17.2: Mutualisms
- Page ID
- 92884
\( \newcommand{\vecs}[1]{\overset { \scriptstyle \rightharpoonup} {\mathbf{#1}} } \)
\( \newcommand{\vecd}[1]{\overset{-\!-\!\rightharpoonup}{\vphantom{a}\smash {#1}}} \)
\( \newcommand{\id}{\mathrm{id}}\) \( \newcommand{\Span}{\mathrm{span}}\)
( \newcommand{\kernel}{\mathrm{null}\,}\) \( \newcommand{\range}{\mathrm{range}\,}\)
\( \newcommand{\RealPart}{\mathrm{Re}}\) \( \newcommand{\ImaginaryPart}{\mathrm{Im}}\)
\( \newcommand{\Argument}{\mathrm{Arg}}\) \( \newcommand{\norm}[1]{\| #1 \|}\)
\( \newcommand{\inner}[2]{\langle #1, #2 \rangle}\)
\( \newcommand{\Span}{\mathrm{span}}\)
\( \newcommand{\id}{\mathrm{id}}\)
\( \newcommand{\Span}{\mathrm{span}}\)
\( \newcommand{\kernel}{\mathrm{null}\,}\)
\( \newcommand{\range}{\mathrm{range}\,}\)
\( \newcommand{\RealPart}{\mathrm{Re}}\)
\( \newcommand{\ImaginaryPart}{\mathrm{Im}}\)
\( \newcommand{\Argument}{\mathrm{Arg}}\)
\( \newcommand{\norm}[1]{\| #1 \|}\)
\( \newcommand{\inner}[2]{\langle #1, #2 \rangle}\)
\( \newcommand{\Span}{\mathrm{span}}\) \( \newcommand{\AA}{\unicode[.8,0]{x212B}}\)
\( \newcommand{\vectorA}[1]{\vec{#1}} % arrow\)
\( \newcommand{\vectorAt}[1]{\vec{\text{#1}}} % arrow\)
\( \newcommand{\vectorB}[1]{\overset { \scriptstyle \rightharpoonup} {\mathbf{#1}} } \)
\( \newcommand{\vectorC}[1]{\textbf{#1}} \)
\( \newcommand{\vectorD}[1]{\overrightarrow{#1}} \)
\( \newcommand{\vectorDt}[1]{\overrightarrow{\text{#1}}} \)
\( \newcommand{\vectE}[1]{\overset{-\!-\!\rightharpoonup}{\vphantom{a}\smash{\mathbf {#1}}}} \)
\( \newcommand{\vecs}[1]{\overset { \scriptstyle \rightharpoonup} {\mathbf{#1}} } \)
\( \newcommand{\vecd}[1]{\overset{-\!-\!\rightharpoonup}{\vphantom{a}\smash {#1}}} \)
\(\newcommand{\avec}{\mathbf a}\) \(\newcommand{\bvec}{\mathbf b}\) \(\newcommand{\cvec}{\mathbf c}\) \(\newcommand{\dvec}{\mathbf d}\) \(\newcommand{\dtil}{\widetilde{\mathbf d}}\) \(\newcommand{\evec}{\mathbf e}\) \(\newcommand{\fvec}{\mathbf f}\) \(\newcommand{\nvec}{\mathbf n}\) \(\newcommand{\pvec}{\mathbf p}\) \(\newcommand{\qvec}{\mathbf q}\) \(\newcommand{\svec}{\mathbf s}\) \(\newcommand{\tvec}{\mathbf t}\) \(\newcommand{\uvec}{\mathbf u}\) \(\newcommand{\vvec}{\mathbf v}\) \(\newcommand{\wvec}{\mathbf w}\) \(\newcommand{\xvec}{\mathbf x}\) \(\newcommand{\yvec}{\mathbf y}\) \(\newcommand{\zvec}{\mathbf z}\) \(\newcommand{\rvec}{\mathbf r}\) \(\newcommand{\mvec}{\mathbf m}\) \(\newcommand{\zerovec}{\mathbf 0}\) \(\newcommand{\onevec}{\mathbf 1}\) \(\newcommand{\real}{\mathbb R}\) \(\newcommand{\twovec}[2]{\left[\begin{array}{r}#1 \\ #2 \end{array}\right]}\) \(\newcommand{\ctwovec}[2]{\left[\begin{array}{c}#1 \\ #2 \end{array}\right]}\) \(\newcommand{\threevec}[3]{\left[\begin{array}{r}#1 \\ #2 \\ #3 \end{array}\right]}\) \(\newcommand{\cthreevec}[3]{\left[\begin{array}{c}#1 \\ #2 \\ #3 \end{array}\right]}\) \(\newcommand{\fourvec}[4]{\left[\begin{array}{r}#1 \\ #2 \\ #3 \\ #4 \end{array}\right]}\) \(\newcommand{\cfourvec}[4]{\left[\begin{array}{c}#1 \\ #2 \\ #3 \\ #4 \end{array}\right]}\) \(\newcommand{\fivevec}[5]{\left[\begin{array}{r}#1 \\ #2 \\ #3 \\ #4 \\ #5 \\ \end{array}\right]}\) \(\newcommand{\cfivevec}[5]{\left[\begin{array}{c}#1 \\ #2 \\ #3 \\ #4 \\ #5 \\ \end{array}\right]}\) \(\newcommand{\mattwo}[4]{\left[\begin{array}{rr}#1 \amp #2 \\ #3 \amp #4 \\ \end{array}\right]}\) \(\newcommand{\laspan}[1]{\text{Span}\{#1\}}\) \(\newcommand{\bcal}{\cal B}\) \(\newcommand{\ccal}{\cal C}\) \(\newcommand{\scal}{\cal S}\) \(\newcommand{\wcal}{\cal W}\) \(\newcommand{\ecal}{\cal E}\) \(\newcommand{\coords}[2]{\left\{#1\right\}_{#2}}\) \(\newcommand{\gray}[1]{\color{gray}{#1}}\) \(\newcommand{\lgray}[1]{\color{lightgray}{#1}}\) \(\newcommand{\rank}{\operatorname{rank}}\) \(\newcommand{\row}{\text{Row}}\) \(\newcommand{\col}{\text{Col}}\) \(\renewcommand{\row}{\text{Row}}\) \(\newcommand{\nul}{\text{Nul}}\) \(\newcommand{\var}{\text{Var}}\) \(\newcommand{\corr}{\text{corr}}\) \(\newcommand{\len}[1]{\left|#1\right|}\) \(\newcommand{\bbar}{\overline{\bvec}}\) \(\newcommand{\bhat}{\widehat{\bvec}}\) \(\newcommand{\bperp}{\bvec^\perp}\) \(\newcommand{\xhat}{\widehat{\xvec}}\) \(\newcommand{\vhat}{\widehat{\vvec}}\) \(\newcommand{\uhat}{\widehat{\uvec}}\) \(\newcommand{\what}{\widehat{\wvec}}\) \(\newcommand{\Sighat}{\widehat{\Sigma}}\) \(\newcommand{\lt}{<}\) \(\newcommand{\gt}{>}\) \(\newcommand{\amp}{&}\) \(\definecolor{fillinmathshade}{gray}{0.9}\)Introduction to Mutualisms
Mutualism
A mutualism occurs when two species benefit from their interaction. For example, termites have a mutualistic relationship with protists that live in the insect’s gut (Figure \(\PageIndex{2}\)a). The termite benefits from the ability of the protists to digest cellulose. However, the protists are able to digest cellulose only because of the presence of symbiotic bacteria within their cells that produce the cellulase enzyme. The termite itself cannot do this: without the protozoa, it would not be able to obtain energy from its food (cellulose from the wood it chews and eats). The protozoa benefit by having a protective environment and a constant supply of food from the wood-chewing actions of the termite. In turn, the protists benefit from the enzymes provided by their bacterial endosymbionts, while the bacteria benefit from a doubly protective environment and a constant source of nutrients from two hosts.
Lichen are a mutualistic relationship between a fungus and photosynthetic algae or cyanobacteria (Figure \(\PageIndex{2}\)b). The glucose produced by the algae provides nourishment for both organisms, whereas the physical structure of the lichen protects the algae from the elements and makes certain nutrients in the atmosphere more available to the algae. The algae of lichens can live independently given the right environment, but many of the fungal partners are unable to live on their own.
