21.3: Artificial Selection- Human-Initiated Change
- Last updated
- Save as PDF
- Page ID
- 74175
- Melissa Ha and Rachel Schleiger
- Yuba College & Butte College via ASCCC Open Educational Resources Initiative
The development of a new crop variety is an example of agricultural biotechnology, a range of tools that include both traditional breeding techniques and more modern lab-based methods. Traditional methods date back thousands of years, whereas biotechnology uses the tools of genetic engineering developed over the last few decades.
Selective Breeding (Artificial Selection)
Nearly all the fruits and vegetables found in your local market would not occur naturally. In fact, they exist only because of human intervention that began thousands of years ago. Humans created the vast majority of crop species by using traditional breeding practices on naturally-occurring, wild plants. These practices rely upon selective breeding (artificial selection), human-facilitated reproduction of individuals with desirable traits. For example, high yield varieties were produced through selective breeding. Traditional breeding practices, although low-tech and simple to perform, have the practical outcome of modifying an organism’s genetic information, thus producing new traits.
Selective breeding is limited, however, by the life cycle of the plant and the genetic variants that are naturally present. For example, even the fastest flowering corn variety has a generation time of 60 days (the time required for a seed to germinate, produce a mature plant, get pollinated, and ultimately produce more seeds) in perfect conditions. Each generation provides an opportunity to selectively breed individual plants and generate seeds that are slightly closer to the desired outcome (for example, producing bigger, juicier kernels). Furthermore, if no individuals happen to possess gene variants that result in bigger, juicier kernels, it is not possible to artificially select this trait. Finally, traditional breeding shuffles all of the genes between the two individuals being bred, which can number into the tens of thousands (maize, for example, has 32,000 genes). When mixing such a large number of genes, the results can be unpredictable.
An interesting example is maize (corn). Biologists have discovered that maize was developed from a wild plant called teosinte. Through traditional breeding practices, humans living thousands of years ago in what is now Southern Mexico began selecting for desirable traits until they were able to transform the plant into what is now known as maize (figure \(\PageIndex{a}\)). In doing so, they permanently (and unknowingly) altered its genetic instructions.
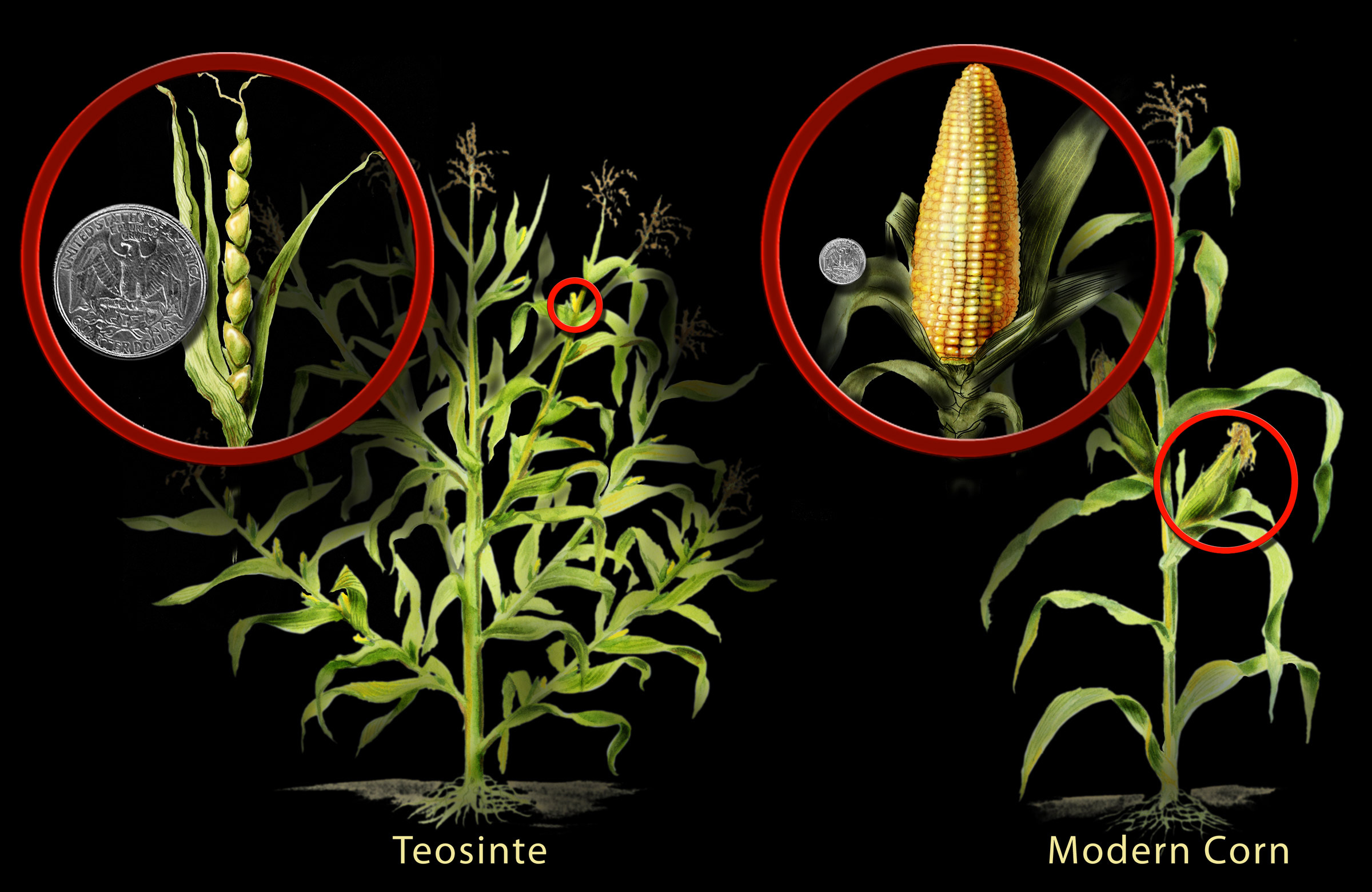
This history of genetic modification is common to nearly all crop species. For example, cabbage, broccoli, Brussel sprouts, cauliflower, and kale were all developed from a single species of wild mustard plant (figure \(\PageIndex{b}\)). Wild nightshade was the source of tomatoes, eggplant, tobacco, and potatoes, the latter developed by humans 7,000 – 10,000 years ago in South America.
