4.4: Chemotrophy
( \newcommand{\kernel}{\mathrm{null}\,}\)
Chemotrophic metabolic reactions can be divided into two major categories, catabolism and anabolism. Catabolic reactions are redox reactions that the organism uses to obtain energy and anabolic reactions are reactions that the organism uses to synthesize biological molecules. When the catabolic reaction includes an electron donor and an electron acceptor that are separate molecules, chemotrophic catabolism is referred to as respiration. For example, the following reaction can describe respiration by the yeast Saccharomyces cerevisiae: \text{C}_{6} \text{H}_{12} \text{O}_{6} + 6 \ \text{O}_{2} \longleftrightarrow 6 \ \text{CO}_{2} + 6 \ \text{H}_{2} \text{O}
The carbon in glucose \left(\text{C}_{6} \text{H}_{12} \text{O}_{6} \right) is oxidized and molecular oxygen is reduced. Therefore, we can say that glucose is the electron donor and oxygen is the electron acceptor in the catabolic reaction. Since they are separate molecules, the reaction is an example of respiration.
In contrast, when the catabolic reaction oxidizes and reduces portions of a single molecule, the chemotrophic catabolism is referred to as fermentation. For example, reaction (\PageIndex{1}) is used by Saccharomyces cerevisiae when molecular oxygen is available. However, when oxygen is not available, Saccharomyces cerevisiae cells can ferment glucose and produce ethanol \left(\text{CH}_{3} \text{CH}_{2} \text{OH}\right): \text{C}_{6} \text{H}_{12} \text{O}_{6} \longleftrightarrow 2 \ \text{CH}_{3} \text{CH}_{2} \text{OH} + 2 \ \text{CO}_{2}
This reaction is an example of alcohol fermentation. Two of the carbons in glucose are oxidized (0 to +4) to make carbon dioxide and four are reduced (0 to -2) to make ethanol. The carbons that were oxidized each donate four electrons for a total of eight (2 carbons × 4 electrons/carbon). The carbons that were reduced each accept two electrons also for a total of eight (4 carbons × 2 electrons/carbon). Thus, charge balance is maintained.
The two components of chemotrophic metabolism, catabolism and anabolism, are linked by the molecule adenosine triphosphate (ATP), which can be thought of as the energy currency of cells. ATP consists of a sugar, a nitrogenous base, and three phosphate molecules (Fig. 4.1). The bonds between the two outermost phosphate groups are high-energy anhydride bonds. As such, energy is effectively stored by creating the bonds through catabolic reactions. That energy can then be released by breaking the bonds in anabolic reactions.

ATP illustration source: https://commons.wikimedia.org/wiki/File:Adenosintriphosphat_protoniert.svg
Chemotrophic organisms generate ATP using two different strategies: substrate-level phosphorylation and oxidative phosphorylation. During substrate-level phosphorylation, a phosphate is added to adenosine diphosphate (ADP) from a high-energy phosphorylated organic compound that is produced when the organism catalyzes its catabolic reaction. During oxidative phosphorylation, ADP is phosphorylated via the electron transport chain. Respiring chemotrophic microorganisms typically generate ATP from oxidative phosphorylation whereas fermenters generally use substrate-level phosphorylation.
To further illustrate how energy can be captured from a redox reaction, we can consider oxidative phosphorylation in more detail. The electron transport chain is a series of proteins embedded in the cytoplasmic membrane of Bacteria and Archaea or the inner membrane of eukaryotic mitochondria. Electrons that are removed from the organism’s electron donor are deposited on the chain, often via an intermediate electron carrier molecule. Once deposited on the electron transport chain, the electrons flow from one protein to the next, ultimately making their way to the organism’s electron acceptor. We can think of the electron acceptor as a drain, which helps pull the electrons through the chain (Fig. 4.2).
As electrons flow along the electron transport chain, ions are pumped across the membrane. Usually those ions are hydrogen ions (i.e., protons). Because ions are being pumped across the membrane, electron flow along the transport chain works to increase the concentration of the ions on one side relative to the other. This gradient in ion concentration effectively provides a source of chemical potential energy that can be used to phosphorylate ADP and make ATP. This gradient is often specifically referred to as the proton motive force.
