Winter_2024_Bis2A_Facciotti_Reading_24
- Page ID
- 122804
Learning Objectives Associated with Winter_2024_Bis2A_Facciotti_Reading_24GI.31 Define, describe and correctly use the terms: point mutation, deletion, insertion, nonsense, frameshift, null, loss-of-function and gain-of-function. Be able to describe their possible impact(s) on protein integrity. GI.33 Use a codon table and your knowledge of protein structure and function to make predictions of how changes at the DNA level might influence protein structure and function. GI.34 Explain the possible different mechanisms by which mutations can cause changes in phenotype. Include mutations to both protein-coding regions and non protein-coding regions in your discussion. GI.35 Explain the potential influence of mutations on the specificity and affinity of protein-DNA interactions and the potential impact of these mutations on gene expression. GI.53 Define and correctly use the terms "haploid", "diploid", and "polyploid" and describe some of the costs and benefits of polyploidy. GI.54 Explain how multiple genes may interact to influence phenotype and apply this concept to interpret or propose a molecular mechanism that explains the phenotypes. GI.55 Explain how multiple alleles for a single gene, when encoded in a genome, can result in multiple distinguishable phenotypes. Propose or interpret a molecular mechanism that explains these multiple phenotypes. GI.56 Define and be able to correctly use the terms heterozygous, homozygous, mutant, wild type, dominant, recessive, allele, gene, loci, and chromosomes. GI.57 Predict a phenotype(s) if given a genotype (for each haploid, diploid and polyploid) and related molecular mechanisms, and vice versa. |
Mutations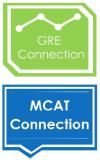
Errors occurring during DNA replication are not the only way by which mutations can arise in DNA. Mutations, variations in the nucleotide sequence of a genome, can also occur because of physical damage to DNA. Such mutations may be of two types: induced or spontaneous. Induced mutations are those that result from an exposure to chemicals, UV rays, x-rays, or some other environmental agent. Spontaneous mutations occur with no exposure to any environmental agent; they result from spontaneous biochemical reactions taking place within the cell.
Mutations may have a wide range of effects. Some mutations create no observable impact; we call these silent mutations. Point mutations are those mutations that affect a single base pair. The most common nucleotide mutations are substitutions, in which one base is replaced by another. These can be of two types, either transitions or transversions. Transition substitution refers to a purine or pyrimidine being replaced by a base of the same kind; for example, a purine such as adenine may be replaced by the purine guanine. Transversion substitution refers to a purine being replaced by a pyrimidine, or vice versa; for example, cytosine, a pyrimidine, is replaced by adenine, a purine. Mutations can also be the result of the addition of a nucleotide, known as an insertion, or the removal of a base, also known as deletion. Sometimes a piece of DNA from one chromosome may get translocated to another chromosome or to another region of the same chromosome; this is known as translocation.
As we will visit later, when a mutation occurs in a protein coding region, it may have several effects. Transition or transversion mutants may lead to no change in the protein sequence (known as silent mutations), change the amino acid sequence (known as missense mutations), or create what is known as a stop codon (known as a nonsense mutation). Insertions and deletions in protein coding sequences lead to frameshift mutations. Missense mutations that lead to conservative changes results in the substitution of similar but not identical amino acids. For example, the acidic amino acid glutamate being substituted for the acidic amino acid aspartate would be considered conservative. We do not expect these types of missense mutations to be as severe as a non-conservative amino acid change; such as a glutamate substituted for a valine. Drawing from our understanding of functional group chemistry, we can correctly infer that this type of substitution may lead to severe functional consequences, depending upon location of the mutation.
Note: Vocabulary Watch
Note that the preceding paragraph had a lot of potentially new vocabulary - it would be a good idea to learn these terms.
Figure 1. Mutations can lead to changes in the protein sequence encoded by the DNA.
Mutations: Some nomenclature and considerations
Mutation
Etymologically, the term mutation means a change or alteration. In genetics, a mutation is a change in the genetic material - DNA sequence - of an organism. By extension, a mutant is the organism in which a mutation has occurred. But what is the change compared to? The answer to this question is that it depends. We can make the comparison with the direct progenitor (cell or organism) or to patterns seen in a population of the organism in question. It mostly depends on the specific context of the discussion. Since genetic studies often look at a population (or key subpopulations) of individuals, we begin by describing the term "wild-type".