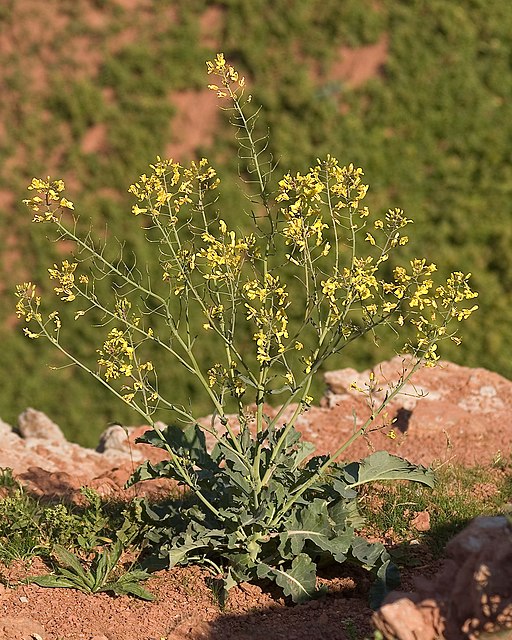
Genetic Engineering
Genetic engineering is the process of directly altering an organism's DNA to produce the desired crops more rapidly than selective breeding. Because genes can be obtained from other species or even synthesized in the lab, scientists are not limited by existing genetic variation within a crop species (or closely related species with which they can be crossed). This broadens the possible traits that can be added to crops. Modern genetic engineering is more precise than selective breeding in the sense that biologists can modify just a single gene. Also, genetic engineering can introduce a gene between two distantly-related species, such as inserting a bacterial gene into a plant (figure \(\PageIndex{c}\)).
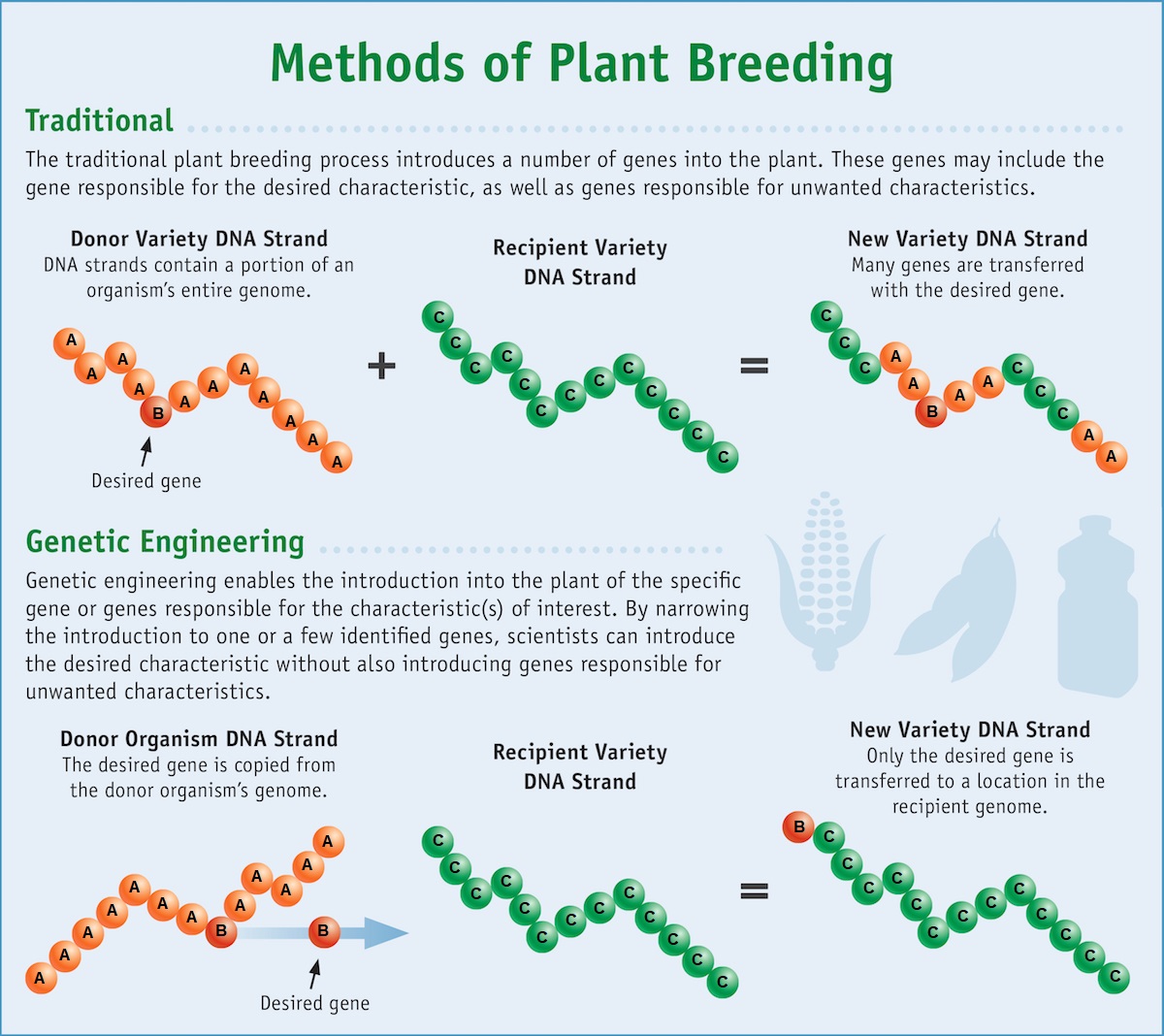
Genetically modified organisms (GMOs) are those that have had their DNA altered through genetic engineering. Genetically modified crops are sometimes called genetically engineered (GE) crops. Transgenic organisms are a type of genetically modified organism that contains genes from a different species. Because they contain unique combinations of genes and are not restricted to the laboratory, transgenic plants and other GMOs are closely monitored by government agencies to ensure that they are fit for human