4.6: Exceptions to simple dominance
- Last updated
- Save as PDF
- Page ID
- 102500
Learning Objectives
- Categorize interactions between alleles of single genes as completely dominant, incompletely dominant, or codominant and explain the biochemical basis of these relationships. Predict phenotypes of offspring using each mode of inheritance.
- Understand that multiple alleles (not just two) may exist for a gene.
- Recognize examples of incomplete penetrance, variable expressivity, and pleiotropy.
- Predict ratios of offspring when certain genotypes are lethal alleles.
Incomplete Dominance
During Mendel’s time, people believed in a concept of blending inheritance whereby offspring demonstrated intermediate phenotypes between those of the parental generation. This was refuted by Mendel’s pea experiments that illustrated a Law of Dominance. Despite this, non-Mendelian inheritance can be observed in sex-linkage and co-dominance where the expected ratios of phenotypes are not observed clearly. Incomplete dominance superficially resembles the idea of blending inheritance, but can still be explained using Mendel’s laws with modification. In this case, alleles do not exert full dominance and the offspring resemble a mixture of the two phenotypes.

The most obvious case of a two allele system that exhibits incomplete dominance is in the snapdragon flower. The alleles that give rise to flower coloration (Red or White) both express and the heterozygous genotype yields pink flowers. There are different ways to denote this. In this case, the superscripts of R or W refer to the red or white alleles, respectively. Since no clear dominance is in effect, using a shared letter to denote the common trait with the superscripts (or subscripts) permit for a clearer denotation of the ultimate genotype to phenotype translations.
Exercise \(\PageIndex{1}\)
If pink flowers arose from blending inheritance, then subsequent crosses of pink flowers with either parental strain would continue to change the phenotype. Using a Punnett Square, perform a cross between a heterozygous plant and a parental to predict the phenotypes of the offspring.
- Answer
-
A cross between a heterozygote FRFW and a red parental FRFR would produce 1/2 red-flowered and 1/2 pink-flowered offspring.
FR
FR FR
FRFR (red) FRFR (red) FW
FRFW (pink) FRFW (pink)
Thinking about the biochemical basis of incomplete dominance
Refer back to the biochemical basis of dominant and recessive alleles. Why do many loss-of-function alleles act as recessive? What must be different about incompletely dominant alleles? How would you test your idea?
The alleles that determine curly or straight hair in dogs provide another example of incomplete dominance. Using GWAS, the KRT71 gene, which encodes a keratin protein, was identified as the locus that determines this phenotype (Cadieu et al, 2010). Dogs with C alleles for a SNP in the KRT71 gene exhibit straight hair, while dogs with curly hair have two T alleles for the SNP that alter an amino acid, which is a missense mutation. When curly and straight haired dogs are bred, such as the cross of poodles and Labrador Retrivers that create Labradoodles, the offspring are heterozygous for the SNP (C/T) and have an intermediately wavy hair.
Query \(\PageIndex{1}\)
Codominance and Multiple Alleles
Codominance occurs when phenotypes associated with two dominant alleles occur simultaneously. The prototypical case for this is the human ABO blood grouping. The ABO gene (also known as the I locus) on human chromosome 9 encodes a glycosyltransferase enzyme that attaches a carbohydrate molecule to proteins present on the surface of red blood cells.
The IA and IB alleles have several DNA sequence differences that produce amino acid changes. As a result, the IA allele produces an enzyme that adds an acetylated galactosamine (GalNac) to a surface protein. The IB allele produces an enzyme that adds galactose (Gal) instead. These alleles are codominant to each other because each the enzymes catalyze reactions that occur independently of the other enzymes that are present. On the surface of red blood cells in heterozygous individuals (IAIB), a mixture of proteins with GalNAc and Gal will be present.
A third allele of the gene, designated i, also exists but contains a single nucleotide deletion that causes a frameshift, completing disrupting the protein (Yamamoto et al, 1990). The protein produced by the i allele does not catalyze the attachment of any sugar. This allele is recessive to IA and IB because the protein has no activity and does not influence the activity of the proteins encoded by the dominant alleles. If an IA or IB allele is also present, those enzymes will still function, so the A or B antigens will be present, producing the dominant phenotype.
