3.3: X-inactivation
( \newcommand{\kernel}{\mathrm{null}\,}\)
Introduction
Having extra or missing chromosomes is not usually a good thing. In fact, for most chromosomes, having an extra or missing copy is lethal to humans (causing an embryo to die early in development).
Yet, human females have two X chromosomes (XX), while human males have just one (XY). Why doesn't it cause problems for men to have just one copy of the X chromosome, while women have two?
X-inactivation
As it turns out, the level of gene activity produced by a single X chromosome is the normal "dosage" for a human. Men have this dosage because, well, they only have one X chromosome! Women have the same dosage for a different reason: they shut down one of their two X chromosomes in a process called X-inactivation.
In X-inactivation, an X chromosome is compacted (or, as my intro bio professor liked to say, "crumpled up into a ball"), to make a small, dense structure called a Barr body. Most of the genes on the Barr body are inactive, meaning that they are not transcribed. The process of X-inactivation was discovered by the British geneticist Mary F. Lyon and is sometimes called lyonization in her honor1.
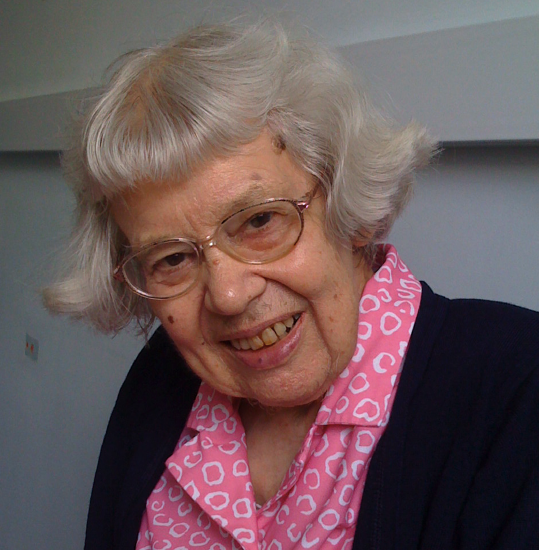
A woman has two X chromosomes, one from each parent. Which one will she inactivate? X-inactivation is a random process that happens separately in individual cells during embryonic development. One cell might shut down the paternal X, while its next-door neighbor might shut down the maternal X instead. All the cells descended from each of these original cells will maintain the same pattern of X-inactivation.
Interesting note: if you were a kangaroo, what I just said would not be true! In kangaroos and other marsupials, it is always the paternal X chromosome that undergoes X-inactivation2.
X-inactivation example: Calico cat
A classic example of X-inactivation is seen in cats. If a female cat is heterozygous for black and tan alleles of a coat color gene found on the X, she will inactivate her two Xs (and thus, the two alleles of the coat color gene) at random in different cells during development.
The result is a tortoiseshell coat pattern, made up of alternating patches of black and tan fur. The black patches come from groups of cells in which the X with the black allele is active, while the tan patches come from cells in which the X with the tan allele is active.
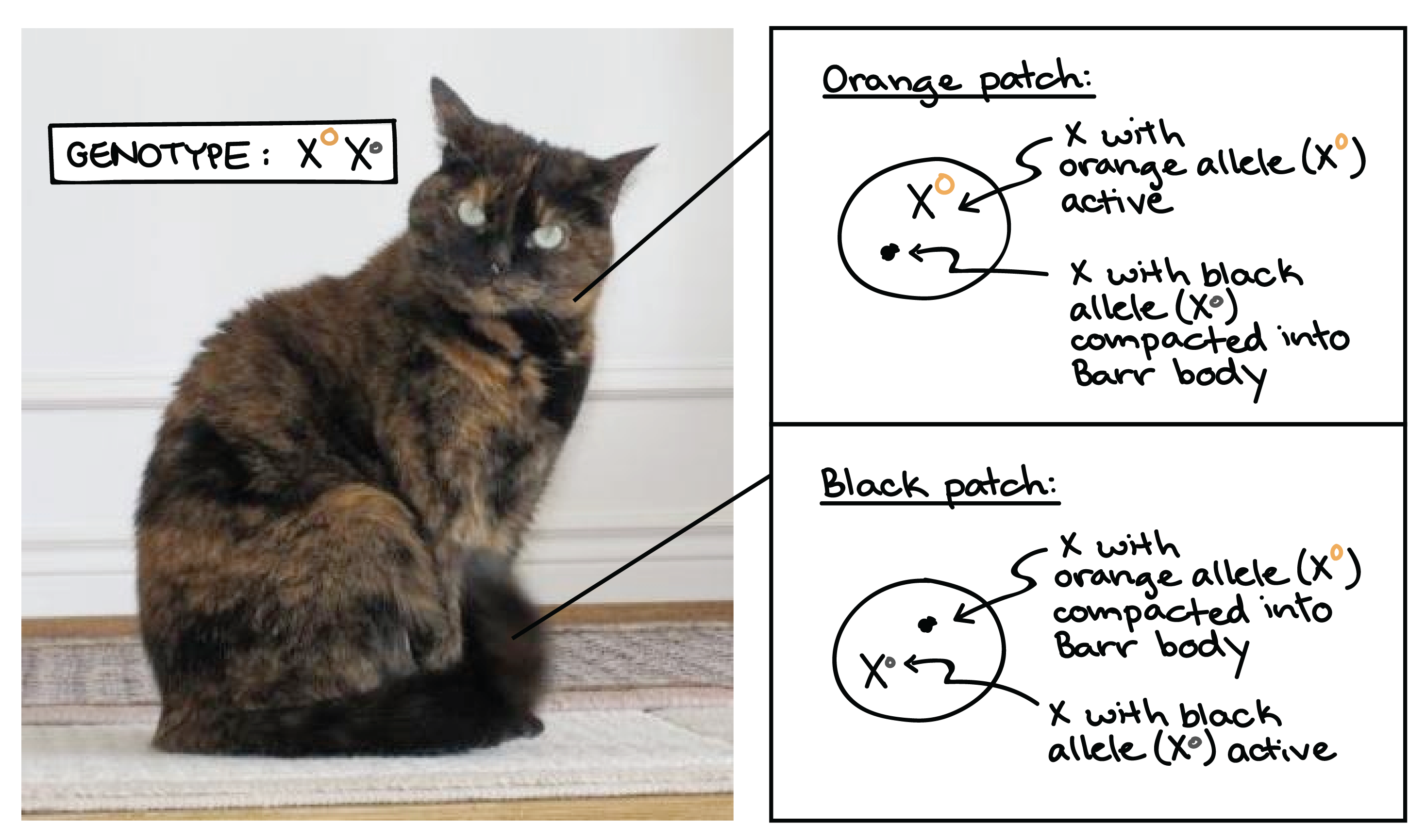
Although it's rarely as easy to see as in the case of the tortoiseshell cat, human females are also "mosaic" for any genes that are present in different alleles on their two X chromosomes.
- [If that's true, why don't female carriers show X-linked disorders?]
-
At this point, you may be wondering why women heterozygous for a recessive, X-linked allele don’t display the associated condition. After all, roughly half of their cells will inactivate the normal allele, leaving only the disorder-causing allele active.
In many cases, it appears that having 50% of cells with a normal copy of a gene is sufficient to produce a normal, or close to normal, phenotype.
A good example of this comes from the X-linked condition of red-green color vision deficiency (colorblindness). Women who are carriers for red-green color vision deficiency alleles have a mixture of working and non-working photoreceptor cells in their eyes. However, the working cells allow them to perceive red and green well enough to function normally in daily life. In more sensitive tests in a laboratory setting, these women actually do show subtle color vision deficiencies relative to women who are not carriers3.
Sex chromosome aneuploidies
When an organism has an extra or missing copy of a chromosome, it is said to be aneuploid. Aneuploidies involving autosomes (non-sex chromosomes), especially large ones, are usually so harmful to development that an aneuploid embryo can't survive to birth.
Aneuploidies of X chromosomes, however, tend to be much less harmful, despite the fact that the X is a large chromosome. This is mostly thanks to X inactivation. Although the purpose of the X-inactivation system is to shut down the second X of an XX female, it can also do a pretty good job of shutting down more X chromosomes if they are present.
Examples of X chromosome aneuploidies include:
- Triple X syndrome, in which a woman has an XXX genotype, which occurs in about 1 out of every 1,000 female newborns4. Women with an XXX genotype have female sex characteristics and are fertile (able to have children). In some cases, triple X syndrome may be associated with learning difficulties, late development of motor skills in infants, and problems with muscle tone4.
- Klinefelter syndrome, in which males have an extra X chromosome, leading to a genotype of XXY. (In rarer cases, Klinefelter syndrome can involve several extra Xs, leading to an XXXY or XXXXY genotype.) Affected men may be infertile or develop less dense body and facial hair than other men. Klinefelter syndrome is thought to affect 1 out of every 500 to 1,000 male newborns5.
Like females, XXY males with Klinefelter syndrome will convert one X to a Barr body in each cell. Triple X females (as well as Klinefelter males with more than two X chromosomes) neutralize their extra Xs by forming additional Barr bodies. For example, there would be two Barr bodies in a cell from an XXX female or XXXY male.
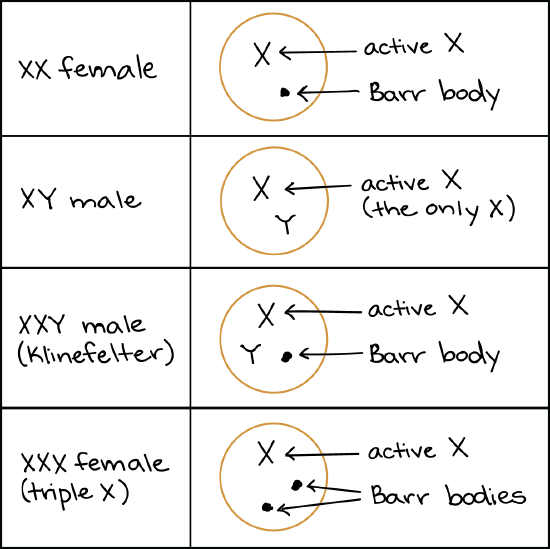
In Turner syndrome, a woman lacks part or all of one of her X chromosomes (leaving her with just one functional X). People with this disorder develop as females, but often have short stature and may exhibit symptoms like infertility and learning difficulties. Turner syndrome is thought to occur in about 1 out of every 2,500 female births6. It has relatively mild effects because humans normally have only one X active in the cells of their body anyway.
Check your understanding
Query \PageIndex{1}
- [Hint]
-
Tortoiseshell cats typically have a pattern of mixed black and orange patches on their coats as a result of X-inactivation. In cats, a gene borne on the X chromosome controls coat color, and heterozygous female cats with one orange allele (\text{X}^O) and one black allele (\text{X}^o) will randomly inactivate one of the two X chromosomes in each cell during embryonic development. All the cells descended from each of these early cells will retain the same inactivation state, giving rise to patches of cells with the same active X and, thus, the same active gene for coat pigmentation.
Normally, male cats do not show a tortoiseshell coat pattern because they have only one X chromosome, and thus do not carry out X-inactivation. Instead, they simply display the solid coat color corresponding to the allele of the coat color gene found on their single X (with \text{X}^O giving orange and \text{X}^o giving black). However, the X-inactivation system is still present in males, even though they don't typically use it, and can come into play if a male receives an unusually high number of X chromosomes.
It's possible to get a tortoiseshell pattern in a male cat if the cat receives two X chromosomes as well as a Y, and if his two Xs bear different alleles of the coat color gene (genotype \text{X}^O\text{X}^o\text{Y}). An XXY genotype can arise through nondisjunction, the failure of sex chromosomes to separate correctly from one another during meiosis. One example of how nondisjunction could lead to an XXY genotype and a tortoiseshell phenotype is shown in the Punnett square below.
In cats (as in humans), male development is specified by the SRY gene on the Y chromosome, so an XXY individual will develop as male. However, only one X can be active in each cell, so one of the two X chromosomes will be randomly inactivated in each of the XXY male's cells during early embryonic development. This results in a patchy tortoiseshell coat pattern like that of a heterozygous female cat.
Male tortoiseshells with an XXY genotype are outwardly male, but are usually sterile (unable to have offspring), with a feline version of the disorder known as Klinefelter syndrome in humans. Not all male tortoiseshell kittens have an abnormal number of X chromosomes, however. Instead, some are chimeras, or genetic mosaics, that result from the fusion of two embryos into one during early development. If the two embryos that fuse have different alleles of the coat color gene, the chimeric embryo will have a mixture of cells with different genotypes, and the mature cat will have patchy fur similar to that of a female tortoiseshell.
Contributors and Attributions
Khan Academy (CC BY-NC-SA 3.0; All Khan Academy content is available for free at www.khanacademy.org)
- [Attribution and references]
-
Attribution:
This article is a modified derivative of "Chromosomal basis of inherited disorders," by OpenStax College, Biology, CC BY 4.0. Download the original article for free at http://cnx.org/contents/185cbf87-c72e-48f5-b51e-f14f21b5eabd@10.53.
This article is licensed under a CC BY-NC-SA 4.0 license.
Works cited:
-
Mary F. Lyon. (2015, November 22). Retrieved December 12, 2015 from Wikipedia: https://en.wikipedia.org/wiki/Mary_F._Lyon.
-
Kimball, J. W. (2015, December 23). Sex chromosomes. In Kimball's biology pages. Retrieved from http://www.biology-pages.info/S/SexChromosomes.html.
-
Chen, Shuai. (2010, March 4). Since only one of a woman's X chromosomes works in a cell, why aren't more women colorblind? [answer]. In Stanford at the Tech: Understanding genetics. Retrieved from http://genetics.thetech.org/ask/ask349.
-
Triple X syndrome. (2014). In Genetics home reference. Retrieved from http://ghr.nlm.nih.gov/condition/triple-x-syndrome.
-
Klinefelter syndrome. (2013). In Genetics home reference. Retrieved from http://ghr.nlm.nih.gov/condition/klinefelter-syndrome.
-
Turner syndrome. (2012). In Genetics home reference. Retrieved from http://ghr.nlm.nih.gov/condition/turner-syndrome.
Additional references:
Bergmann, D. C. (2011). Mutations, aneuploidy and sex determination. In Genetics lecture notes (pp. 36-44). Biosci41, Stanford University.
Chen, Shuai. (2010, March 4). Since only one of a woman's X chromosomes works in a cell, why aren't more women colorblind? [answer]. In Stanford at the Tech: Understanding genetics. Retrieved from http://genetics.thetech.org/ask/ask349.
Deeb, S. S. and Motulsky, A. G. (2015, February 5). Red-green color vision defects. In GeneReviews. Retrieved from http://www.ncbi.nlm.nih.gov/books/NBK1301/.
Genetic factors and hormones that determine gender. (2007, June 27). In Human embryology: Organogenesis. Retrieved from http://www.embryology.ch/anglais/ugenital/molec02.html.
Kimball, J. W. (2015, December 23). Sex chromosomes. In Kimball's biology pages. Retrieved from http://www.biology-pages.info/S/SexChromosomes.html.
Klinefelter syndrome. (2013). In Genetics home reference. Retrieved from http://ghr.nlm.nih.gov/condition/klinefelter-syndrome.
Krempels, D. M. (n.d.). The genetics of calico cats. Retrieved from www.bio.miami.edu/dana/dox/calico.html.
Mary F. Lyon. (2015, November 22). Retrieved December 12, 2015 from Wikipedia: https://en.wikipedia.org/wiki/Mary_F._Lyon.
OpenStax College, Biology. (2015, May 13). Chromosomal theory and genetic linkage. In OpenStax CNX. Retrieved from http://cnx.org/contents/185cbf87-c72e-48f5-b51e-f14f21b5eabd@9.85:64/Chromosomal-Theory-and-Genetic
O'Neil, Dennis. (2013). Sex chromosome abnormalities. In Human chromosomal abnormalities. Retrieved from http://anthro.palomar.edu/abnormal/abnormal_5.htm.
OPN1LW. (2015). In Genetics home reference. Retrieved from http://ghr.nlm.nih.gov/gene/OPN1LW.
Purves, W. K., Sadava, D. E., Orians, G. H., and Heller, H.C. (2003). Sex determination and sex-linked inheritance. In Life: The science of biology (7th ed., pp. 125-144). Sunderland, MA: Sinauer Associates.
Reece, J. B., Urry, L. A., Cain, M. L., Wasserman, S. A., Minorsky, P. V., and Jackson, R. B. (2011). Alterations of chromosome number or structure cause some genetic disorders. In Campbell biology (10th ed., pp. 304-307). San Francisco, CA: Pearson.
Reece, J. B., Urry, L. A., Cain, M. L., Wasserman, S. A., Minorsky, P. V., and Jackson, R. B. (2011). Sex-linked genes exhibit unique patterns of inheritance. In Campbell biology (10th ed., pp. 205-209). San Francisco, CA: Pearson.
Testis determining factor. (2015, November 10). Retrieved December 11, 2015 from Wikipedia: https://en.wikipedia.org/wiki/Testis_determining_factor.
Triple X syndrome. (2014). In Genetics home reference. Retrieved from http://ghr.nlm.nih.gov/condition/triple-x-syndrome.
Turner syndrome. (2012). In Genetics home reference. Retrieved from http://ghr.nlm.nih.gov/condition/turner-syndrome.
XY sex-determination system. (2015, November 19). Retrieved December 11, 2015 from Wikipedia: https://en.wikipedia.org/wiki/XY_sex-determination_system.
-