consumption and do not endanger other plant and animal life. Because these foreign genes (transgenes) can spread to other species in the environment, particularly in the pollen and seeds of plants, extensive testing is required to ensure ecological stability.
How to Genetically Modify Plant Cells
DNA can be inserted into plant cells through various techniques. For example, a gene gun propels DNA bound to gold particles into plant cells. (DNA is negatively charge and clings to positively charged gold.) A more traditional approach employs the plant pathogen Agrobacterium tumefaciens (figure \(\PageIndex{d}\)). Ordinarily, this bacterium causes crown gall disease in plants by inserting a circular piece of DNA, called the Ti plasmid, into plant cells. This DNA incorporates into plant chromosomes, giving them genes to produce the gall (figure \(\PageIndex{e}\)), which provides a home for the bacterial pathogen.
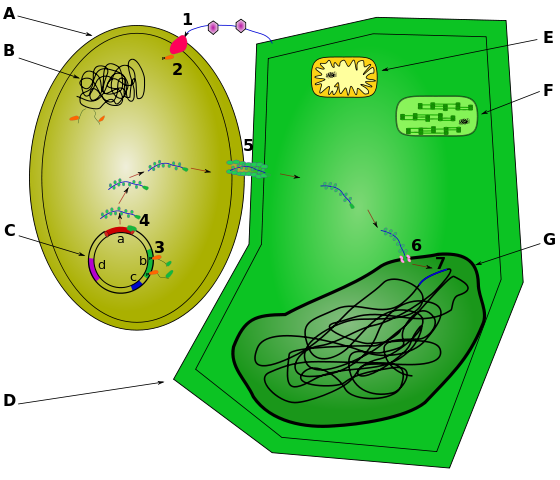
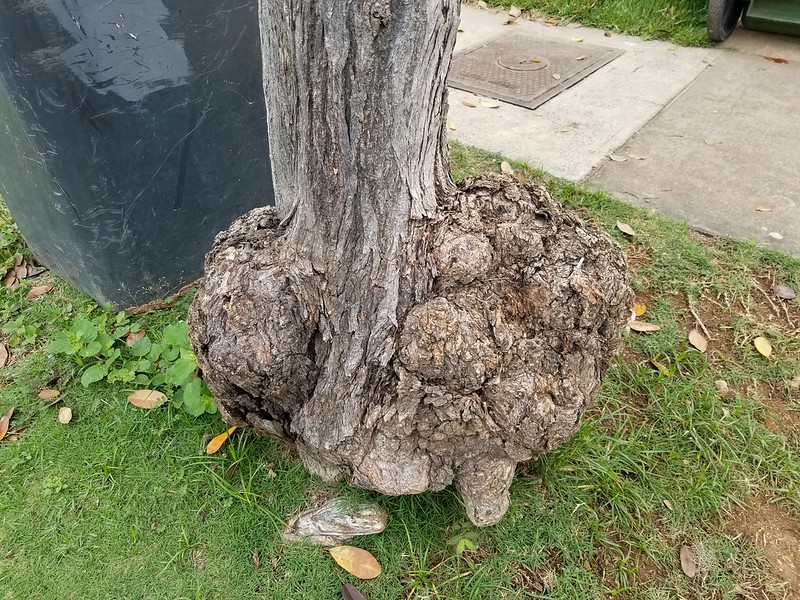
Scientists alters the process by which Agrobacterium infects and genetically alter plant cells to produce genetically modified plants with agriculturally beneficial traits as follows (figure \(\PageIndex{f}\)):
- T-DNA, which codes for the crown gall is removed from the Ti plasmid, and genes for desired traits are added.