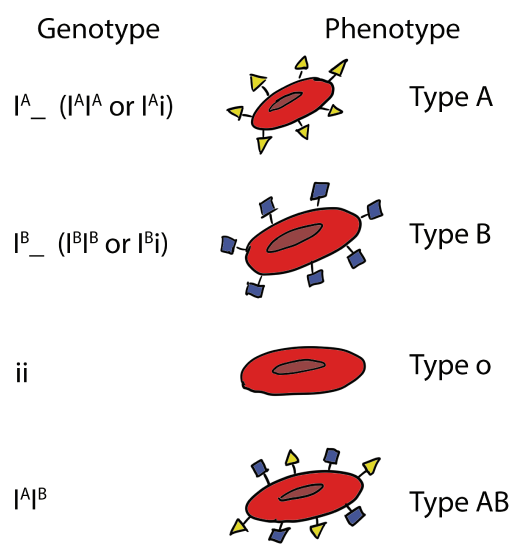
Query \(\PageIndex{2}\)
Query \(\PageIndex{3}\)
Importance of ABO Blood Types
The alleles of the ABO gene determine features that are present on the surface of blood cells that are used when cells identify foreign cells. An individual with blood type A has proteins with the GalNac modification, but does not have proteins with the Gal modification produced by the IB allele. The individual will produce antibodies that recognize the Gal modification that is present in Type B blood. Therefore, if Type B blood should not be introduced into a Type A individual.
Blood type influences blood donors and recipients
The table shows how blood type influences blood donations. If an individual receives a blood type that they make antibodies against, the blood will be recognized as foreign and the immune system will attack the donated cells. Because individuals will Type O blood have cells without any sugar modifications, their blood can be donated to any other blood type; they are sometimes called universal donors. In contrast, individuals with Type AB blood recognize either the GalNac or Gal modification and can receive any type of blood; they are sometimes called universal recipient.
Blood type | Antibodies against: | Can donate blood to: | Can receive blood from: |
A | B | A or AB | A or O |
B | A | B or AB | B or O |
AB | none | AB | A, B, AB, or O |
O | A and B | A, B, AB, or O | O |
There are additional genes that influence human blood type, for example, blood type might also be described as O positive or AB negative. Whether blood type is positive or negative is determined by a second locus, the RHD gene, which encodes a surface protein called the Rh Blood Group D Antigen. As Mendelian inheritance predicts, the two genes, ABO on chromosome 9 and RHD on chromosome 1, are inherited independently during meiosis.
Penetrance and Expressivity
The terms penetrance and expressivity are also useful to describe the relationship between certain genotypes and their phenotypes.
- Penetrance is the proportion of individuals (usually expressed as a percentage) with a particular genotype that display a corresponding phenotype. For example, all pea plants that are homozygous for the allele for white flowers actually have white flowers, this genotype is completely penetrant. In contrast, many human genetic diseases are incompletely penetrant, because not all individuals with the disease genotype actually develop symptoms associated with the disease.
- Expressivity describes the variability in mutant phenotypes observed in individuals with a particular phenotype. Many human genetic diseases provide examples of broad expressivity, because individuals with the same genotypes may vary greatly in the severity of their symptoms. Incomplete penetrance and broad expressivity are due to random chance, non-genetic (environmental), and genetic factors (mutations in other genes), although often it remains unknown which of these cause(s) are most responsible for individual phenotypes.
Example of variable penetrance and expressivity from nematodes
Mutations in the gene meg-1 in C. elegans provide an example of variable penetrance as well as broad expressivity. This gene encodes a protein (MEG-1) that functions to establish the reproductive system in cells during early embryonic development. Mutant worms that lack meg-1 therefore produce sterile offspring. However, the sterile phenotype is not fully penetrant, approximately 20-25% of mutant offspring will be fertile, despite the lack of MEG-1. These sterile adults also exhibit variable expressivity; some sterile adults have severely-reduced germ-line development with few identifiable germ cells, whereas other sterile adults have an identifiable germ line but lack gametes.
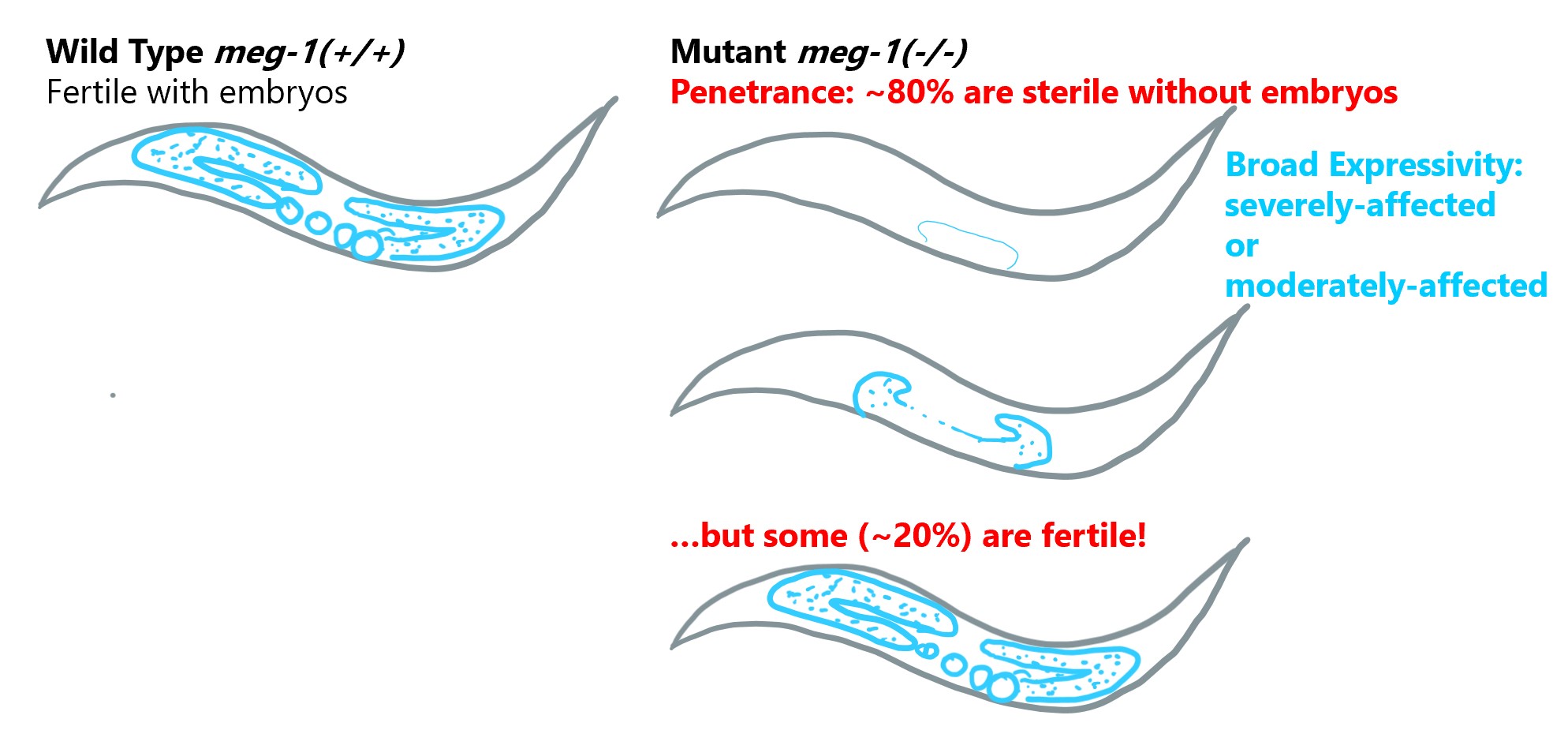
Data summarized from Leacock and Reinke, 2008
Pleiotropy
While some genes are associated with a single trait, many genes encode proteins that function in multiple ways or are the basis for other traits. Pleiotropy occurs when a single genotype affects multiple phenotypes. For example, the human gene CFTR is associated with the disease cystic fibrosis. However, variations in this gene are also associated with other phenotypes, such as congenital bilateral absence of the vas deferens (a feature that causes male infertility) and pancreatitis.
Pleiotropy is often observed when a gene is expressed in more than one tissue or organ. The CFTR protein plays a role in ion balance in the lungs, where lack of the protein is associated with the thick lung mucus characteristic of cystic fibrosis. However, CFTR is expressed and functions in ion balance in other tissues as well. Experiments that assay transcripts in multiple tissues, show that the CFTR mRNA is present in a number of tissues, including pancreas and testis. This expression pattern is consistent with some of the observed phenotypes that occur when CFTR is mutated.
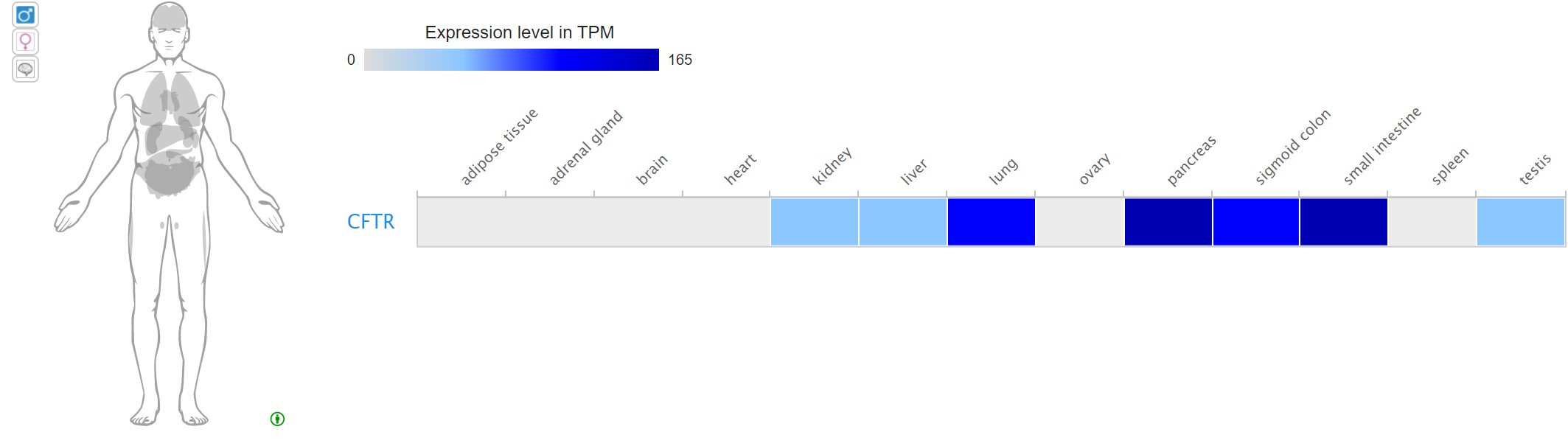
Lethal Alleles
Mutations that cause loss-of-function of a protein that is essential for organismal viability could result in lethality; often these alleles are recessive because the second copy of the gene will produce sufficient protein for survival. When two heterozygotes are crossed, 25% of offspring are expected to be homozygous for the lethal allele. Thus, the ratio of homozygous wild type to heterozygotes among the surviving offspring will be 2:1 and the offspring may all have the same phenotype, depending on the relationship between the two alleles.
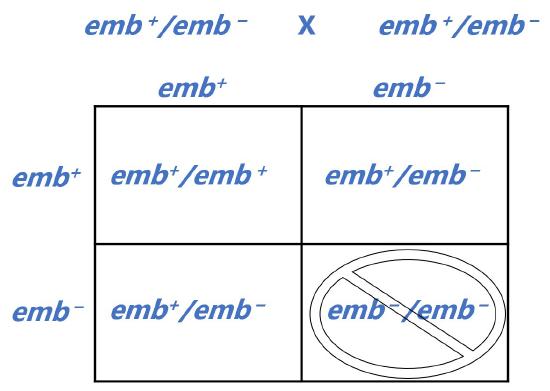
For some alleles, the heterozygote may have a different phenotype than the homozygote, in which case the phenotypic ratios of the progeny may differ from the expected ratios. The agouti yellow (Ay) allele in mice is one example of an allele that produces a dominant yellow phenotype in heterozygotes, as well as pleiotropic effects including obesity and increased tumor incidence. Mammals typically produce two types of pigments, pheomelanin (a yellow pigment) and eumelanin (a black-brown pigment). The protein encoded by agouti is a signaling protein that suppresses a receptor controlling eumelanin production. When agouti protein is present transiently during hair production, individual hairs have eumelanin at the base and tip with a central band of pheomelanin, giving an overall brown fur appearance. In A/Ay heterozygotes agouti is constantly expressed, suppressing eumelanin completely and the phenotype is yellow. The pleiotropic effects observed are likely due to interactions of the Agouti signaling protein with similar receptors that control weight and feeding behavior.
The Ay allele is lethal when homozygous due to the nature of the mutation. The Ay allele is a large (170kb) deletion that removes a part of an upstream gene Raly, which stands for RNA-binding protein associated with the lethal yellow mutation, and the agouti promoter (Michaud et al 1993; Duhl et al 1994). Now the Raly promoter controls the transcription of agouti, such that it is overproduced, suppressing melanin production and leading to yellow fur. Due to the size and position of the deletion, the coding sequences of the Raly protein are absent in the Ay allele. In heterozygous embryos a functional copy of Raly remains, but Raly protein, which is normally expressed in early mouse embryos (preimplantation), is absent in homozygous Ay/Ay embryos. When translation of the Raly mRNA was blocked in wild-type mouse embryos, only 4% of embryos developed to the blastocyst stage, consistent with the deletion of Raly, and not the effect of agouti, as the cause of the embryonic lethality (Duhl et al. 1994).
Example \(\PageIndex{1}\)
The mouse gene Agouti (A) influences the pattern of pigment in mouse fur. The mutant allele Ay produces fur that is entirely yellow, instead of the wild-type pattern of black hairs with a central band of yellow pigment. However, AyAy homozygous embryos die in early stages of development. You cross two yellow mice. What ratio of offspring genotypes and phenotypes do you expect?
Solution
The yellow mice must have the heterozygous genotype AAy. The fertilized embryos will have the genotype ratio 1 AyAy : 2 AAy : 1 AA.
However the AyAy embryos will not survive. The ratio of the surviving offspring will be 2 AAy yellow : 1 AA agouti.
References:
Cadieu E, Neff MW, Quignon P, Walsh K, Chase K, Parker HG, Vonholdt BM, Rhue A, Boyko A, Byers A, Wong A, Mosher DS, Elkahloun AG, Spady TC, André C, Lark KG, Cargill M, Bustamante CD, Wayne RK, Ostrander EA. Coat variation in the domestic dog is governed by variants in three genes. Science. 2009 Oct 2;326(5949):150-3. doi: 10.1126/science.1177808. Epub 2009 Aug 27. PMID: 19713490; PMCID: PMC2897713.
Duhl DM, Stevens ME, Vrieling H, Saxon PJ, Miller MW, Epstein CJ, Barsh GS. Pleiotropic effects of the mouse lethal yellow (Ay) mutation explained by deletion of a maternally expressed gene and the simultaneous production of agouti fusion RNAs. Development. 1994 Jun;120(6):1695-708. PMID: 8050375.
Howe K, et al Ensembl 2021. Nucleic Acids Res. 2021, vol. 49(1):884–891. PubMed PMID: 33137190.
Leacock SW, Reinke V. MEG-1 and MEG-2 are embryo-specific P-granule components required for germline development in Caenorhabditis elegans. Genetics. 2008 Jan;178(1):295-306. doi: 10.1534/genetics.107.080218. PMID: 18202375; PMCID: PMC2206079. (https://doi.org/10.1534/genetics.107.080218)
Lin S, Lin Y, Nery JR, Urich MA, Breschi A, Davis CA, Dobin A, Zaleski C, Beer MA, Chapman WC, Gingeras TR, Ecker JR, Snyder MP. Comparison of the transcriptional landscapes between human and mouse tissues. Proc Natl Acad Sci U S A. 2014 Dec 2;111(48):17224-9. doi: 10.1073/pnas.1413624111. Epub 2014 Nov 20. PMID: 25413365; PMCID: PMC4260565.
Michaud EJ, Bultman SJ, Klebig ML, et al. A molecular model for the genetic and phenotypic characteristics of the mouse lethal yellow (Ay) mutation. Proc Natl Acad Sci U S A. 1994 Mar 29;91(7):2562-6. doi: 10.1073/pnas.91.7.2562. PMID: 8146154; PMCID: PMC43409. (https://www.pnas.org/content/91/7/2562.long)
Papatheodorou I, Moreno P, Manning J, et al. Expression Atlas update: from tissues to single cells. Nucleic Acids Res. 2020 Jan 8;48(D1):D77-D83. doi: 10.1093/nar/gkz947. PMID: 31665515; PMCID: PMC7145605.
Yamamoto et al. Molecular genetic basis of the histo-blood group ABO system. Nature 345, 229–233 (1990). https://doi.org/10.1038/345229a0
Contributors and Attributions
Dr. Todd Nickle and Isabelle Barrette-Ng (Mount Royal University) The content on this page is licensed under CC SA 3.0 licensing guidelines.