Wild Type vs Mutant
What do we mean by "wild type"? Since the definition can depend on context, this concept is not entirely straightforward. Here are a few examples of definitions you may run into:
Possible meanings of "wild-type"
- An organism having an appearance characteristic of the species in a natural breeding population (i.e. a cheetah's spots and tear-like dark streaks that extend from the eyes to the mouth).
- The form or forms of a gene most commonly occurring in nature in a given species.
- A phenotype, genotype, or gene that predominates in a natural population of organisms or strain of organisms in contrast to that of natural or laboratory mutant forms.
- The normal, as opposed to the mutant gene or allele.
The common thread to all of the definitions listed above is based on the "norm" for a set of characteristics with respect to a specific trait compared to the overall population. In the "Pre-DNA sequencing Age" species were classified based on common phenotypes (what they looked like, where they lived, how they behaved, etc.). A "norm" was established for the species in question. For example, Crows display a common set of characteristics, they are large, black birds that live in specific regions, eat certain types of food and behave in a certain characteristic way. If we see one, we know its a crow based on these characteristics. If we saw one with a white head, we would think that either it is a different bird (not a crow) or a mutant, a crow that has some alteration from the norm or wild type.
In this class we take what is common about those varying definitions and adopt the idea that "wild type" is simply a reference standard against which we can compare members of a population.
Possible NB Discussion Point
If you were assigning wild type traits to describe a dog, what would they be? What is the difference between a mutant trait and variation of a trait in a population of dogs? Is there a wild type for a dog that we could use as a standard? How would we begin to think about this concept with respect to dogs?
Figure 2. Mutations can lead to changes in the protein sequence encoded by the DNA that then impact the outward appearance of the organism.
(Source)
Mutations are simply changes from the "wild type", reference or parental sequence for an organism. While the term "mutation" has colloquially negative connotations, we must remember that change is neither inherently "bad". Mutations (changes in sequences) should not primarily be thought of as "bad" or "good", but rather simply as changes and a source of genetic and phenotypic diversity on which evolution by natural selection can occur. Natural selection ultimately determines the long-term fate of mutations. If the mutation confers a selective advantage to the organism, the mutation will be selected and may eventually become very common in the population. Conversely, if the mutation is deleterious, natural selection will ensure that the mutation will be lost from the population. If the mutation is neutral, that is it neither provides a selective advantage or disadvantage, then it may persist in the population. Different forms of a gene, including those associated with "wild type" and respective mutants, in a population are termed alleles.
Consequences of Mutations
For an individual, the consequence of mutations may mean little or it may mean life or death. Some deleterious mutations are null or knock-out mutations which result in a loss of function of the gene product. These mutations can arise by a deletion of the either the entire gene, a portion of the gene, or by a point mutation in a critical region of the gene that renders the gene product non-functional. These types of mutations are also referred to as loss-of-function mutations. Alternatively, mutations may lead to a modification of an existing function (i.e. the mutation may change the catalytic efficiency of an enzyme, a change in substrate specificity, or a change in structure). In rare cases a mutation may create a new or enhanced function for a gene product; this is often referred to as a gain-of-function mutation. Lastly, mutations may occur in non-coding regions of DNA. These mutations can have a variety of outcomes including altered regulation of gene expression, changes in replication rates or structural properties of DNA and other non-protein associated factors.
Mutations and cancer
Mutations can affect either somatic cells or germ cells. Sometimes mutations occur in DNA repair genes, in effect compromising the cell's ability to fix other mutations that may arise. If, as a result of mutations in DNA repair genes, many mutations accumulate in a somatic cell, they may lead to problems such as the uncontrolled cell division observed in cancer. Cancers, including forms of pancreatic cancer, colon cancer, and colorectal cancer have been associated with mutations like these in DNA repair genes. If, by contrast, a mutation in DNA repair occurs in germ cells (sex cells), the mutation will be passed on to the next generation, as in the case of diseases like hemophilia and xeroderma pigmentosa. In the case of xeroderma pigmentoas individuals with compromised DNA repair processes become very sensitive to UV radiation. In severe cases these individuals may get severe sun burns with just minutes of exposure to the sun. Nearly half of all children with this condition develop their first skin cancers by age 10.
