Winter_2024_Bis2A_Facciotti_Reading_13
- Page ID
- 122711
\( \newcommand{\vecs}[1]{\overset { \scriptstyle \rightharpoonup} {\mathbf{#1}} } \)
\( \newcommand{\vecd}[1]{\overset{-\!-\!\rightharpoonup}{\vphantom{a}\smash {#1}}} \)
\( \newcommand{\id}{\mathrm{id}}\) \( \newcommand{\Span}{\mathrm{span}}\)
( \newcommand{\kernel}{\mathrm{null}\,}\) \( \newcommand{\range}{\mathrm{range}\,}\)
\( \newcommand{\RealPart}{\mathrm{Re}}\) \( \newcommand{\ImaginaryPart}{\mathrm{Im}}\)
\( \newcommand{\Argument}{\mathrm{Arg}}\) \( \newcommand{\norm}[1]{\| #1 \|}\)
\( \newcommand{\inner}[2]{\langle #1, #2 \rangle}\)
\( \newcommand{\Span}{\mathrm{span}}\)
\( \newcommand{\id}{\mathrm{id}}\)
\( \newcommand{\Span}{\mathrm{span}}\)
\( \newcommand{\kernel}{\mathrm{null}\,}\)
\( \newcommand{\range}{\mathrm{range}\,}\)
\( \newcommand{\RealPart}{\mathrm{Re}}\)
\( \newcommand{\ImaginaryPart}{\mathrm{Im}}\)
\( \newcommand{\Argument}{\mathrm{Arg}}\)
\( \newcommand{\norm}[1]{\| #1 \|}\)
\( \newcommand{\inner}[2]{\langle #1, #2 \rangle}\)
\( \newcommand{\Span}{\mathrm{span}}\) \( \newcommand{\AA}{\unicode[.8,0]{x212B}}\)
\( \newcommand{\vectorA}[1]{\vec{#1}} % arrow\)
\( \newcommand{\vectorAt}[1]{\vec{\text{#1}}} % arrow\)
\( \newcommand{\vectorB}[1]{\overset { \scriptstyle \rightharpoonup} {\mathbf{#1}} } \)
\( \newcommand{\vectorC}[1]{\textbf{#1}} \)
\( \newcommand{\vectorD}[1]{\overrightarrow{#1}} \)
\( \newcommand{\vectorDt}[1]{\overrightarrow{\text{#1}}} \)
\( \newcommand{\vectE}[1]{\overset{-\!-\!\rightharpoonup}{\vphantom{a}\smash{\mathbf {#1}}}} \)
\( \newcommand{\vecs}[1]{\overset { \scriptstyle \rightharpoonup} {\mathbf{#1}} } \)
\( \newcommand{\vecd}[1]{\overset{-\!-\!\rightharpoonup}{\vphantom{a}\smash {#1}}} \)
Learning Objectives Associated with Winter_2024_Bis2A_Facciotti_Reading_13ME.16 Apply the concept of the “conservation of mass” to metabolism by describing the different forms mass takes as it enters and leaves the cell (e.g. input: reduced molecules like glucose, lipids, proteins, etc. & output: oxidized molecules like CO2, H2O etc.). ME.15 Apply the concept of the "conservation of energy" to central metabolism. Follow energy from "sources" of electrons with relatively low reduction potentials to "sinks" with higher redox potentials, describe the major transfers of energy and how this energy is "stored" at each stage. ————-Learning objectives above are for the full course, but particularly relevant to the discussion of metabolism and thus extend well beyond this lecture——————- ME.11 Create an energy story explaining the functional value of pyruvate oxidation and its importance in generating building blocks for biomolecules. ME.8 Create an “energy story” explaining the functional value of pyruvate reduction (fermentation) to a cell and its importance in regenerating the NAD+ pool. ME.14 Given metabolic maps of glycolysis and TCA cycle, follow the flow of electrons from energy source to mobile carrier NADH and use a provided redox tower to quantitatively describe the energy transformations. ME.12 Create an energy story for each reaction in the TCA cycle. ME.10 Explain the central role of Acetyl CoA in carbon metabolism including its formation from various sources (the oxidative decarboxylation of pyruvate, oxidation of fatty acids, and/or oxidative degradation of some amino acids) and as a carbon source in both the TCA cycle and lipid synthesis. ME.13 Describe and give examples of cyclic processes and how they are involved in energy harvesting and the inter-conversion of molecule types. ME.18 Relate Coenzyme A's ability to form thioesters with the concepts of its function of carrying "activated" acyl groups. ME.19 Identify Coenzyme A and its functionally important thiol group by inspection of a molecular model. ME.9 Discuss at least two key fates for glucose other than its direct oxidation to pyruvate, highlighting the role of glycolytic intermediates in processes related to carbon flow (functions that are not associated with energy harvesting). ME.91 Explain the role of glucose in the creation of medium- and long-term energy storage compounds. |
Fermentation and regeneration of NAD+
Section summary
This section discusses the process of fermentation. Due to the heavy emphasis in this course on central carbon metabolism, the discussion of fermentation understandably focuses on the fermentation of pyruvate. Nevertheless, some of the core principles that we cover in this section apply equally well to the fermentation of many other small molecules.