- The modified plasmid is then added back to Agrobacterium.
- Agrobacterium infects undifferentiated plant cells (stem cells that can develop into any part of the plant; figure \(\PageIndex{g}\)).
- The modified plant cells are given hormones to produce the entire plant.
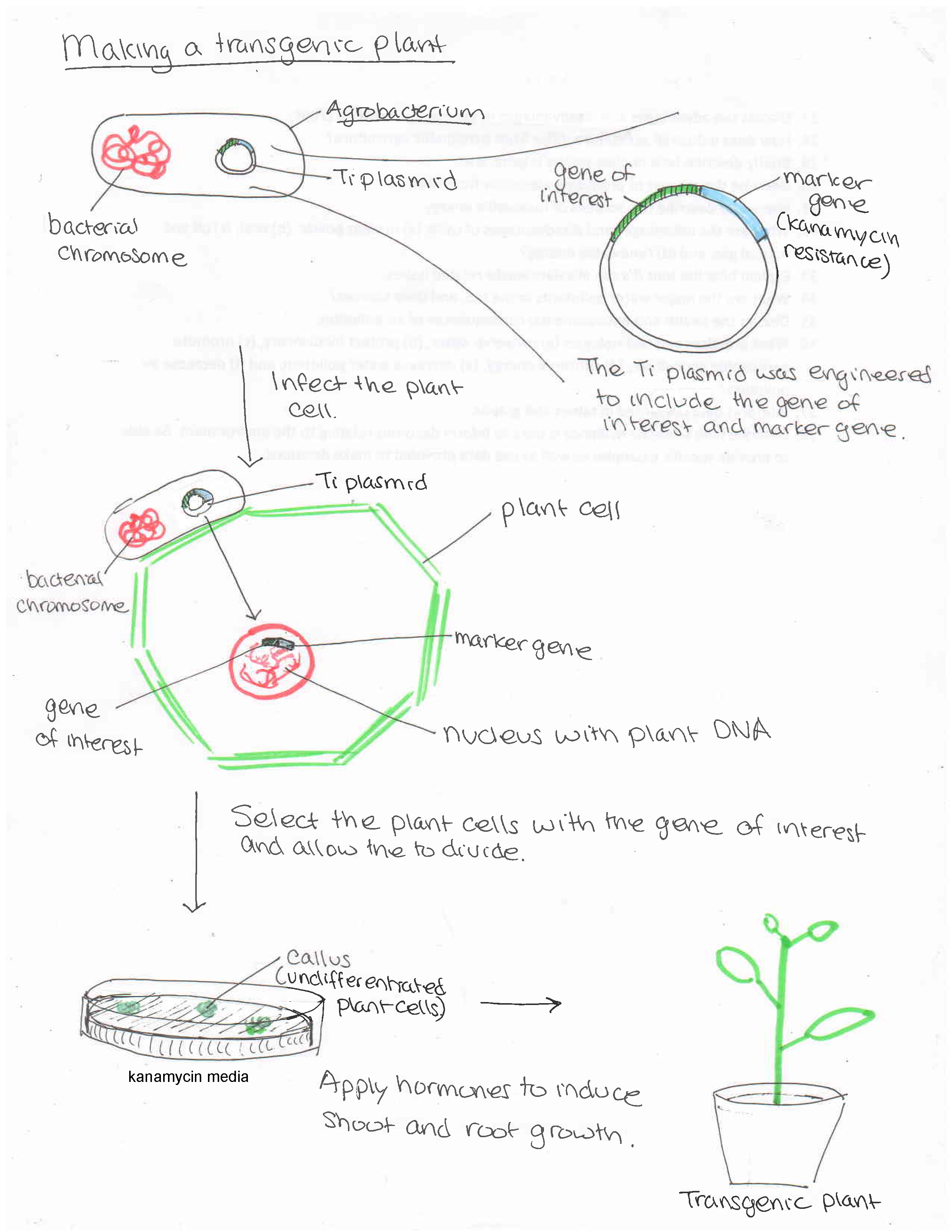
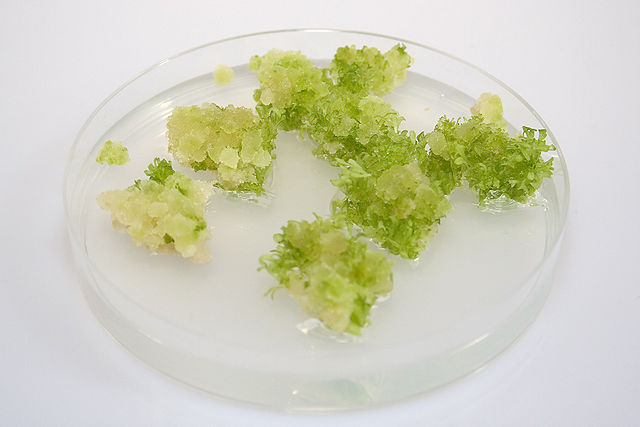
Examples of Genetically Modified Crops
Many genetically modified crops have been approved in the U.S. and produce our foods. The first genetically modified organism approved by the U.S. Food and Drug Administration (FDA) in 1994 was Flavr Savr™ tomatoes, which have a longer shelf life (delayed rotting) because a gene responsible for breaking down cells in inhibited. Flavr Savr tomatoes are genetically modified (because their DNA has been altered) but not trasgenic (because they do not contain genes from another species). The Flavr Savr tomato did not successfully stay in the market because of problems maintaining and shipping the crop. Golden rice