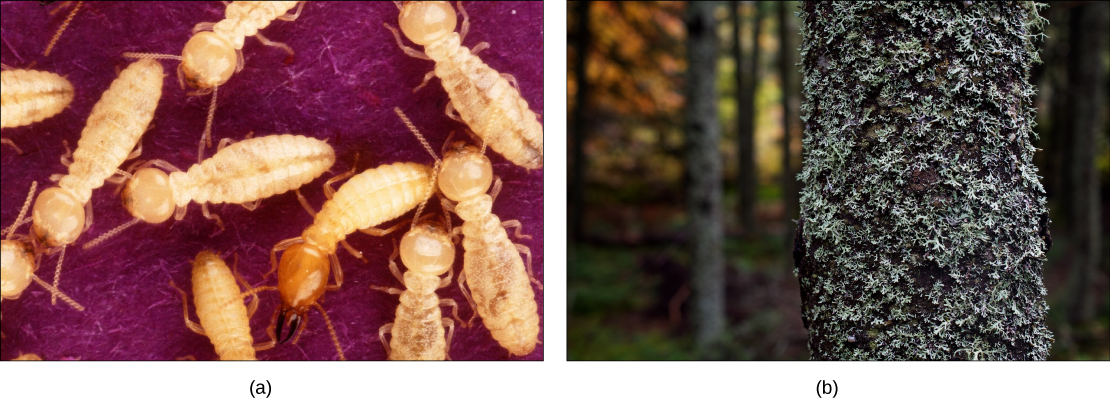
Types of Mutualisms
Resource-resource relationships
Mutualistic relationships can be thought of as a form of "biological barter" in mycorrhizal associations between plant roots and fungi, with the plant providing carbohydrates to the fungus in return for primarily phosphate but also nitrogenous compounds. Other examples include rhizobia bacteria that fix nitrogen for leguminous plants (family Fabaceae) in return for energy-containing carbohydrates.
Service-resource relationships

Service-resource relationships are common. Three important types are pollination, cleaning symbiosis, and zoochory. In pollination, a plant trades food resources in the form of nectar or pollen for the service of pollen dispersal. Phagophiles feed (resource) on ectoparasites, thereby providing anti-pest service, as in cleaning symbiosis ()Figure \(\PageIndex{2}\). Elacatinus and Gobiosoma, genera of gobies, feed on ectoparasites of their clients while cleaning them and zoochory is the dispersal of the seeds of plants by animals. This is similar to pollination in that the plant produces food resources (for example, fleshy fruit, overabundance of seeds) for animals that disperse the seeds (service). Plants may advertise these resources using color and a variety of other fruit characteristics. Another type is ant protection of aphids, where the aphids trade sugar-rich honeydew (a by-product of their mode of feeding on plant sap) in return for defense against predators such as ladybugs.
Service-service relationships

Strict service-service interactions are very rare, for reasons that are far from clear. One example is the relationship between sea anemones and anemone fish in the family Pomacentridae: the anemones provide the fish with protection from predators (which cannot tolerate the stings of the anemone's tentacles) and the fish defend the anemones against butterflyfish (family Chaetodontidae), which eat anemones (Figure \(\PageIndex{3}\). However, in common with many mutualisms, there is more than one aspect to it: in the anemonefish-anemone mutualism, waste ammonia from the fish feeds the symbiotic algae that are found in the anemone's tentacles. Therefore, what appears to be a service-service mutualism in fact has a service-resource component.
A second example is that of the relationship between some ants in the genus Pseudomyrmex and trees in the genus Acacia, such as the whistling thorn and bullhorn acacia. The ants nest inside the plant's thorns. In exchange for shelter, the ants protect acacias from attack by herbivores (which they frequently eat when those are small enough, introducing a resource component to this service-service relationship) and competition from other plants by trimming back vegetation that would shade the acacia. In addition, another service-resource component is present, as the ants regularly feed on lipid-rich food-bodies called Beltian bodies that are on the Acacia plant.
Evolution of Mutualisms
Many mutualisms are thought to have evolved from antagonistic interactions. Several studies have determined ecological or evolutionary conditions driving transitions from mutualism to antagonism (mutualism breakdown), yet limited theory has been developed to mechanistically explain the evolutionary transitions from antagonism to mutualism. Models of virulence predict evolution of reduced antagonism, perhaps toward mutualism, in cases of parasitism where host fidelity is high and availability of alternate hosts is low. In these models, host fidelity is achieved when lineages of parasites and their host are tightly linked through vertical transmission from parent to offspring.