ADP phosphorylation occurs at a special protein in the membrane known as ATP synthase. At the protein, hydrogen ions flow back across the membrane, which allows a phosphate to be added to ADP: \text{ADP}^{2-} + \text{H}_{2} \text{PO}_{4}^{-} \longleftrightarrow \text{ATP}^{3-} + \text{H}_{2} \text{O} Under the conditions within cells, the forward direction of this chemical reaction is not favorable. However, the chemical energy derived from the ion gradient allows the enzyme to push the reaction forward. Thus, in summary, an ion gradient forms as a result of a redox reaction, and that gradient allows respiring organisms to produce ATP, which is then used as fuel for anabolic reactions.
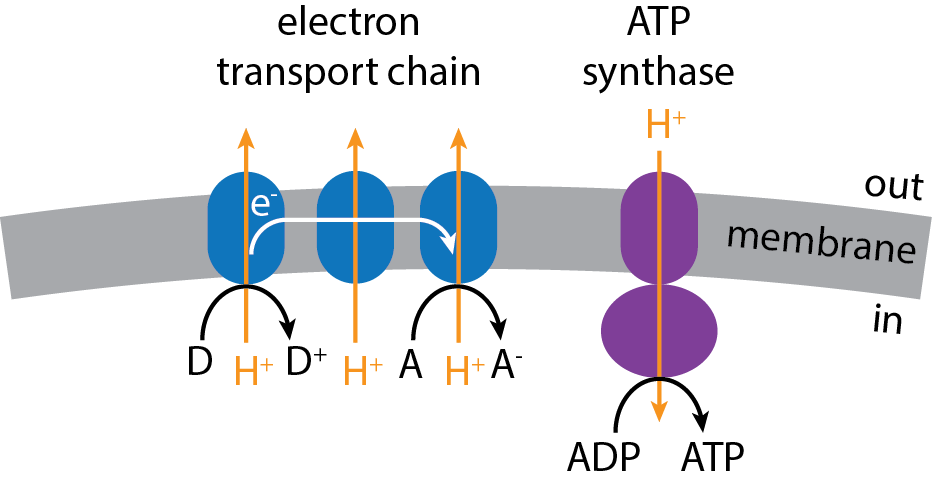
https://commons.wikimedia.org/wiki/File:Electron_transport_chain.png
Identify whether each of the following chemotrophic reactions is a respiration or fermentation reaction:
- \text{C}_{6} \text{H}_{12} \text{O}_{6} \longleftrightarrow \text{CH}_{3} \text{CHOHCOOH} + \text{C}_{2} \text{H}_{5} \text{OH} + \text{CO}_{2}
- \text{C}_{6} \text{H}_{12} \text{O}_{6} + 4 \ \text{H}_{2} \text{O} \longleftrightarrow 2 \ \text{CH}_{3} \text{COO}^{-} + 2 \ \text{HCO}_{3}^{-} + 4 \ \text{H}^{+} + 4 \ \text{H}_{2}
- \text{CH}_{3} \text{COO}^{-} + 4 \ \text{H}_{2} \text{O} \longleftrightarrow 4 \ \text{H}_{2} + 2 \ \text{HCO}_{3}^{-} + H^{+}
- \text{CH}_{3} \text{COO}^{-} + \text{H}^{+} \longleftrightarrow \text{CH}_{4} + \text{CO}_{2}
- \text{CO}_{2} + 4 \ \text{H}_{2} \longleftrightarrow \text{CH}_{4} + 2 \ \text{H}_{2} \text{O}
- \text{Fe}^{2+} + 1.2 \ \text{H}^{+} + 0.2 \ \text{NO}_{3}^{-} \longleftrightarrow \text{Fe}^{3+} + 0.1 \ \text{N}_{2} + 0.6 \ \text{H}_{2} \text{O}