The "purpose" of fermentation
The oxidation of a variety of small organic compounds is a process that is utilized by many organisms to garner energy for cellular maintenance and growth. The oxidation of glucose via glycolysis is one such pathway. Several key steps in the oxidation of glucose to pyruvate involve the reduction of the electron/energy shuttle NAD+ to NADH. You were already asked to figure out what options the cell might reasonably have to reoxidize the NADH to NAD+ in order to avoid consuming the available pools of NAD+ and to thus avoid stopping glycolysis. Put differently, during glycolysis, cells can generate large amounts of NADH and slowly exhaust their supplies of NAD+. If glycolysis is to continue, the cell must find a way to regenerate NAD+, either by synthesis or by some form of recycling.
In the absence of any other process—that is, if we consider glycolysis alone—it is not immediately obvious what the cell might do. One choice is to try putting the electrons that were once stripped off of the glucose derivatives right back onto the downstream product, pyruvate, or one of its derivatives. We can generalize the process by describing it as the returning of electrons to the molecule that they were once removed, usually to restore pools of an oxidizing agent. This, in short, is fermentation. As we will discuss in a different section, the process of respiration can also regenerate the pools of NAD+ from NADH. Cells lacking respiratory chains or in conditions where using the respiratory chain is unfavorable may choose fermentation as an alternative mechanism for garnering energy from small molecules.
An example: lactic acid fermentation
An everyday example of a fermentation reaction is the reduction of pyruvate to lactate by the lactic acid fermentation reaction. This reaction should be familiar to you: it occurs in our muscles when we exert ourselves during exercise. When we exert ourselves, our muscles require large amounts of ATP to perform the work we are demanding of them. As the ATP is consumed, the muscle cells are unable to keep up with the demand for respiration, O2 becomes limiting, and NADH accumulates. Cells need to get rid of the excess and regenerate NAD+, so pyruvate serves as an electron acceptor, generating lactate and oxidizing NADH to NAD+. Many bacteria use this pathway as a way to complete the NADH/NAD+ cycle. You may be familiar with this process from products like sauerkraut and yogurt. The chemical reaction of lactic acid fermentation is the following:
Pyruvate + NADH ↔ lactic acid + NAD+
Figure 1. Lactic acid fermentation converts pyruvate (a slightly oxidized carbon compound) to lactic acid. In the process, NADH is oxidized to form NAD+. Attribution: Marc T. Facciotti (original work)
Energy story for the fermentation of pyruvate to lactate
An example (if a bit lengthy) energy story for lactic acid fermentation is the following:
The reactants are pyruvate, NADH, and a proton. The products are lactate and NAD+. The process of fermentation results in the reduction of pyruvate to form lactic acid and the oxidation of NADH to form NAD+. Electrons from NADH and a proton are used to reduce pyruvate into lactate. If we examine a table of standard reduction potential, we see under standard conditions that a transfer of electrons from NADH to pyruvate to form lactate is exergonic and thus thermodynamically spontaneous. The reduction and oxidation steps of the reaction are coupled and catalyzed by the enzyme lactate dehydrogenase.
A second example: alcohol fermentation
Another familiar fermentation process is alcohol fermentation, which produces ethanol, an alcohol. The alcohol fermentation reaction is the following:
Figure 2. Ethanol fermentation is a two-step process. Pyruvate (pyruvic acid) is first converted into carbon dioxide and acetaldehyde. The second step converts acetaldehyde to ethanol and oxidizes NADH to NAD+. Attribution: Marc T. Facciotti (original work)
In the first reaction, a carboxyl group is removed from pyruvic acid, releasing carbon dioxide as a gas (some of you may be familiar with this as a key component of various beverages). The second reaction removes electrons from NADH, forming NAD+ and producing ethanol (another familiar compound—usually in the same beverage) from the acetaldehyde, which accepts the electrons.
Fermentation pathways are numerous
While the lactic acid fermentation and alcohol fermentation pathways described above are examples, there are many more reactions (too numerous to go over) that Nature has evolved to complete the NADH/NAD+ cycle. It is important that you understand the general concepts behind these reactions. In general, cells try to maintain a balance or constant ratio between NADH and NAD+; when this ratio becomes unbalanced, the cell compensates by modulating other reactions to compensate. The only requirement for a fermentation reaction is that it uses a small organic compound as an electron acceptor for NADH and regenerates NAD+. Other familiar fermentation reactions include ethanol fermentation (as in beer and bread), propionic fermentation (it's what makes the holes in Swiss cheese), and malolactic fermentation (it's what gives Chardonnay its more mellow flavor—the more conversion of malate to lactate, the softer the wine). In Figure 3, you can see a large variety of fermentation reactions that various bacteria use to reoxidize NADH to NAD+. All of these reactions start with pyruvate or a derivative of pyruvate metabolism, such as oxaloacetate or formate. Pyruvate is produced from the oxidation of sugars (glucose or ribose) or other small, reduced organic molecules. It should also be noted that other compounds can be used as fermentation substrates besides pyruvate and its derivatives. These include methane fermentation, sulfide fermentation, or the fermentation of nitrogenous compounds such as amino acids. You are not expected to memorize all of these pathways. You are, however, expected to recognize a pathway that returns electrons to products of the compounds that were originally oxidized to recycle the NAD+/NADH pool and to associate that process with fermentation.