Compelling evidence for the ‘virulence theory’ comes from interactions involving microbial parasites. For example, Wolbachia are endosymbiotic bacteria that live inside the cells of their invertebrate hosts. They are transmitted directly from mother to offspring, and spread through populations by manipulating the reproduction of their hosts. The most common reproductive manipulation responsible for the spread of these bacteria, called “cytoplasmic incompatibility,” arises when infected males mate with uninfected females, resulting in fewer offspring than normal. There are fitness costs for the hosts associated with Wolbachia infections, most commonly involving a reduction in egg production. The virulence theory predicts and evidence suggests that there is selection for the bacteria to evolve a more benign lifestyle, changing the bacterium from being parasitic to more mutualistic, where Wolbachia-infected hosts have higher rates of egg production than their uninfected counterparts.
Reciprocal evolutionary responses are most likely important to the evolution of symbiotic mutualisms, and subsequent diversification may be the result of the mutualism improving niche width, population size, or transition into adaptive zones. However, with the exception of plant-pollinator interactions, it is unclear how important mutualistic coevolution is as a driving force of diversification, especially as compared to the well-established mechanisms associated with competitive and antagonistic coevolution.
Plant-pollinator interactions are powerful tools of evolution because pollinators transport gametes (via pollen) between flowers. Plant-pollinator relationships are thought to be critical to the historical diversification of angiosperm flowers. In fact, up to 25% of plant speciation events could be a result of interactions with pollinators.
The obligate pollinating seed-predation mutualisms of the yucca and yucca moth and fig and fig wasps are attributed to coevolution diversification because the intimacy of the association may prevent species from making phylogenetically drastic switches to new partners. Interacting with a distant relative of your partner may not be an option if coevolution has created incompatibilities in specialized traits required for the interaction (Figure \(\PageIndex{4}\)).
For instance, if laying eggs into yucca flowers requires a particular complement of ovipositor traits and moth behaviors, we might predict finding host switches only between closely related yuccas. Initial analyses of yucca and yucca moth phylogenies suggest that the trees are somewhat congruent, although there have been at least two instances of moths switching hosts to quite distantly related yuccas. The interaction between yuccas and yucca moths is clearly one that is highly specialized and coevolved yet we do not find a phylogenetic pattern of perfect matching between plant and insect.
However, there is also evidence opposing the primacy of mutualistic coevolution in these relationships. Figs have been shown to be successfully pollinated regardless of pollinator species and pollinator host-switch may be more frequent than co-speciation events (Figure \(\PageIndex{5}\)). There is also evidence that geographic isolation plays a significant role in yucca diversification. These together suggest that while coevolution plays a role in the emergence of these mutualisms, it is not the exclusive mechanism and may not even be the primary one.
Attributions
This chapter was written by Aaron Howard.
References
Ollerton, J. 2006. "Biological Barter": Interactions of Specialization Compared across Different Mutualisms. pp. 411–435 in: Waser, N.M. & Ollerton, J. (Eds) Plant-Pollinator Interactions: From Specialization to Generalization. University of Chicago Press.
Denison, RF; Kiers, ET (2004). "Why are most rhizobia beneficial to their plant hosts, rather than parasitic". Microbes and Infection. 6 (13): 1235–1239. doi:10.1016/j.micinf.2004.08.005. PMID 15488744.
M.C. Soares; I.M. Côté; S.C. Cardoso & R.Bshary (August 2008). "The cleaning goby mutualism: a system without punishment, partner switching or tactile stimulation" (PDF). Journal of Zoology. 276 (3): 306–312. doi:10.1111/j.1469-7998.2008.00489.x.
Lim, Ganges; Burns, Kevin C. (24 November 2021). "Do fruit reflectance properties affect avian frugivory in New Zealand?". New Zealand Journal of Botany: 1–11. doi:10.1080/0028825X.2021.2001664. ISSN 0028-825X. S2CID 244683146.