produces β-carotene, a precursor to vitamin A (figure \(\PageIndex{h}\); β-carotene is also in high concentrations in carrots, sweet potatoes, and cantaloupe, giving them their orange color.) Roundup Ready® corn, cotton, and soybeans are resistant to this common herbicide, making it easier to uniformly spray it in a field to kill the weeds without harming the crops (figure \(\PageIndex{i}\)).
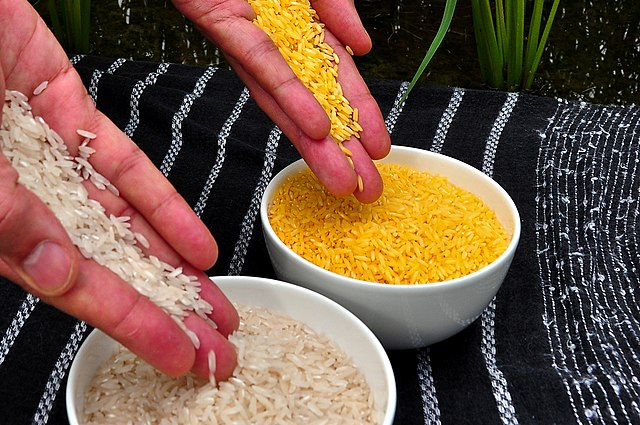
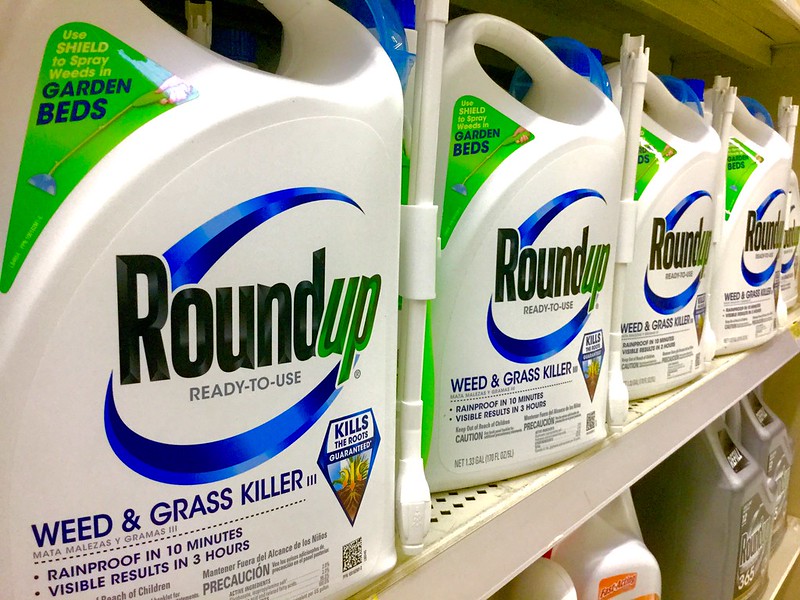
Crops have also been engineered to produce insecticides. Bacillus thuringiensis (Bt) is a bacterium that produces protein crystals that are toxic to many insect species that feed on plants. Insects that have eaten Bt toxin stop feeding on the plants within a few hours. After the toxin is activated in the intestines of the insects, death occurs within a couple of days. The gene to produce Bt toxin has been added to many crops including corn (figure \(\PageIndex{j}\)), potatoes, and cotton, providing plants with defense against insects.
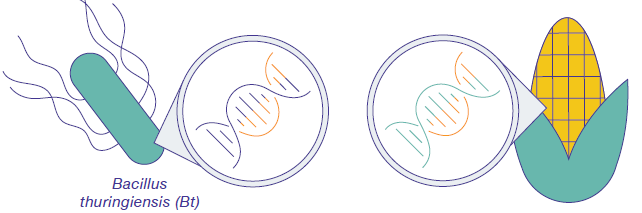
Genetically modified foods are widespread in the United States. For example, 94% of soy crops were genetically modified for herbicide resistance in 2020. Likewise, 8% of cotton and 10% of corn crops were modified for herbicide resistance in addition to the 83% of cotton and 79% of corn crops that were genetically modified in multiple ways.
Genetically modified animals have recently entered the market as well. AquaAdvantage® salmon are modified to grow more rapidly and were approved in November of 2015. However, as of March 2021, they have still not been sold due to legal challenges. In 2020, the FDA approved GalSafe™ pigs for medicine and food production. These pigs lack a molecule on the outside of their cells that cause allergies in some people.
Advantages of Genetically Modified Crops
Advances in biotechnology may provide consumers with foods that are nutritionally-enriched, longer-lasting, or that contain lower levels of certain naturally occurring toxins present in some food plants. For example, researchers are using biotechnology to try to reduce saturated fats in cooking oils and reduce allergens in foods. Whether these benefits will reach the people who need them most remains to be seen. While cultivating golden rice could address vitamin A deficiency in millions of people, it has not historically been accessible to these people because it is patented and expensive. Similarly, genetically modified seeds could increase the income of impoverished farmers if they were available at low or no cost, but this is not always the case.
Rainbow and SunUp papayas are a success story of how genetically modified crops can benefit small farmers and the economy in general. In the early 1990s, an emerging disease was destroying Hawaii’s production of papaya and threatening to decimate the $11-million industry (figure \(\PageIndex{k}\)). Fortunately, a man named Dennis Gonsalves (figure \(\PageIndex{l}\)), who was raised on a sugar plantation and then became a plant physiologist at Cornell University, would develop papaya plants genetically engineered to resist the deadly virus. By the end of the decade, the Hawaiian papaya industry and the livelihoods of many farmers were saved thanks to the free distribution of Dr. Gonsalves's seeds.
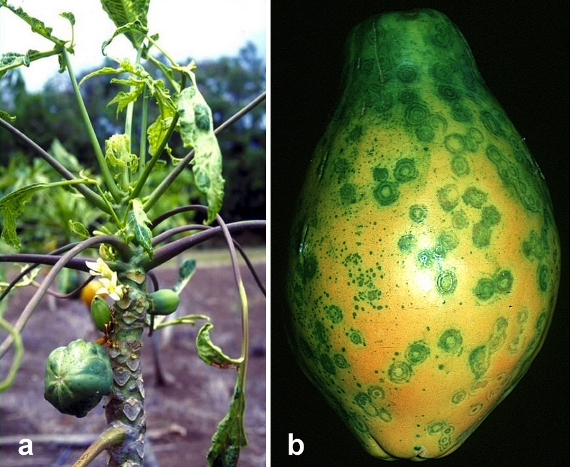
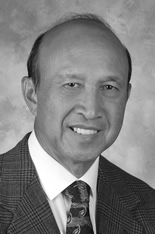
The effect of genetically modified crops on the environment depends on the specific genetic modification and which agricultural practices it promotes. For example, Bt crops produce their own insecticides such that external application of these chemicals is unnecessary, reducing the negative impacts of industrial agriculture. Ongoing research is exploring whether crops can be engineered to fix nitrogen in the atmosphere (as some bacteria do) rather than relying on ammonium, nitrites, and nitrates in the soil. If these crops were successfully engineered, they could reduce synthetic fertilizer application and minimize nutrient runoff that leads to eutrophication.
Genetically modified crops may have the potential to conserve natural resources, enable animals to more effectively use nutrients present in feed, and help meet the increasing world food and land demands. In practice, however, countries that use genetically modified crops compared to those that do not only enjoy a slight (or nonexistent) increase in yield.
Disadvantages of Genetically Modified Crops
Social Concerns
Intellectual property rights are one of the important factors in the current debate on genetically modified crops. Genetically modified crops can be patented by agribusinesses, which can lead to them controlling and potentially exploiting agricultural markets. Some accuse companies, such as Monsanto, of allegedly controlling seed production and pricing, much to the detriment of farmers (figure \(\PageIndex{m}\)).