Figure 3. This figure shows various fermentation pathways using pyruvate as the initial substrate. In the figure, pyruvate is reduced to a variety of products via different and sometimes multistep (dashed arrows represent possible multistep processes) reactions. All details are deliberately not shown. The key point is to appreciate that fermentation is a broad term not solely associated with the conversion of pyruvate to lactic acid or ethanol. Source: Marc T. Facciotti (original work)
A note on the link between substrate-level phosphorylation and fermentation
Fermentation occurs in the absence of molecular oxygen (O2). It is an anaerobic process. Notice there is no O2 in any of the fermentation reactions shown above. Many of these reactions are quite ancient, hypothesized to be some of the first energy-generating metabolic reactions to evolve. This makes sense if we consider the following:
- The early atmosphere was highly reduced, with little molecular oxygen readily available.
- Small, highly reduced organic molecules were relatively available, arising from a variety of chemical reactions.
- These types of reactions, pathways, and enzymes are found in many different types of organisms, including bacteria, archaea, and eukaryotes, suggesting these are very ancient reactions.
- The process evolved long before O2 was found in the environment.
- The substrates, highly reduced, small organic molecules, like glucose, were readily available.
- The end products of many fermentation reactions are small organic acids, produced by the oxidation of the initial substrate.
- The process is coupled to substrate-level phosphorylation reactions. That is, small, reduced organic molecules are oxidized, and ATP is generated by first a red/ox reaction followed by the substrate-level phosphorylation.
- This suggests that substrate-level phosphorylation and fermentation reactions coevolved.
Consequences of fermentation
Imagine a world where fermentation is the primary mode for extracting energy from small molecules. As populations thrive, they reproduce and consume the abundance of small, reduced organic molecules in the environment, producing acids. One consequence is the acidification (decrease in pH) of the environment, including the internal cellular environment. This can be disruptive, since changes in pH can have a profound influence on the function and interactions among various biomolecules. Therefore, mechanisms needed to evolve that could remove the various acids. Fortunately, in an environment rich in reduced compounds, substrate-level phosphorylation and fermentation can produce large quantities of ATP.
It is hypothesized that this scenario was the beginning of the evolution of the F0F1-ATPase, a molecular machine that hydrolyzes ATP and translocates protons across the membrane (we'll see this again in the next section). With the F0F1-ATPase, the ATP produced from fermentation could now allow for the cell to maintain pH homeostasis by coupling the free energy of hydrolysis of ATP to the transport of protons out of the cell. The downside is that cells are now pumping all of these protons into the environment, which will now start to acidify.
Oxidation of Pyruvate and the TCA Cycle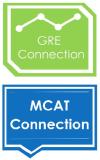
Overview of Pyruvate Metabolism and the TCA Cycle
Under appropriate conditions, pyruvate can be further oxidized. One of the most studied oxidation reactions involving pyruvate is a two-part reaction involving NAD+ and a molecule called co-enzyme A, often abbreviated as "CoA". This reaction oxidizes pyruvate, leads to a loss of one carbon via decarboxylation, and creates a new molecule called acetyl-CoA. The resulting acetyl-CoA can enter several pathways for the biosynthesis of larger molecules or it can flow into another pathway of central metabolism called the Citric Acid Cycle, sometimes also called the Krebs Cycle, or Tricarboxylic Acid (TCA) Cycle. Here, the remaining two carbons in the acetyl group can either be further oxidized or serve again as precursors for the construction of various other molecules. We discuss these scenarios below.
The different fates of pyruvate and other end products of glycolysis
The glycolysis module left off with the end-products of glycolysis: 2 pyruvate molecules, 2 ATPs and 2 NADH molecules. This module and the module on fermentation explore what the cell can do with the pyruvate, ATP and NADH that were generated.
The fates of ATP and NADH
ATP can be used for or coupled to a variety of cellular functions including biosynthesis, transport, replication, etc. We will see many such examples throughout the course.
What to do with the NADH however, depends on the conditions under which the cell is growing. Sometimes, the cell will opt to recycle NADH rapidly back into NAD+. This occurs through a process called fermentation. This process returnsthe electrons initially taken from the glucose derivatives to more downstream products through another red/ox transfer (described in more detail in the module on fermentation). Alternatively, NADH can recycle back into NAD+ by donating electrons to something known as an electron transport chain (we cover this in the module on respiration and electron transport).
The fate of cellular pyruvate
- Pyruvate can be a terminal electron acceptor (either directly or indirectly) in fermentation reactions and we discuss this in the fermentation module.
- Pyruvate can be secreted from the cell as a waste product.
- Pyruvate can be further oxidized to extract more free energy from this fuel.
- Pyruvate can serve as a valuable intermediate compound linking some core carbon processing metabolic pathways
The further oxidation of pyruvate
In respiring bacteria and archaea, the pyruvate is further oxidized in the cytoplasm. In aerobically respiring eukaryotic cells, cells transport the pyruvate molecules produced at the end of glycolysis into mitochondria. These sites of cellular respiration house oxygen consuming electron transport chains (ETC in the module on respiration and electron transport). Organisms from all three domains of life share similar mechanisms to further oxidize the pyruvate to CO2. First pyruvate is decarboxylated and covalently linked to co-enzyme A via a thioester linkage to form the molecule known as acetyl-CoA. While acetyl-CoA can feed into multiple other biochemical pathways, we now consider its role in feeding the circular pathway known as the Tricarboxylic Acid Cycle, also referred to as the TCA cycle, the Citric Acid Cycle or the Krebs Cycle. We detail this process below.
