the relationship of the inherited alleles. The technique can be more informative if using multiple probes simultaneously for different loci or to use multi-locus probes that hybridize to multiple locations.
Homologous chromosomes with restriction sites noted by triangles. the rectangle sitting on the chromosomes correspond to a probe locus. Credit: Jeremy Seto (CC0)
While RFLPs can arise from SNPs (single-nucleotide polymorphisms) that create or destroy a restriction enzyme recognition sequence, they may also be caused by the expansion or contraction of repeated elements such as microsatellites between restriction sites. These repeated elements of DNA are referred to as Variable Number Tandem Repeats (VNTR) and illustrate polymorphisms that normally occur in non-coding regions of the genome.
RFLPs may arise from differences in the STR/VNTR repeats between restriction sites. Credit: Jeremy Seto (CC0)