Consequences of errors in replication, transcription and translation
Something key to think about:
Cells have evolved a variety of ways to make sure DNA errors are both detected and corrected, rom proof reading by the various DNA-dependent DNA polymerases, to more complex repair systems. Why did so many mechanisms evolve to repair errors in DNA? By contrast, similar proof-reading mechanisms did NOT evolve for errors in transcription or translation. Why might this be? What would be the consequences of an error in transcription? Would such an error effect the offspring? Would it be lethal to the cell? What about translation? Ask the same questions about the process of translation. What would happen if the wrong amino acid was accidentally put into the growing polypeptide during the translation of a protein? Contrast this with DNA replication.
Mutations as instruments of change
Mutations are how populations can adapt to changing environmental pressures
Mutations are randomly created in the genome of every organism, and this in turn creates genetic diversity and a plethora of different alleles per gene per organism in every population on the planet. If mutations did not occur, and chromosomes were replicated and transmitted with 100% fidelity, how would cells and organisms adapt? Whether evolution keeps mutations in a population depends on whether the mutation provides selective advantage, poses some selective cost or is at the very least, not harmful. Indeed, mutations that appear neutral may persist in the population for many generations and only be meaningful when a population is challenged with a new environmental challenge. At this point, the apparently previously neutral mutations may provide a selective advantage.
Example: Antibiotic resistance
The bacterium E. coli is sensitive to an antibiotic called streptomycin, which inhibits protein synthesis by binding to the ribosome. The ribosomal protein L12 can be mutated such that streptomycin no longer binds to the ribosome and inhibits protein synthesis. Wild type and L12 mutants grow equally well and the mutation appears to be neutral in the absence of the antibiotic. In the presence of the antibiotic wild type cells die and L12 mutants survive. This example shows how genetic diversity is important for the population to survive. If mutations did not randomly occur, when the population is challenged by an environmental event, such as the exposure to streptomycin, the entire population would die. For most populations this becomes a numbers game. If the mutation rate is 10-6 then a population of 107 cells would have 10 mutants; a population of 108 would have 100 mutants, etc.
Uncorrected errors in DNA replication lead to mutation. In this example, an uncorrected error was passed onto a bacterial daughter cell. This error is in a gene that encodes for part of the ribosome. The mutation results in a different final 3D structure of the ribosome protein. While the wildtype ribosome can bind to streptomycin (an antibiotic that will kill the bacterial cell by inhibiting the ribosome function) the mutant ribosome cannot bind to streptomycin. This bacteria is now resistant to streptomycin. Source: Bis2A Team original image
An example: Lactate dehydrogenase
Lactate Dehydrogenase (LDH), the enzyme that catalyzes the reduction of pyruvate into lactic acid in fermentation, while virtually every organism has this activity, the corresponding enzyme and therefore gene differs immensely between humans and bacteria. The proteins are clearly related, they perform the same basic function but have a variety of differences, from substrate binding affinities and reaction rates to optimal salt and pH requirements. Each of these attributes have been evolutionarily tuned for each specific organism through multiple rounds of mutation and selection.
Possible NB Discussion Point
We can use comparative DNA sequence analysis to generate hypotheses about the evolutionary relationships between three or more organisms. One way to accomplish this is to compare the DNA or protein sequences of proteins found in each of the organisms we wish to compare. Let us, for example, imagine that we were to compare the sequences of lactate dehydrogenase (LDH) from three different organisms. The schematic below depicts the primary structures of LDH proteins from Organisms A, B, and C. The letters in the center of the proteins' line diagram represent amino acids at a unique position and the proposed differences in each sequences (Attribution: Marc T. Facciotti [original work]). Question: Is Organism C more closely related to Organism A or B? The simplest explanation is that Organism A is the earliest form, a mutation occurred giving rise to Organism B. Over time a second mutation arose in the B lineage to give rise to the enzyme found in Organism C. This is the simplest explanation, however we cannot rule out other possibilities. Can you think of other ways the different forms of the LDH enzyme arose these three organisms?
Real-life Application:
As we have seen in the "Mutations and Mutants" module, changing even one nucleotide can have major effects on the translated product. Read more about an undergraduate's work on point mutations and GMOs here.