Conversion of Pyruvate into Acetyl-CoA
In a multi-step reaction catalyzed by the enzyme pyruvate dehydrogenase, pyruvate is oxidized by NAD+, decarboxylated, and covalently linked to a molecule of co-enzyme A via a thioester bond. The release of the carbon dioxide is important here, this reaction often results in a loss of mass from the cell as the CO2 will diffuse or be transported out of the cell and become a waste product. In addition, cells reduce one molecule of NAD+ to NADH during this process per molecule of pyruvate oxidized. Remember: there are two pyruvate molecules produced at the end of glycolysis for every molecule of glucose metabolized; thus, if both pyruvate molecules are oxidized to acetyl-CoA two of the original six carbons will have converted to waste.
Figure 1. Upon entering the mitochondrial matrix, a multi-enzyme complex converts pyruvate into acetyl CoA. In the process, carbon dioxide is released and one molecule of NADH is formed.
In the presence of a suitable terminal electron acceptor, acetyl CoA delivers (exchanges a bond) its acetyl group to a four-carbon molecule, oxaloacetate, to form citrate (designated the first compound in the cycle). This cycle is called by different names: the citric acid cycle (for the first intermediate formed—citric acid, or citrate), the TCA cycle (since citric acid or citrate and isocitrate are tricarboxylic acids), and the Krebs cycle, after Hans Krebs, who first identified the steps in the pathway in the 1930s in pigeon flight muscles.
The Tricarboxcylic Acid (TCA) Cycle
In bacteria and archaea reactions in the TCA cycle typically happen in the cytosol. In eukaryotes, the TCA cycle takes place in the matrix of mitochondria. Almost all (but not all) of the enzymes of the TCA cycle are water soluble (not in the membrane), with the single exception of the enzyme succinate dehydrogenase, which is embedded in the inner membrane of the mitochondrion (in eukaryotes). Unlike glycolysis, the TCA cycle is a closed loop: the last part of the pathway regenerates the compound used in the first step. The eight steps of the cycle are a series of red/ox, dehydration, hydration, and decarboxylation reactions that produce two carbon dioxide molecules, one ATP, and reduced forms of NADH and FADH2.
Figure 2. In the TCA cycle, the acetyl group from acetyl CoA attaches to a four-carbon oxaloacetate molecule to form a six-carbon citrate molecule. Through a series of steps, citrate is oxidized, releasing two carbon dioxide molecules for each acetyl group fed into the cycle. In the process, three NAD+ molecules are reduced to NADH, one FAD+ molecule is reduced to FADH2, and one ATP or GTP (depending on the cell type) is produced (by substrate-level phosphorylation). Because the final product of the TCA cycle is also the first reactant, the cycle runs continuously in the presence of sufficient reactants. Attribution: “Yikrazuul”/Wikimedia Commons (modified)
NOTE:
We are explicitly referring to eukaryotes, bacteria and archaea when we discuss the location of the TCA cycle because many beginning students of biology only associate the TCA cycle with mitochondria. Yes, the TCA cycle occurs in the mitochondria of eukaryotic cells. However, this pathway is not exclusive to eukaryotes; it occurs in bacteria and archaea too!
Steps in the TCA Cycle
Step 1:
The first step of the cycle is a condensation reaction involving the two-carbon acetyl group of acetyl-CoA with one four-carbon molecule of oxaloacetate. The products of this reaction are the six-carbon molecule citrate and free co-enzyme A. This step is considered irreversible because it is so highly exergonic. ATP concentration controls the rate of this reaction through negative feedback. If ATP levels increase, the rate of this reaction decreases. If ATP is in short supply, the rate increases. If not already, the reason will become clear shortly.
Step 2:
In step two, citrate loses one water molecule and gains another as citrate converts into its isomer, isocitrate.
Step 3:
In step three, isocitrate is oxidized by NAD+ and decarboxylated. Keep track of the carbons! This carbon now more than likely leaves the cell as waste and is no longer available for building new biomolecules. The oxidation of isocitrate therefore produces a five-carbon molecule, α-ketoglutarate, a molecule of CO2 and NADH. This step is also regulated by negative feedback from ATP and NADH, and via positive feedback from ADP.
Step 4:
Step 4 is catalyzed by the enzyme succinate dehydrogenase. Here, α-ketoglutarate is further oxidized by NAD+. This oxidation again leads to a decarboxylation and thus the loss of another carbon as waste. So far two carbons have come into the cycle from acetyl-CoA and two have left as CO2. At this stage, there is no net gain of carbons assimilated from the glucose molecules that are oxidized to this stage of metabolism. Unlike the previous step however succinate dehydrogenase - like pyruvate dehydrogenase before it - couples the free energy of the exergonic red/ox and decarboxylation reaction to drive the formation of a thioester bond between the substrate co-enzyme A and succinate (what is left after the decarboxylation). Succinate dehydrogenase is regulated by feedback inhibition of ATP, succinyl-CoA, and NADH.
Possible NB Discussion Point
We have seen several steps in this and other pathways that are regulated by allosteric feedback mechanisms. Why is it so important to be able to regulate cellular processes in the context of metabolism? Is there something(s) common to these regulated steps in the TCA cycle? Why might these steps be good steps to regulate in particular?
Step 5:
In step five, a substrate level phosphorylation event occurs. Here an inorganic phosphate (Pi) is added to GDP or ADP to form GTP (an ATP equivalent for our purposes) or ATP. The energy that drives this substrate level phosphorylation event comes from the hydrolysis of the CoA molecule from succinyl~CoA to form succinate. Why is GTP or ATP produced? In animal cells there are two isoenzymes (different forms of an enzyme that carries out the same reaction), for this step, depending upon the type of animal tissue in which we find those cells. We find one isozyme in tissues that use large amounts of ATP, such as heart and skeletal muscle. This isozyme produces ATP. We find the second isozyme of the enzyme in tissues that have many anabolic pathways, such as liver. This isozyme produces GTP. GTP is energetically equivalent to ATP; However, its use is more restricted. In particular, the process of protein synthesis primarily uses GTP. Most bacterial systems produce GTP in this reaction.
Step 6:
Step six is another red/ox reactions in which succinate is oxidized by FAD+ into fumarate. Two hydrogen atoms are transferred to FAD+, producing FADH2. The difference in reduction potential between the fumarate/succinate and NAD+/NADH half reactions does not make NAD+ a suitable reagent for oxidizing succinate with NAD+ under cellular conditions. However, the difference in reduction potential with the FAD+/FADH2 half reaction is adequate to oxidize succinate and reduce FAD+. Unlike NAD+, FAD+ remains attached to the enzyme and transfers electrons to the electron transport chain directly. A cell makes this process possible by localizing the enzyme catalyzing this step inside the inner membrane of the mitochondrion or plasma membrane (depending on whether or not the organism in question is eukaryotic).
Step 7:
Water is added to fumarate during step seven, and malate is produced. The last step in the citric acid cycle regenerates oxaloacetate by oxidizing malate with NAD+. Another molecule of NADH is produced in the process.
Summary
Note that this process (oxidation of pyruvate to Acetyl-CoA followed by one "turn" of the TCA cycle) completely oxidizes 1 molecule of pyruvate, a 3 carbon organic acid, to 3 molecules of CO2. Overall, 4 molecules of NADH, 1 molecule of FADH2, and 1 molecule of GTP (or ATP) are also produced. For respiring organisms this is a significant mode of energy extraction, since each molecule of NADH and FAD2 can feed directly into the electron transport chain, and as we will soon see, the subsequent red/ox reactions that are driven by this process will indirectly power the synthesis of ATP. The discussion so far suggests that the TCA cycle is primarily an energy extracting pathway; evolved to extract or convert as much potential energy from organic molecules to a form that cells can use, ATP (or the equivalent) or an energized membrane. However - and let us not forget - the other important outcome of evolving this pathway is the ability to produce several precursors or substrate molecules necessary for various catabolic reactions (this pathway provides some early building blocks to make bigger molecules). As we will discuss below, there is a strong link between carbon metabolism and energy metabolism.
Connections to Carbon Flow
One hypothesis that we have explored in this reading and in class is the idea that "central metabolism" evolved to generate carbon precursors for catabolic reactions. Our hypothesis also states that as cells evolved, these reactions became linked into pathways: glycolysis and the TCA cycle, to maximize their effectiveness for the cell. We can postulate that a side benefit to evolving this metabolic pathway was the generation of NADH from the complete oxidation of glucose - we saw the beginning of this idea when we discussed fermentation. We have already discussed how glycolysis not only provides ATP from substrate level phosphorylation but also yields a net of 2 NADH molecules and 6 essential precursors: glucose-6-P, fructose-6-P, 3-phosphoglycerate, phosphoenolpyruvate, and pyruvate. While ATP can be used by the cell directly as an energy source, NADH poses a problem and must be recycled back into NAD+, to keep the pathway in balance. As we see in the fermentation module, the most ancient way cells deal with this problem is to use fermentation reactions to regenerate NAD+.
During the process of pyruvate oxidation via the TCA cycle, 4 additional essential precursors are formed: acetyl~CoA, α-ketoglutarate, oxaloacetate, and succinyl~CoA. Three molecules of CO2 are lost, and this represents a net loss of mass for the cell. These precursors, however, are substrates for a variety of catabolic reactions including the production of amino acids, fatty acids, and various co-factors, such as heme. This means that the rate of reactions through the TCA cycle will be sensitive to the concentrations of each metabolic intermediate (more on the thermodynamics in class). A metabolic intermediate is a compound that is produced by one reaction (a product) and then acts as a substrate for the next reaction. This also means that metabolic intermediates, in particular the 4 essential precursors, can be removed for catabolic reactions, if there is a demand, changing the thermodynamics of the cycle.
Possible NB Discussion Point
Some of the TCA cycle intermediates, particularly glutamate and succinyl-CoA, are diverted away from the TCA cycle to other reactions. Unless something is done about it, this can leave the TCA cycle with too few intermediates to function effectively. Either through your own imagination or external study (hint: search "anapleurotic reactions" with a search engine) describe how this problem might be solved. What is required for this to happen? As a follow-up, you can also try to explain what you might need to run the TCA cycle backwards. Many organisms need to do this. What is required for this to happen and under what circumstances might it be helpful? Search "reductive Krebs Cycle" for leads.
Not all cells have a complete TCA cycle.
Since all cells require the ability of make these precursor molecules, one might expect that all organisms would have a fully complete TCA cycle. In fact, the cells of many organisms DO NOT have all the enzymes required to form a complete cycle - all cells, however, DO have the capability of making the 4 TCA cycle precursors noted in the previous paragraph. How can the cells make precursors and not have a full cycle? Remember that most of these reactions are freely reversible, so, if NAD+ is required to for the oxidation of pyruvate or acetyl~CoA, then the reverse reactions would require NADH. We often refer to this process as the reductive TCA cycle. To drive these reactions in reverse (regarding the direction discussed above) requires energy, in this case carried by ATP and NADH. If you get ATP and NADH driving a pathway one direction, driving it in reverse will require ATP and NADH as "inputs". So, organisms that do not have a full cycle can still make the 4 key metabolic precursors by using previously extracted energy and electrons (ATP and NADH) to drive some key steps in reverse.
Additional Links
Here are some additional links to videos and pages that you may find useful.
Chemwiki Links
- Chemwiki TCA cycle - link down until key content corrections are made to the resource
PRACTICE POST GUIDE
General Practice
3. Why: One of the “big” lessons in Bis2a learning how to connect different lessons and concepts together. Nowhere is it more straightforward to make connections - and to think about what it means for the “bigger picture” - that in our discussion of central metabolism. To really “get it”, however, you need to start building a mental picture of the connections between the core pathways we talk about in the class. Practice that here.
How to practice: I suggest that you practice recreating a high-level overview of glycolysis and TCA cycle. Let’s do that here!
-
- Try to write down the two high-level questions that we wanted to ask with our discussion of glycolysis. From a Design Challenge perspective, what were the two key problems that we wanted to think more about?
- We talked about one of these problems at length in the context of a redox reaction catalyzed by glyceraldehyde-3-phosphate dehydrogenase. Here, try to summarize that reactions’ energy story and recreate it from scratch if you can. Use our new vocabulary when you think it helps your explanation. The story includes concepts from redox chemistry, energetics, and new bond types, as well as comparisons of free-energies of hydrolysis.
- The second part of the story of glycolysis has to do with some of the special 12 compounds that make “everything”—summarize it.
This exercise helps you practice learning objectives: ME.16 Apply the concept of the “conservation of mass” to metabolism by describing the different forms mass takes as it enters and leaves the cell (e.g. input: reduced molecules like glucose, lipids, proteins, etc. & output: oxidized molecules like CO2, H2O etc.).; ME.15 Apply the concept of the "conservation of energy" to central metabolism. Follow energy from "sources" of electrons with relatively low reduction potentials to "sinks" with higher redox potentials, describe the major transfers of energy and how this energy is "stored" at each stage.; ME.14 Given metabolic maps of glycolysis and TCA cycle, follow the flow of electrons from energy source to mobile carrier NADH and use a provided redox tower to quantitatively describe the energy transformations.; ME.10 Explain the central role of Acetyl CoA in carbon metabolism including its formation from various sources (the oxidative decarboxylation of pyruvate, oxidation of fatty acids, and/or oxidative degradation of some amino acids) and as a carbon source in both the TCA cycle and lipid synthesis.; ME.7 Create an "energy story" for glycolysis. The story should list the overall reactants and products, sources of energy, energy transfers, the types of reactions involved in energy transfer, and the mediators of the transformations of matter and transfers of energy.
4. Why: ATP is a crucially important molecule in biological systems - it plays many different roles. Here we want to review its role as an energy carrier and make sure we understand some of the basis of how “it works”.
How to practice: As discussed in the “book”, we often talk about the hydrolysis of ATP to ADP+Pi as an exergonic process. Your chemistry professor will correctly tell you that breaking bonds takes energy. How can both of these observations be true? What is the distinction between saying that we “hydrolyze ATP" and “breaking the phosphate bond” that makes both of the previous statements true? Why does the teaching staff take great pains to note the the “hydrolysis of certain bonds” is energetically favorable?
This exercise helps you practice learning objectives: ME.5 Explain the important contribution of water in determining the negative ΔG0’ of the hydrolysis of a phosphoanhydride bond in ATP.; MS.27 Identify the “high-energy” bonds in ATP. - These learning goals carry over from the previous lecture, but we’ll keep practicing here!
5. Why: Acetyl-CoA is a critical molecule for many reactions in biological systems. In our class we see it as a link between glycolysis and the TCA cycle and as a precursor for lipid synthesis or product of their breakdown. Here we look at its role connecting glycolysis and the TCA cycle. At this point in the course we are building on material and learning goals from numerous previous lectures.
How to practice: Can you identify places in the reaction below involving Acetyl-CoA where you’d need to understand material/concepts from previous lectures to fully explain or the reaction? For instance:
- Can you identify functional groups in these molecules?
- Can you say something about why we consider them functional groups?
- Can you draw a reaction coordinate diagram (graph) with properly labeled axes?
- Can you describe endergonic and exergonic components of this reaction?
- Can you explain to us what the ∆G tells us about how fast this reaction will go?
- Can you describe what the role of the enzyme is and how it does its job?
- Can you make reasonable hypotheses about how the enzyme and the substrates will interact at the molecular level?
- If given pKa values for certain functional groups, can you say something about how pH might influence the interactions of these substrates with the enzyme or the enzyme’s functional groups?
- Can you describe what role coenzyme A plays in this reaction?
This exercise helps you practice learning objectives: ME.18 Relate Coenzyme A's ability to form thioesters with the concepts of its function of carrying "activated" acyl groups.; ME.10 Explain the central role of Acetyl CoA in carbon metabolism including its formation from various sources (the oxidative decarboxylation of pyruvate, oxidation of fatty acids, and/or oxidative degradation of some amino acids) and as a carbon source in both the TCA cycle and lipid synthesis.
6. Why: Just in case you didn’t have enough practice with Acetyl-CoA we can examine/review the reaction involved in its formation from pyruvate (the end product of glycolysis).
How to practice: Pyruvate oxidation and the formation of acetyl-CoA links glycolysis to the TCA cycle.
- Energy story: What are the reactants and the products in this reaction? What is the enzyme?
- Can you say anything about the how the enzyme involved in this reaction might work? This is a bit complicated, but think back to the lesson in glycolysis involving an enzyme that linked redox to the formation of new bonds (and the role of a thioester linkages).
This exercise helps you practice learning objectives: ME.18 Relate Coenzyme A's ability to form thioesters with the concepts of its function of carrying "activated" acyl groups.; ME.10 Explain the central role of Acetyl CoA in carbon metabolism including its formation from various sources (the oxidative decarboxylation of pyruvate, oxidation of fatty acids, and/or oxidative degradation of some amino acids) and as a carbon source in both the TCA cycle and lipid synthesis.
7. Why: Fermentation is a key process for recycling NADH into NAD+ and in the production of many
How to practice: In the reading assignments and in lecture, you were given the problem of figuring out what options the cell might reasonably have to re-oxidize the NADH to NAD+ in order to avoid consuming the available pools of NAD+ and thus stopping glycolysis. In today’s lecture we discussed one solution—fermentation.
- What is your definition of fermentation? Does it relate to oxygen or to regenerating NAD+ pools?
- Can fermentation happen in the presence of oxygen?.
- Is fermentation the same thing as anaerobic respiration?
- We will revisit this topic again so start thinking about it. Be aware that the last two parts of this question refer to a very common misconception.
This exercise helps you practice learning objectives: ME.8 Create an “energy story” explaining the functional value of pyruvate reduction (fermentation) to a cell and its importance in regenerating the NAD+ pool.
8. Why: We want to learn how to recognize Coenzyeme A.
How to practice: Coenzyme A is depicted below. The part of the molecule circled in red was derived from the amino acid cysteine. Another part was derived from a nucleotide.
Circle the part that came from a nucleotide.
The part of the molecule that came from cysteine is missing a portion of the amino acid. Look at the structure of cysteine. What is different between the structure of cysteine and the part of coenzyme A circled in red?
This exercise helps you practice learning objectives: ME.19 Identify Coenzyme A and its functionally important thiol group by inspection of a molecular model.
9. Why: Here is more practice with Coenzyme A.
How to practice: We have now discussed the sulfhydryl functional group ~SH in multiple lecture. (Its chemical class is thiol.) Be able to recognize this functional group. This same chemistry (formation of thioester) showed up in the discussion of the active site of glyceraldehyde-3-phosphate dehydrogenase.
- What role did this group serve in the energy story of this reaction?
- What role do you think it is going to have in coenzyme A?
This exercise helps you practice learning objectives: ME.19 Identify Coenzyme A and its functionally important thiol group by inspection of a molecular model.; ME.18 Relate Coenzyme A's ability to form thioesters with the concepts of its function of carrying "activated" acyl groups.
10. Why: We discussed the need to recycle NADH to NAD+ and the role that fermentation can play in that process. Here we want to practice thinking about the reactions involved in fermentation and whether we can extend the core ideas to
How to practice: Is the conversion of NAD+ to NADH an oxidation or a reduction? Now examine the table of reduction potentials on the next page and identify four different reactions that could be coupled to the NAD+/NADH reaction to regenerate the NAD+ pools. One of the products is NAD+. What is the other product of the coupled reaction?
oxidant |
reductant |
n (electrons) |
Eo ́ (volts) |
CO2 |
glucose |
24 |
-0.43 |
Pyruvate + CO2 |
malate |
2 |
-0.33 |
NAD+ + 2H+ |
NADH + H+ |
2 |
-0.32 |
1,3 bisphosphoglycerate + 2H+ |
glyceraldehyde-3-P + Pi |
2 |
-0.29 |
FAD+ (free) + 2H+ |
FADH2 |
2 |
-0.22 |
Acetaldehyde + 2H+ |
ethanol |
2 |
-0.20 |
Pyruvate + 2H+ |
lactate |
2 |
-0.19 |
Oxaloacetate + 2H+ |
malate |
2 |
-0.17 |
α-ketoglutarate + NH4+ |
glutamate |
2 |
-0.14 |
Fumarate + 2H+ |
succinate |
2 |
0.03 |
1/2 O2 + 2H+ |
H2O |
2 |
0.816 |
Since other factors influence whether or not an organism can use a particular half-reaction to do fermentation, the half-reaction might not be used in the “real” world. This exercise is supposed to get you to think about all the possibilities. Scientists are discovering microorganisms that grow on all sorts of compounds, natural and man-made. New metabolic pathways and maybe even new fermentation pathways can be discovered in these microorganisms.
This exercise helps you practice learning objectives: ME.8 Create an “energy story” explaining the functional value of pyruvate reduction (fermentation) to a cell and its importance in regenerating the NAD+ pool. Note: This also reviews some of our skills with redox and redox towers (always bringing back previous lessons).
11. Why: NAD+ is an important cellular electron carrier. However, other molecules also act as electron carriers. Here we introduce a new carrier in the context of a metabolic pathway and ask you to integrate some of the various lessons that we’ve learned so far in a new context.
How to practice: This question will challenge you to track the path of electrons across multiple different types of carriers. Look at the figure of the hydrogenosome below. The little numbers represent enzymes that catalyze reactions. Fd is a protein called ferredoxin that can exist in both an oxidized form (Fd+) and reduced form (Fd-). Complex I is an electron transport chain that can also exist in two states.
First, examine the flow of electrons from pyruvate. Where are the electrons from pyruvate going during the oxidation step to make acetyl-CoA? What gets oxidized and what gets reduced in this reaction? What finally accepts those electrons in this fermentation reaction (it's a bit different than the theme we saw in class with the sugars)? What is different about this conversion of pyruvate to acetyl-CoA than what we saw in class? How does it function by similar principles?
Second, examine the synthesis of ATP by enzyme 7. Create an energy story that tracks where the energy came from to make that ATP from the oxidation of pyruvate. Is this familiar or similar to another reaction you saw elsewhere?
This exercise helps you practice learning objectives: ME.15 Apply the concept of the "conservation of energy" to central metabolism. Follow energy from "sources" of electrons with relatively low reduction potentials to "sinks" with higher redox potentials, describe the major transfers of energy and how this energy is "stored" at each stage.; ME.16 Apply the concept of the “conservation of mass” to metabolism by describing the different forms mass takes as it enters and leaves the cell (e.g. input: reduced molecules like glucose, lipids, proteins, etc. & output: oxidized molecules like CO2, H2O etc.).; ME.18 Relate Coenzyme A's ability to form thioesters with the concepts of its function of carrying "activated" acyl groups.
12. Why: The TCA cycle is a central pathway for carbon flow and energy extraction. One of its reactions also serves as another example of the role of thioester formation and the role of Coenzyme A.
How to practice: The conversion of alpha-ketoglutarate to succinyl-CoA involves various reactants and products. Construct an energy story for this reaction. Do this for the other highlighted steps in the TCA cycle shown in class.
This exercise helps you practice learning objectives: ME.12 Create an energy story for each reaction in the TCA cycle.; ME.18 Relate Coenzyme A's ability to form thioesters with the concepts of its function of carrying "activated" acyl groups.
13. Why: Continuing to practice skills from previous lectures is important - here’s one that revisits lessons about ATP.
How to practice: One of the ways that the ATP supply in muscle tissue is regenerated is through the molecule creatine phosphate (or phosphocreatine). Look at the reaction below. Tell its energy story for this process. Which mechanism is used to produce new ATP?
This exercise helps you practice learning objectives: ME.4 Explain the process of substrate level phosphorylation (SLP) and identify the SLP reactions when given a collection of reactions, such as in a pathway.
PRACTICE EXAM QUESTION
Question Q13.1
Q13.1 All of the following are products of the Citric Acid (TCA) Cycle except_____.
- CO2
- GTP
- NAD+
- H2O
- All of the above are products of the TCA cycle.
Question Q13.2 (from a Canadian MCAT-like exam)
Q13.2 GAPDH converts an aldehyde into a carboxylic acid in order to change NAD+ to NADH. Which of the following is true regarding this reaction in humans?
- The aldehyde is reduced.
- NAD+ is oxidized.
- NAD+ gains 2 electrons and one proton.
- The carboxylic acid (pKa = 2.19) is mostly in its protonated form.
Question Q13.3
Q13.3 Which process (ethanol or lactic acid fermentation) harvests the most chemical energy from pyruvate?
- ethanol fermentation
- lactic acid fermentation
- both produce the same amount
- It depends on whether oxygen is present.
Question Q13.4
Q13.4 Complete the following sentence which tells part of the “energy story” for the reaction below:
The _______ of 2-oxoadipate by NAD+ is a(n)____________ reaction that helps drive a decarboxylation and the formation of a thioester bond involving coenzyme A.
- oxidation, exergonic
- oxidation, endergonic
- reduction, exergonic
- reduction, endergonic
- None of the above.
Question Q13.5
Q13.5 Which mechanism of ATP production is being used in the reaction to the right?
- oxidative phosphorylation
- photophosphorylation
- substrate-level phosphorylation
- None of the above.
- More information is needed for me to answer this question.