Porat, D.; Chadwick-Furman, N. E. (2004). "Effects of anemonefish on giant sea anemones: expansion behavior, growth, and survival". Hydrobiologia. 530 (1–3): 513–520. doi:10.1007/s10750-004-2688-y. S2CID 2251533.
Porat, D.; Chadwick-Furman, N. E. (2005). "Effects of anemonefish on giant sea anemones: ammonium uptake, zooxanthella content and tissue regeneration". Mar. Freshw.Behav. Phys. 38: 43–51.
"Swollen Thorn Acacias". www2.palomar.edu. Retrieved 22 February 2019.
Johnson, C.A., Smith, G.P., Yule, K. et al. Coevolutionary transitions from antagonism to mutualism explained by the Co-Opted Antagonist Hypothesis. Nat Commun 12, 2867 (2021). https://doi.org/10.1038/s41467-021-23177-x
Segraves, K.A. Branching Out with Coevolutionary Trees. Evo Edu Outreach 3, 62–70 (2010). https://doi.org/10.1007/s12052-009-0199-z
Wang, G., Zhang, X., Herre, E.A. et al. Genomic evidence of prevalent hybridization throughout the evolutionary history of the fig-wasp pollination mutualism. Nat Commun 12, 718 (2021). https://doi.org/10.1038/s41467-021-20957-3
Weeks, A., Turelli, M., Harcombe, W., Reynolds, K. & Hoffmann, A. From parasite to mutualist: rapid evolution of Wolbachia in natural populations of Drosophila. PLoS Biol. 5, e114 (2007).
References
Kiers, T. E., Palmer, T. M., Ives, A. R., Bruno, J. F. & Bronstein, J. L. Mutualisms in a changing world: an evolutionary perspective. Ecol. Lett. 13, 1459–1474 (2010).
Sachs, J. & Simms, E. Pathways to mutualism breakdown. Trends Ecol. Evol. 21, 585–592 (2006).
de Mazancourt, C., Loreau, M. & Dieckmann, U. Can the evolution of plant defense lead to plant‐herbivore mutualism? Am. Nat. 158, 109–123 (2001).
de Mazancourt, C., Loreau, M. & Dieckmann, U. Understanding mutualism when there is adaptation to the partner. J. Ecol. 93, 305–314 (2005).
Yamamura, N. Vertical transmission and evolution of mutualism from parasitism. Theor. Popul. Biol. 44, 95–109 (1993).
Yamamura, N. Evolution of mutualistic symbiosis: a differential equation model. Res. Popul. Ecol. 38, 211–218 (1996).
Weeks, A., Turelli, M., Harcombe, W., Reynolds, K. & Hoffmann, A. From parasite to mutualist: rapid evolution of Wolbachia in natural populations of Drosophila. PLoS Biol. 5, e114 (2007).
Sachs, J., Skophammer, R., Bansal, N. & Stajich, J. Evolutionary origins and diversification of proteobacterial mutualists. Proc. R. Soc. B Biol. Sci. Sci. 281, 20132146 (2014).
Hembry, David H, Jeremy B Yoder, and Kari Roesch Goodman. 2014. “Coevolution and the Diversification of Life.” The American Naturalist 184 (4): 425–38. https://doi.org/10.1086/677928.
van der Niet, T., and S. D. Johnson. 2012. Phylogenetic evidence for pollinator-driven diversification of angiosperms. Trends in Ecology and Evolution 27:353–361.
Pellmyr O. Yuccas, yucca moths, and coevolution: a review. Ann Mo Bot Gard. 2003;90:35–55.
Moe, A. M., and G. D. Weiblen. 2012. Pollinator-mediated reproductive isolation among dioecious fig species (Ficus, Moraceae). Evolution 66:3710–3721.
Althoff, D. M., K. A. Segraves, C. I. Smith, J. Leebens-Mack, and O. Pellmyr. 2012. Geographic isolation trumps coevolution as a driver of yucca and yucca moth diversification. Molecular Phylogenetics and Evolution 62:898–906.
Smith, C. I., O. Pellmyr, D. M. Althoff, M. Balcázar-Lara, J. Leebens-Mack, and K. A. Segraves. 2008. Pattern and timing of diversification in Yucca (Agavaceae): specialized pollination does not elevate rates of diversification. Proceedings of the Royal Society B: Biological Sciences 275:249–258.