Environmental Concerns
Genetically modified crops present several environmental concerns. Monoculture farming already reduces biodiversity, and cultivating genetically modified crops, for which individual plants are quite similar genetically, exacerbates this. The use of Roundup Ready® crops naturally encourages widespread herbicide use, which could unintentionally kill nearby native plants. This practice would also increase herbicide residues on produce. While Bt crops are beneficial in the sense that they do not require external insecticide application, but Bt toxin is spread in their pollen. An early study found that Bt corn pollen may be harmful to monarch caterpillars (figure \(\PageIndex{n}\)), but only at concentrations that are seldom reached in nature. Follow-up studies found that most of Bt corn grown did not harm monarchs; however, the one strain of Bt corn did was consequently removed from the market.
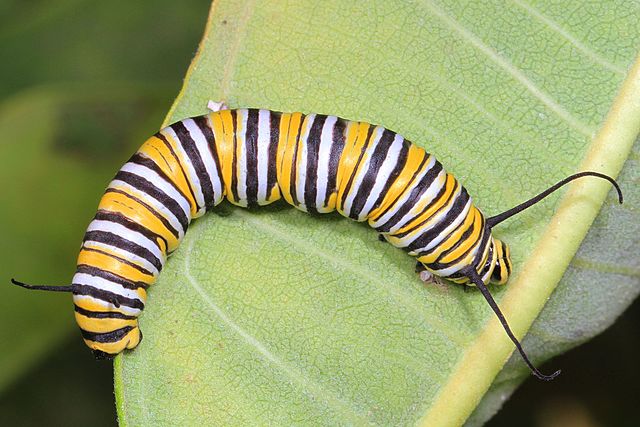
Through interbreeding, or hybridization, genetically modified crops might share their transgenes with wild relatives. This could affect the genetics of those wild relatives and have unforeseen consequences on their populations and could even have implications for the larger ecosystem. For example, if a gene engineered to confer herbicide resistance were to pass from a genetically modified crop to a wild relative, it might transform the wild species into a super weed – a species that could not be controlled by herbicide. Its rampant growth could then displace other wild species and the wildlife that depends on it, thus inflecting ecological harm.
Not only could escaped genes alter weedy species, but they could also enter populations of native species. This could make some native species better competitors than they were previously, disrupting ecosystem dynamics. (They could potentially outcompete other native species with which they would otherwise coexist.)
While there is evidence of genetic transfer between genetically modified crops and wild relatives, there is not yet evidence of ecological harm from that transfer. Clearly, continued monitoring, especially for newly-developed crops, is warranted.
The escape of genetically modified animals has potential to disrupt ecosystems as well. For example, if AquaAdvantage salmon were to escape into natural ecosystem, as farmed fish often do, they could outcompete native salmon, including endangered species. Their genetic modification, which facilitates rapid growth, could result in a competitive advantage.
Health Concerns
In addition to environmental risks, some people are concerned about potential health risks of genetically modified crops because they feel that genetic modification alters the intrinsic properties, or essence, of an organism. As discussed above, however, it is known that both traditional breeding practices and modern genetic engineering produce permanent genetic changes. Furthermore, selective breeding actually has a larger and more unpredictable impact on a species’s genetics because of its comparably crude nature.
To address these concerns (and others), the US National Academies of Sciences, Engineering, and Medicine (NASEM) published a comprehensive, 500-page report in 2016 that summarized the current scientific knowledge regarding genetically modified crops. The report, titled Genetically Engineered Crops: Experiences and Prospects, reviewed more than 900 research articles, in addition to public comments and expert testimony. NASEM’s GE Crop Report found “no substantiated evidence of a difference in risks to human health between current commercially available genetically engineered (GE) crops and conventionally bred crops, nor did it find conclusive cause-and-effect evidence of environmental problems from the GE crops.” Additionally, the UN’s Food and Agriculture Organization has concluded that risks to human and animal health from the use of GMOs are negligible. The scientific consensus on genetically modified crops is quite clear: they are safe for human consumption.
The potential of genetically modified crops to be allergenic is one of the potential adverse health effects, and it should continue to be studied, especially because some scientific evidence indicates that animals fed genetically modified crops have been harmed. NASEM’s GE Crop Report concluded that when developing new crops, it is the product that should be studied for potential health and environmental risks, not the process that achieved that product. What this means is, because both traditional breeding practices and modern genetic engineering produce new traits through genetic modification, they both present potential risks. Thus, for the safety of the environment and human health, both should be adequately studied.
Are Genetically Modified Crops the Solution We Need?
Significant resources, both financial and intellectual, have been allocated to answering the question: are genetically modified crops safe for human consumption? After many hundreds of scientific studies, the answer is yes. But a significant question still remains: are they necessary? Certainly, such as in instances like Hawaii’s papaya, which were threatened with eradication due to an aggressive disease, genetic engineering was a quick and effective solution that would have been extremely difficult, if not impossible, to solve using traditional breeding practices.
However, in many cases, the early promises of genetically engineered crops – that they would improve nutritional quality of foods, confer disease resistance, and provide unparalleled advances in crop yields – have largely failed to come to fruition. NASEM’s GE Crop Report states that while genetically modified crops have resulted in the reduction of agricultural loss from pests, reduced pesticide use, and reduced rates of injury from insecticides for farm workers, they have not increased the rate at which crop yields are advancing when compared to non-GE crops. Additionally, while there are some notable exceptions like golden rice or virus-resistant papayas, very few genetically engineered crops have been produced to increase nutritional capacity or to prevent plant disease that can devastate a farmer’s income and reduce food security. The vast majority of genetically modified crops are developed for only two purposes: to introduce herbicide resistance or pest resistance. Genetically modified crops are concentrated in developed countries, and their availability in developing countries, where they are perhaps most needed, is limited (figure \(\PageIndex{o}\)).
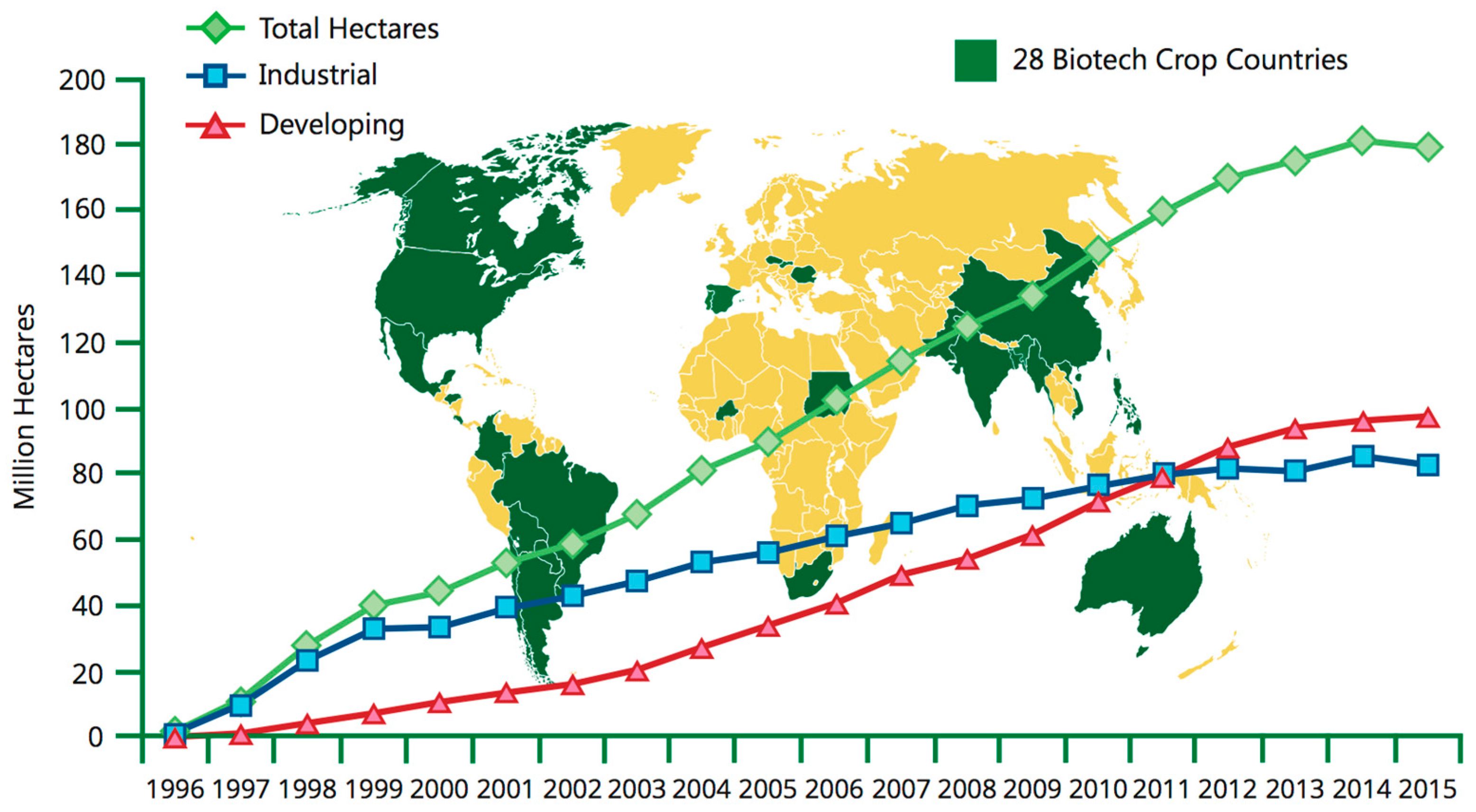
Suggested Supplementary Reading
NASEM. 2016. Genetically Engineered Crops: Experiences and Prospects.
Attributions
Modified by Melissa Ha from the following sources:
- Biotechnology and Genetic Engineering from Environmental Biology by Matthew R. Fisher (licensed under CC-BY)
- Biotechnology in Medicine and Agriculture from Concepts in Biology by OpenStax (licensed under CC-BY)