POST PRACTICE GUIDE
General Practice
3. Why: Reading a figure that depicts steps in a metabolic pathway is a key skill that you need in future courses on cellular metabolism and anywhere else you might encounter metabolic pathways. Here we use and practice that skill - and thrown in a little wrinkle of thinking about the genes that encode the enzymes in the pathway while linking our hard-earned lessons on metabolism, enzymes, learning to read pathways, getting comfortable with molecular structures and the funny names of enzymes and compounds etc. to phenotype.
How to practice: Look at the figure below: This is the same figure we discussed in class with a couple of additions. Create and “energy story” for these reactions.
This exercise helps you practice learning objectives: This is “reloading” learning objectives from our section on metabolism that expect you to read a metabolic pathway. Here we add one more layer, which is to recognize the gene name that encodes the enzyme catalyzing a step in the pathway (in this figure ade5 and ade2) and to add this into your energy story.
4. Why: One of the big pictures objectives we instructors have for you is to start being able to tie together concepts and ideas from across the course. In this case we want you to be able to talk about the molecular (in this case can be gene and enzyme level) details that can explain a simple phenotype of an organism.
How to practice: Use the figure above to explain the molecular basis for the phenotype associated with a haploid genotype in which the ade2 gene has been deleted.
This exercise helps you practice learning objectives: GI.57 Predict a phenotype(s) if given a genotype (for each haploid, diploid and polyploid) and related molecular mechanisms, and vice versa.
5. Why: Mutations are the engine of organismal diversity required for natural selection. Here we want you to link the idea of mutation to different phenotype and to start linking the idea of a change in the genome and how that can mechanistically cause differences in phenotype. We want you to practice the ideas above but now get you to think about how mutations factor in to the story of the genotype-phenotype relationship.
How to practice: Explain the possible outcomes of mutations (nucleotide changes) in the region of DNA encoding the ade2 gene. What are the possible effects on the protein encoded by the gene? Can you propose a hypothetical scenario that might explain HOW a change in the ade2 gene leads to an amino-acid-level change in the protein that is responsible for a “novel” phenotype. (suggestion: think about the previous questions probing molecular interactions with the ribosome).
This exercise helps you practice learning objectives: GI.34 Explain the possible different mechanisms by which mutations can cause changes in phenotype. Include mutations to both protein-coding regions and non protein-coding regions in your discussion.; GI.57 Predict a phenotype(s) if given a genotype (for each haploid, diploid and polyploid) and related molecular mechanisms, and vice versa.; GI.54 Explain how multiple genes may interact to influence phenotype and apply this concept to interpret or propose a molecular mechanism that explains the phenotypes.; GI.55 Explain how multiple alleles for a single gene, when encoded in a genome, can result in multiple distinguishable phenotypes. Propose or interpret a molecular mechanism that explains these multiple phenotypes.
6. Why: What happens to phenotype when you can have multiple copies of a gene in a genome and those copies are different versions (mutants/alleles) of one another? In Bis2a we want to you start thinking about this with the very simplest starting assumptions (which you will see in later classes do not hold true in all cases). This exercise is basically asking you to take the concepts/skills you worked on in exercises 4 and 5 and to combine them in a scenario where multiple variants of a gene can co-exist in the same cell.
How to practice: Imagine the following partially hypothetical scenario: A typical sized yeast cell (haploid or diploid) requires a minimum of 100 units of enzyme activity from the protein encoded by the ade2 gene in order to keep a white phenotype when the purine biosynthetic pathway is operating. The wild-type ade2 allele produces 100 units of activity. A haploid yeast expresses an allele of ade2 (we’ll call it ade2-a) that encodes an enzyme that provides 50 units of activity. Create figures/cartoons to help you keep track of what is going on!!
