4.5: Phototrophy
( \newcommand{\kernel}{\mathrm{null}\,}\)
As noted above, phototrophs use solar radiation rather than redox reactions to make ATP. However, redox reactions still play an important role in their metabolism. They use redox reactions to fix carbon, or in other words, convert it from an inorganic to organic form. For example, a phototroph that fixes carbon by converting carbon dioxide into glucose would need to add four electrons to each carbon dioxide from some electron source. Electrons are transferred in the reaction and thus it is a redox reaction.
We can divide phototrophic reactions into two major groups, the light and dark reactions. Light reactions are those that generate ATP, and dark reactions use ATP and fix carbon. Note that solar radiation is necessary for light reactions and sometimes also used in dark reactions, despite their name.
ATP is generated in light reactions through photophosphorylation, which is similar to oxidative phosphorylation. Electrons flow down an electron transport chain embedded in a membrane. A key difference is the source of electrons. The electrons are supplied by a redox reaction in chemotrophs but in phototrophs, the electrons are generated when solar radiation is absorbed by a pigment, such as chlorophyll, that is created by the phototroph.
Two major groups of phototrophs are defined based on whether their dark reactions generate molecular oxygen (O2). Oxygenic phototrophs produce molecular oxygen, whereas anoxygenic phototrophs do not. The difference between the two groups reflects the ultimate source of electrons in the dark reactions. If the electrons used to reduce carbon are produced by splitting water, molecular oxygen is produced and the phototrophy is oxygenic (Fig. 4.3A). However, instead of water, the electrons can ultimately come from reduced sulfur or iron, which can be dissolved or within a mineral (Bose et al., 2014; Guzman et al., 2019) (Fig. 4.3B). In that case, oxidized sulfur or iron is generated rather than oxygen and therefore the phototrophy is anoxygenic.
Some examples of oxygenic phototrophs are plants, algae, and cyanobacteria. At least six groups of Bacteria use anoxygenic phototrophy: green sulfur and non-sulfur bacteria, purple sulfur and non-sulfur bacteria, Heliobacteria, and Chloroacidobacteria (Tang et al., 2011). Pigments generated by phototrophic organisms, together with minerals that may form alongside their growth, provide some of the most vivid displays of microbial growth in nature (Fig. 4.4).
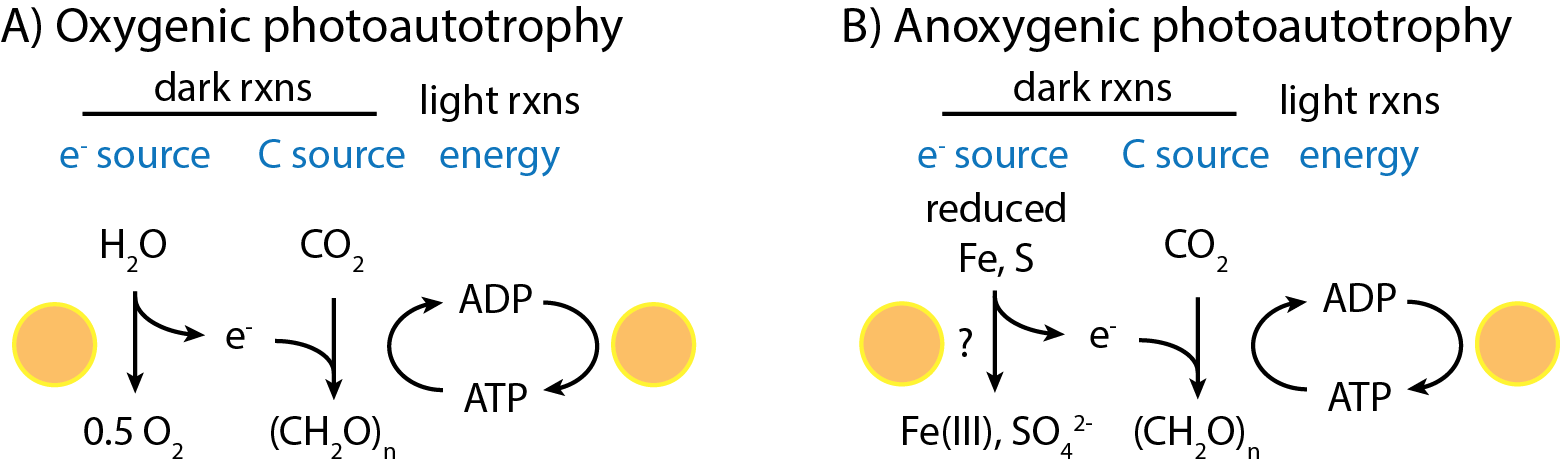
https://commons.wikimedia.org/wiki/File:Photoautotrophy.png

https://en.Wikipedia.org/wiki/File:Grand_Prismatic_Spring_and_Midway_Geyser_Basin_from_above.jpg