Predict the phenotype and explain the molecular details of:
(a) a haploid cell expressing a single copy of ade2-a
(b) a haploid cell expressing a single copy of the ade2 gene
(c) a heterozygous diploid cell expressing a single copy of ade2-a allele and a deletion of the wild-type ade2 allele gene on the homologous chromosome
(d) a heterozygous diploid cell expressing a single copy of the ade2 allele and and a deletion of the wild-type ade2 allele gene on the homologous chromosome
(e) a homozygous diploid cell expressing two copies of the ade2-a allele
This exercise helps you practice learning objectives: GI.56 Define and be able to correctly use the terms heterozygous, homozygous, mutant, wild type, dominant, recessive, allele, gene, loci, and chromosomes.; GI.53 Define and correctly use the terms "haploid", "diploid", and "polyploid" and describe some of the costs and benefits of polyploidy.; GI.57 Predict a phenotype(s) if given a genotype (for each haploid, diploid and polyploid) and related molecular mechanisms, and vice versa.; GI.54 Explain how multiple genes may interact to influence phenotype and apply this concept to interpret or propose a molecular mechanism that explains the phenotypes.
7. Why: Here we want you to think about the same concepts as in exercise 6 but with a different example.
How to practice: The allele pool in a population can be large. Using the same logic as in exercise 6 propose a reasonable hypothesis for the phenotype of the following:
(a) haploid cells encoding an ade-z allele (the ade-z allele encodes and enzyme that produces 20 units of activity).
(b) haploid cells encoding an ade-x allele (the ade-x allele encodes and enzyme that produces 40 units of activity).
(c) a heterozygous diploid expressing the ade-z and ade-x alleles.
(d) a homozygous dipoid expressing the ade-z allele
(e) a homozygous dipoid expressing the ade-x allel
This exercise helps you practice learning objectives: GI.56 Define and be able to correctly use the terms heterozygous, homozygous, mutant, wild type, dominant, recessive, allele, gene, loci, and chromosomes.; GI.53 Define and correctly use the terms "haploid", "diploid", and "polyploid" and describe some of the costs and benefits of polyploidy.; GI.57 Predict a phenotype(s) if given a genotype (for each haploid, diploid and polyploid) and related molecular mechanisms, and vice versa.; GI.54 Explain how multiple genes may interact to influence phenotype and apply this concept to interpret or propose a molecular mechanism that explains the phenotypes.
8. Why: This is related to the questions in class related to the alleles of the blue6 gene. More practice on genotype-to-phenotype relationship from a different angle. Same skills/different look.
How to practice: Given the following allele pool, propose a reasonable hypothesis for the following scenarios. You may consider various factors including ploidy.
Allele |
Activity at 30°C |
blue6-1 |
25 |
blue6-2 |
45 |
blue6-3 |
100 |
blue6-4 |
65 |
blue6-5 |
80 |
blue6-6 |
20 |
Propose hypotheses that explain phenotype (a). Propose one or more hypotheses that explain the observations about the two phenotypes for (b). Propose hypotheses that explain phenotype (c).
The Blue6 and the Ade2 gene examples have been idealized a little bit for the convenience of talking about interactions between different alleles for the same locus. What are some key assumptions that we make when we treat the total activity of multiple alleles as simply additive?
This exercise helps you practice learning objectives: GI.56 Define and be able to correctly use the terms heterozygous, homozygous, mutant, wild type, dominant, recessive, allele, gene, loci, and chromosomes.; GI.53 Define and correctly use the terms "haploid", "diploid", and "polyploid" and describe some of the costs and benefits of polyploidy.; GI.57 Predict a phenotype(s) if given a genotype (for each haploid, diploid and polyploid) and related molecular mechanisms, and vice versa.; GI.54 Explain how multiple genes may interact to influence phenotype and apply this concept to interpret or propose a molecular mechanism that explains the phenotypes.
9. Why: As we have seen in several cases many processes in biological systems are interconnected in various ways and all of these interconnected processes are regulated either positively or negatively at various points. The interconnections between functions, pathways, compounds, you name it in the cell are often drawn in network diagram form where positive and negative regulation is depicted by special symbols. Here we ask you to practice reading these kinds of figures.
How to practice: There are common symbols in regulatory pathways to indicate positive and negative regulatory circuits. Identify some of the positive and negative circuits in the image below. Then, select a specific protein and pretend that a mutation has been generated in the gene encoding it. What would be the impact of the mutation on the phenotype (apoptosis)?
This exercise helps you practice learning objectives: GI.47 Using a regulatory network, accurately interpret relationships between genes/proteins in the network and predict potential targets of regulation by different possible types of signaling molecules.; GI.44 Given some specific constraints on a regulatory circuit, predict the behavior of the circuit under the possible influence of environmental or genetic perturbations (changes).
10. Why: Translation is a core process in all cells. We practiced some skills related to this process in previous study guides but want to keep exercising the skills associated with the reading of DNA sequences and their translation. Keeping it fresh on the mind!
How to practice: Consider the following hypothetical sequence:
5’CGCTACGTCTTACGCTGGAGCTCTCATGGATCGGTTCGGTAGGGCTCGATCACATCGCTAGCCAT 3’
3’GCGATGCAGAATGCGACCTCGAGAGTACCTAGCCAAGCCATCCCGAGCTAGTGTAGCGATCGGTA 5’
a) How many potential open reading frames (pairs of start and stop codons) are present in this DNA fragment (you may need to look up a codon table on-line)?
b) Look at only the top strand. Identify the location(s) of the start codons and the stop codons. Which frame could encode a potential protein?
c) Look at only the bottom strand. Identify the location(s) of the start codons and the stop codons. Which frame could encode a potential protein? (Remember that mRNAs are read by the ribosome 5’ to 3.’)
d) Take the ORFs identified in (b) and (c) and use this information to obtain a possible protein sequence. Which ORF makes the longest protein?
This exercise helps you practice learning objectives: This is an exercise to help you keep practicing learning objectives from the translation section.
Practice Exam Questions
Question Q24.1.
Q24.1 In the reaction depicted in the figure below the most likely phenotype of a Δade5 genotype expressed in a haploid yeast is:
A. cells turn red
B. cells will be cream colored
C. cells will be pink
D. cells will turn blue
E. cells will turn purple
Question Q24.2.
Q24.2. The figure to the right diagrams a metabolic pathway that might controls the color of flowers in certain plants: The genotype of a diploid wild-type flower is AA BB CC DD, and its phenotype is light purple. Alleles A, B, C, D each encoded enzymes that catalyze the eponymous reactions in the Figure. a, b, c, and d are loss-of-function alleles. What will the mostly likely phenotype of the AA Bb cc DD double mutant?
A. Purple
B. White
C. Orange
D. Red
E. Yellow
Question Q24.3. Phenotype/genotype with alleles and chromosomal location.
Q24.3. Within a group of mutants (genetically related organisms with slightly different genotypes) with the same overt phenotype, DNA sequencing studies mapping the location of the mutations determined that mutations in different organisms were on different chromosomes. This indicates that
A. the same gene governs all the steps in a particular biological pathway.
B. different genes can govern different individual steps in the same biological pathway.
C. different genes govern the same step in a particular biological pathway.
D. all biological pathways are governed by different genes.
E. genes do not govern steps in biological pathways.
Question Q24.4. A bit of practice reading and interpreting networks.
Q24.4 In the network below we see the functional relationships network of transcription factors, the genes encoding them, environmental factors and the genes responsible for a complex bacterial phenotype called heterocyst formation (hgl, hep, and nif). Which interpretation of the figure is most reasonable?
A. Calcium negatively regulates CcbP.
B. The expression of gene patS leads to an increase in the expression of hgl.
C. Nitrogen limitation “turns-on” hgl expression.
D.The NtcA transcription factor can act both negatively and positively.
E. C & D
Question Q24.5. Relatively easy question if you understand the definition of allele and diploid genomes.
Q24.5. Within the human population, 200 different alleles exist for a specific locus. Each person carries at most __________ alleles for this locus.
A. 1
B. 2
C. 100
D. 200
E. 400
GLOSSARY
mutation that results from exposure to chemicals or environmental agents
variation in the nucleotide sequence of a genome
type of repair mechanism in which mismatched bases are removed after replication
type of DNA repair mechanism in which the wrong base, along with a few nucleotides upstream or downstream, are removed
function of DNA pol in which it reads the newly added base before adding the next one
mutation that affects a single base
mutation that is not expressed
mutation that takes place in the cells as a result of chemical reactions taking place naturally without exposure to any external agent
when a purine is replaced with a purine or a pyrimidine is replaced with another pyrimidine
when a purine is replaced by a pyrimidine or a pyrimidine is replaced by a purine
- induced mutation:
- mutation:
- mismatch repair:
- nucleotide excision repair:
- proofreading:
- point mutation:
- silent mutation:
- spontaneous mutation:
- transition substitution:
- transversion substitution: