3.2: X-linked inheritance
- Page ID
- 73540
\( \newcommand{\vecs}[1]{\overset { \scriptstyle \rightharpoonup} {\mathbf{#1}} } \)
\( \newcommand{\vecd}[1]{\overset{-\!-\!\rightharpoonup}{\vphantom{a}\smash {#1}}} \)
\( \newcommand{\id}{\mathrm{id}}\) \( \newcommand{\Span}{\mathrm{span}}\)
( \newcommand{\kernel}{\mathrm{null}\,}\) \( \newcommand{\range}{\mathrm{range}\,}\)
\( \newcommand{\RealPart}{\mathrm{Re}}\) \( \newcommand{\ImaginaryPart}{\mathrm{Im}}\)
\( \newcommand{\Argument}{\mathrm{Arg}}\) \( \newcommand{\norm}[1]{\| #1 \|}\)
\( \newcommand{\inner}[2]{\langle #1, #2 \rangle}\)
\( \newcommand{\Span}{\mathrm{span}}\)
\( \newcommand{\id}{\mathrm{id}}\)
\( \newcommand{\Span}{\mathrm{span}}\)
\( \newcommand{\kernel}{\mathrm{null}\,}\)
\( \newcommand{\range}{\mathrm{range}\,}\)
\( \newcommand{\RealPart}{\mathrm{Re}}\)
\( \newcommand{\ImaginaryPart}{\mathrm{Im}}\)
\( \newcommand{\Argument}{\mathrm{Arg}}\)
\( \newcommand{\norm}[1]{\| #1 \|}\)
\( \newcommand{\inner}[2]{\langle #1, #2 \rangle}\)
\( \newcommand{\Span}{\mathrm{span}}\) \( \newcommand{\AA}{\unicode[.8,0]{x212B}}\)
\( \newcommand{\vectorA}[1]{\vec{#1}} % arrow\)
\( \newcommand{\vectorAt}[1]{\vec{\text{#1}}} % arrow\)
\( \newcommand{\vectorB}[1]{\overset { \scriptstyle \rightharpoonup} {\mathbf{#1}} } \)
\( \newcommand{\vectorC}[1]{\textbf{#1}} \)
\( \newcommand{\vectorD}[1]{\overrightarrow{#1}} \)
\( \newcommand{\vectorDt}[1]{\overrightarrow{\text{#1}}} \)
\( \newcommand{\vectE}[1]{\overset{-\!-\!\rightharpoonup}{\vphantom{a}\smash{\mathbf {#1}}}} \)
\( \newcommand{\vecs}[1]{\overset { \scriptstyle \rightharpoonup} {\mathbf{#1}} } \)
\( \newcommand{\vecd}[1]{\overset{-\!-\!\rightharpoonup}{\vphantom{a}\smash {#1}}} \)
\(\newcommand{\avec}{\mathbf a}\) \(\newcommand{\bvec}{\mathbf b}\) \(\newcommand{\cvec}{\mathbf c}\) \(\newcommand{\dvec}{\mathbf d}\) \(\newcommand{\dtil}{\widetilde{\mathbf d}}\) \(\newcommand{\evec}{\mathbf e}\) \(\newcommand{\fvec}{\mathbf f}\) \(\newcommand{\nvec}{\mathbf n}\) \(\newcommand{\pvec}{\mathbf p}\) \(\newcommand{\qvec}{\mathbf q}\) \(\newcommand{\svec}{\mathbf s}\) \(\newcommand{\tvec}{\mathbf t}\) \(\newcommand{\uvec}{\mathbf u}\) \(\newcommand{\vvec}{\mathbf v}\) \(\newcommand{\wvec}{\mathbf w}\) \(\newcommand{\xvec}{\mathbf x}\) \(\newcommand{\yvec}{\mathbf y}\) \(\newcommand{\zvec}{\mathbf z}\) \(\newcommand{\rvec}{\mathbf r}\) \(\newcommand{\mvec}{\mathbf m}\) \(\newcommand{\zerovec}{\mathbf 0}\) \(\newcommand{\onevec}{\mathbf 1}\) \(\newcommand{\real}{\mathbb R}\) \(\newcommand{\twovec}[2]{\left[\begin{array}{r}#1 \\ #2 \end{array}\right]}\) \(\newcommand{\ctwovec}[2]{\left[\begin{array}{c}#1 \\ #2 \end{array}\right]}\) \(\newcommand{\threevec}[3]{\left[\begin{array}{r}#1 \\ #2 \\ #3 \end{array}\right]}\) \(\newcommand{\cthreevec}[3]{\left[\begin{array}{c}#1 \\ #2 \\ #3 \end{array}\right]}\) \(\newcommand{\fourvec}[4]{\left[\begin{array}{r}#1 \\ #2 \\ #3 \\ #4 \end{array}\right]}\) \(\newcommand{\cfourvec}[4]{\left[\begin{array}{c}#1 \\ #2 \\ #3 \\ #4 \end{array}\right]}\) \(\newcommand{\fivevec}[5]{\left[\begin{array}{r}#1 \\ #2 \\ #3 \\ #4 \\ #5 \\ \end{array}\right]}\) \(\newcommand{\cfivevec}[5]{\left[\begin{array}{c}#1 \\ #2 \\ #3 \\ #4 \\ #5 \\ \end{array}\right]}\) \(\newcommand{\mattwo}[4]{\left[\begin{array}{rr}#1 \amp #2 \\ #3 \amp #4 \\ \end{array}\right]}\) \(\newcommand{\laspan}[1]{\text{Span}\{#1\}}\) \(\newcommand{\bcal}{\cal B}\) \(\newcommand{\ccal}{\cal C}\) \(\newcommand{\scal}{\cal S}\) \(\newcommand{\wcal}{\cal W}\) \(\newcommand{\ecal}{\cal E}\) \(\newcommand{\coords}[2]{\left\{#1\right\}_{#2}}\) \(\newcommand{\gray}[1]{\color{gray}{#1}}\) \(\newcommand{\lgray}[1]{\color{lightgray}{#1}}\) \(\newcommand{\rank}{\operatorname{rank}}\) \(\newcommand{\row}{\text{Row}}\) \(\newcommand{\col}{\text{Col}}\) \(\renewcommand{\row}{\text{Row}}\) \(\newcommand{\nul}{\text{Nul}}\) \(\newcommand{\var}{\text{Var}}\) \(\newcommand{\corr}{\text{corr}}\) \(\newcommand{\len}[1]{\left|#1\right|}\) \(\newcommand{\bbar}{\overline{\bvec}}\) \(\newcommand{\bhat}{\widehat{\bvec}}\) \(\newcommand{\bperp}{\bvec^\perp}\) \(\newcommand{\xhat}{\widehat{\xvec}}\) \(\newcommand{\vhat}{\widehat{\vvec}}\) \(\newcommand{\uhat}{\widehat{\uvec}}\) \(\newcommand{\what}{\widehat{\wvec}}\) \(\newcommand{\Sighat}{\widehat{\Sigma}}\) \(\newcommand{\lt}{<}\) \(\newcommand{\gt}{>}\) \(\newcommand{\amp}{&}\) \(\definecolor{fillinmathshade}{gray}{0.9}\)Key points:
- In humans and other mammals, biological sex is determined by a pair of sex chromosomes: XY in males and XX in females.
- Genes on the X chromosome are said to be X-linked. X-linked genes have distinctive inheritance patterns because they are present in different numbers in females (XX) and males (XY).
- X-linked human genetic disorders are much more common in males than in females due to the X-linked inheritance pattern.
Introduction
If you’re a human being (which seems like a good bet!), most of your chromosomes come in homologous pairs. The two chromosomes of a homologous pair contain the same basic information – that is, the same genes in the same order – but may carry different versions of those genes.
Are all of your chromosomes organized in homologous pairs? The answer depends on whether you’re (chromosomally) male.
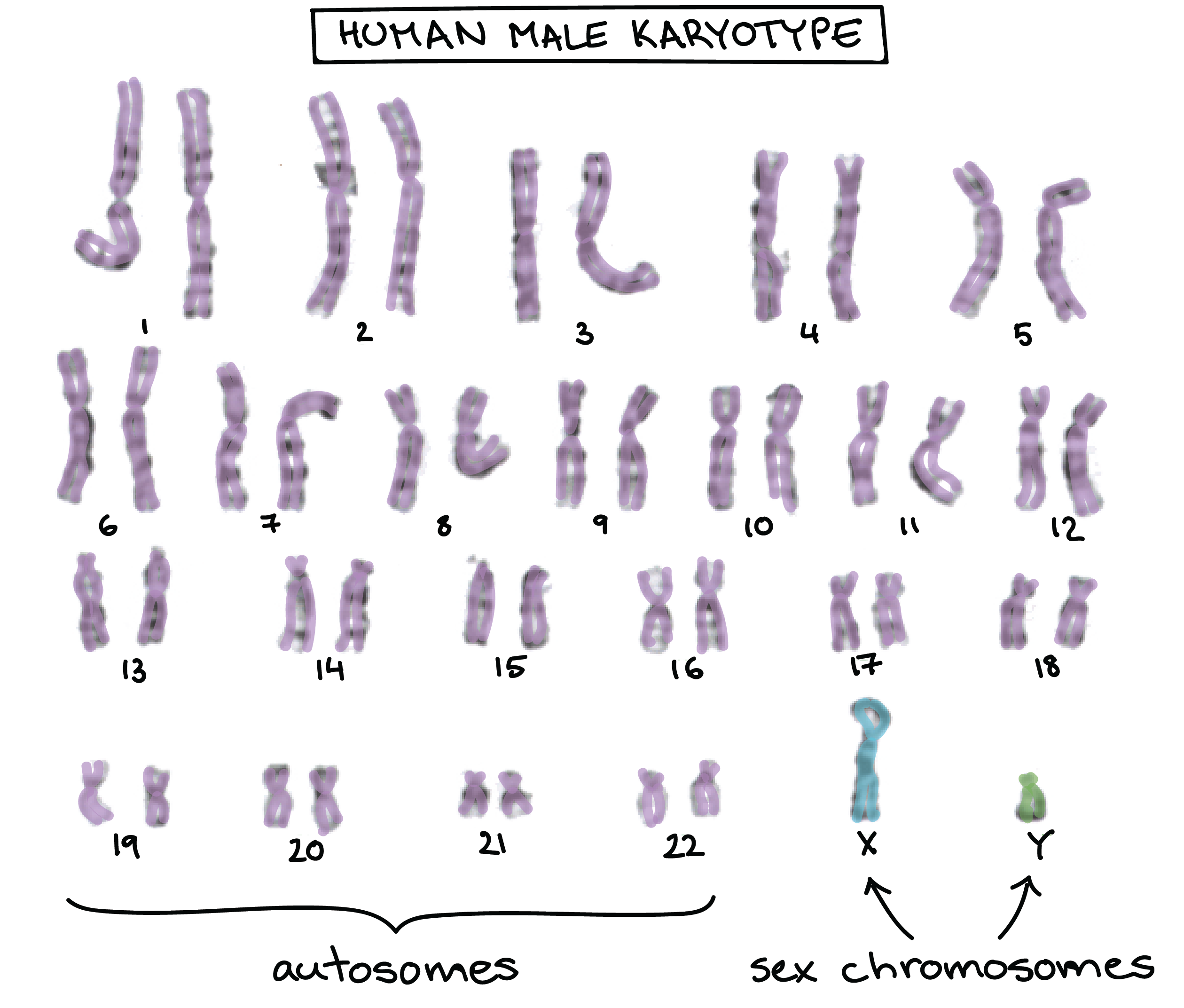
- A human male has two sex chromosomes, the X and the Y. Unlike the 44 autosomes (non-sex chromosomes), the X and Y don’t carry the same genes and aren’t considered homologous.
- Instead of an X and a Y, a human female has two X chromosomes. These X chromosomes do form a bona fide homologous pair.
Because sex chromosomes don’t always come in homologous pairs, the genes they carry show unique, distinctive patterns of inheritance.
Sex chromosomes in humans
Human X and Y chromosomes determine the biological sex of a person, with XX specifying female and XY specifying male. Although the Y chromosome contains a small region of similarity to the X chromosome so that they can pair during meiosis, the Y chromosome is much shorter and contains many fewer genes.
To put some numbers to it, the X chromosome has about 800-900 protein-coding genes with a wide variety of functions, while the Y chromosome has just 60-70 protein-coding genes, about half of which are active only in the testes (sperm-producing organs)1,2,3,4.
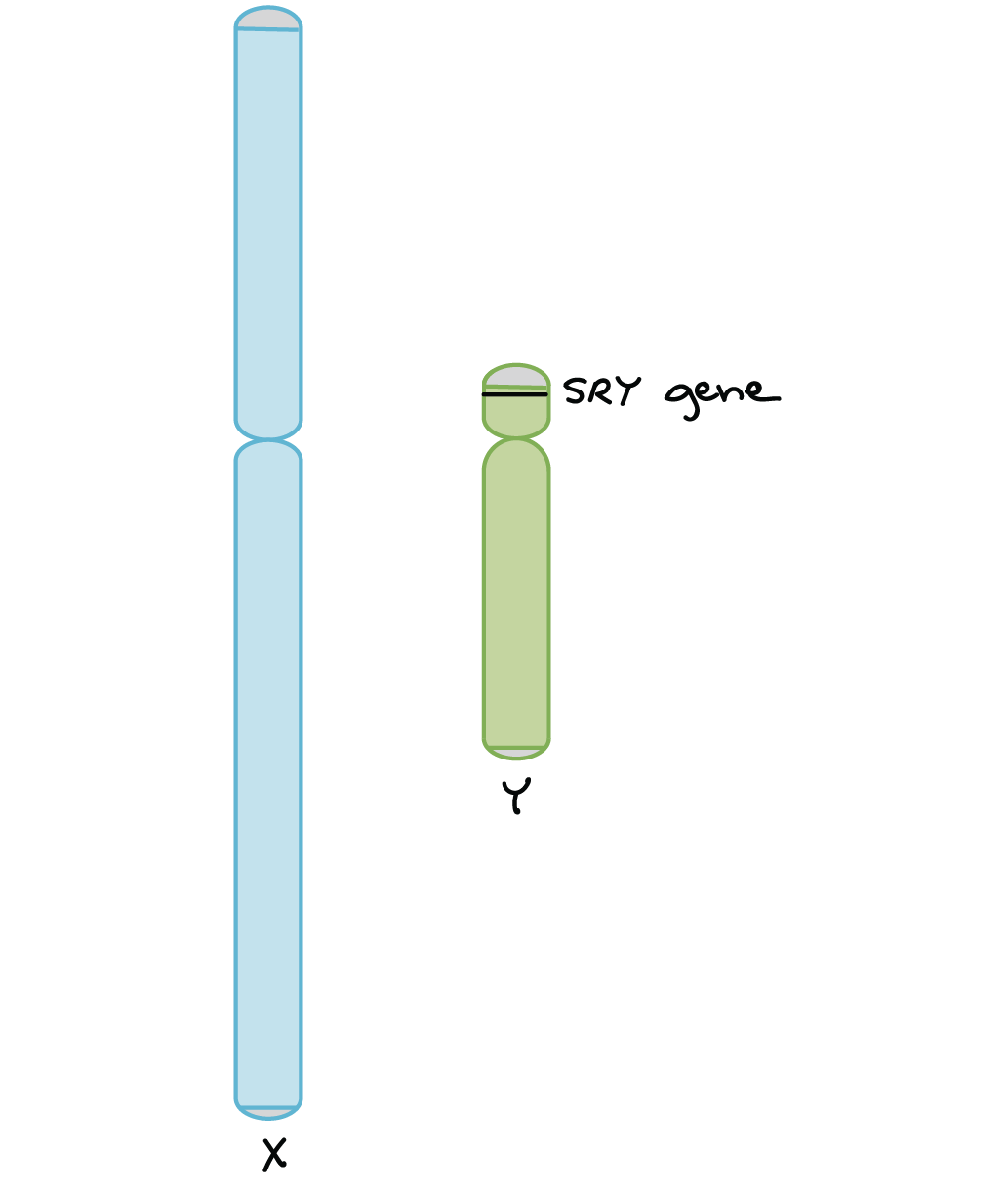
The human Y chromosome plays a key role in determining the sex of a developing embryo. This is mostly due to a gene called SRY (“sex-determining region of Y”). SRY is found on the Y chromosome and encodes a protein that turns on other genes required for male development5,6.
- XX embryos don't have SRY, so they develop as female.
- XY embryos do have SRY, so they develop as male.
In rare cases, errors during meiosis may transfer SRY from the Y chromosome to the X chromosome. If an SRY-bearing X chromosome fertilizes a normal egg, it will produce a chromosomally female (XX) embryo that develops as a male7. If an SRY-deficient Y chromosome fertilizes a normal egg, it will produce a chromosomally male embryo (XY) that develops as a female8.
- [Do all species with X and Y chromosomes have the SRY gene?]
-
No, not all of them. The molecular mechanisms of sex determination vary greatly among species, even those that use an X-Y sex determination system. In most placental mammals, SRY is found on the Y chromosome and is used for sex determination. However, the SRY gene is not present in other species with X-Y sex determination systems, such as fruit flies and other insects9.
X and Y chromosomes have evolved independently many times
To understand how this is possible, it's useful to keep in mind that “X” and “Y” are just generic labels applied to the dimorphic (di- = two, -morphe = form), or dissimilar, chromosomes found in species with X-Y sex determination systems10. X is whatever chromosome the female has two of, while Y is the chromosome paired with a single X in the male. The nature of the chromosomes — what genes are on them, and how they determine maleness or femaleness — may be quite different between species.
Sex chromosomes have evolved independently many times11. Thus, although fruit flies and humans both have X and Y chromosomes that match the above definition, and although XY and XX chromosome combinations correspond to maleness and femaleness, respectively, in both species, the sex determination mechanism in flies is very different from that of humans.
Example: Humans vs. fruit flies
In humans, as described above, the Y chromosome specifies maleness through the action of the SRY gene, a master regulator of male development.
In flies, the presence of a single X chromosome specifies maleness, while the presence of two Xs specifies femaleness, regardless of the presence or absence of a Y chromosome. (More specifically, it appears that sex is determined by the ratio of X chromosomes to autosomes, with a 1:2 ratio specifying maleness and a 2:2 ratio specifying femaleness. These ratios are "measured" through the levels of proteins produced by specific genes on the X chromosome and on autosomes.)12
Because of these differences in sex determination mechanisms, an XXY fly will develop as a female (see article on Thomas Hunt Morgan's experiments), while an XXY human will develop as a male (in a condition known as Klinefelter syndrome; see article on large-scale chromosomal changes).
X-linked genes
When a gene is present on the X chromosome, but not on the Y chromosome, it is said to be X-linked. X-linked genes have different inheritance patterns than genes on non-sex chromosomes (autosomes). That's because these genes are present in different copy numbers in males and females.
- [What about genes on the Y?]
-
Genes on either the X or the Y have unusual inheritance patterns and are called sex-linked, but X-linkage is much more common than Y-linkage (since there are many more genes on the X than the Y).
Since a female has two X chromosomes, she will have two copies of each X-linked gene. For instance, in the fruit fly Drosophila (which, like humans, has XX females and XY males), there is an eye color gene called white that's found on the X chromosome, and a female fly will have two copies of this gene. If the gene comes in two different alleles, such as \(\text{X}^W\) (dominant, normal red eyes) and \(\text{X}^w\) (recessive, white eyes), the female fly may have any of three genotypes: \(\text{X}^W\text{X}^W\) (red eyes), \(\text{X}^W\text{X}^w\) (red eyes), and \(\text{X}^w\text{X}^w\) (white eyes).
A male has different genotype possibilities than a female. Since he has only one X chromosome (paired with a Y), he will have only one copy of any X-linked genes. For instance, in the fly eye color example, the two genotypes a male can have are \(\text{X}^W\text{Y}\) (red eyes) and \(\text{X}^w\text{Y}\) (white eyes). Whatever allele the male fly inherits for an X-linked gene will determine his appearance, because he has no other gene copy—even if the allele is recessive in females. Rather than homozygous or heterozygous, males are said to be hemizygous for X-linked genes.
We can see how sex linkage affects inheritance patterns by considering a cross between two flies, a white-eyed female (\(\text{X}^w\text{X}^w\)) and a red-eyed male (\(\text{X}^W\text{Y}\)). If this gene were on a non-sex chromosome, or autosome, we would expect all of the offspring to be red-eyed, because the red allele is dominant to the white allele. What we actually see is the following:
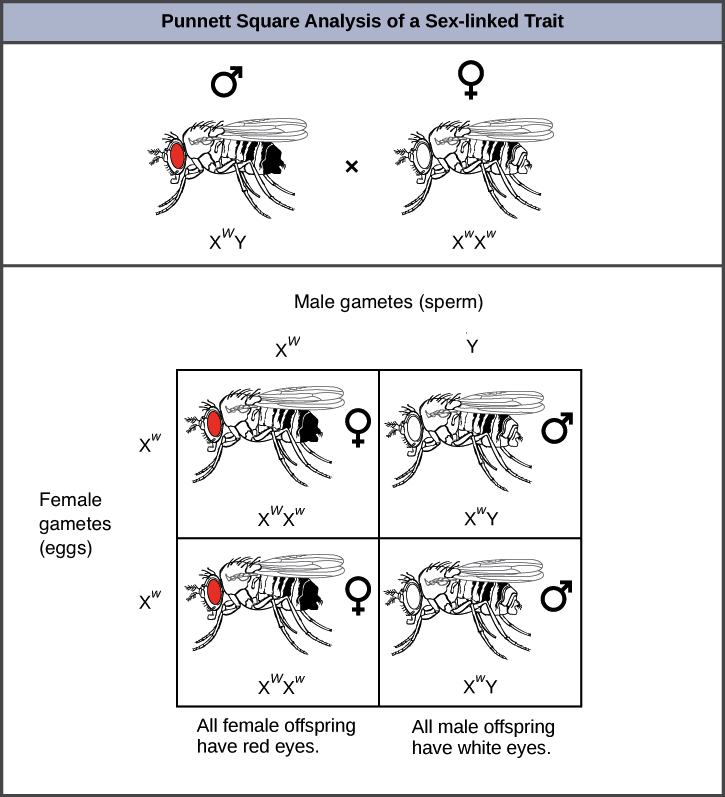
However, because the gene is X-linked, and because it was the female parent who had the recessive phenotype (white eyes), all the male offspring—who get their only X from their mother—have white eyes (\(\text{X}^w\text{Y}\)). All the female offspring have red eyes because they received two Xs, with the \(\text{X}^W\) from the father concealing the recessive \(\text{X}^w\) from the mother.
X-linked genetic disorders
The same principles we see at work in fruit flies can be applied to human genetics. In humans, the alleles for certain conditions (including some forms of color blindness, hemophilia, and muscular dystrophy) are X-linked. These diseases are much more common in men than they are in women due to their X-linked inheritance pattern.
Why is this the case? Let's explore this using an example in which a mother is heterozygous for a disease-causing allele. Women who are heterozygous for disease alleles are said to be carriers, and they usually don't display any symptoms themselves. Sons of these women have a 50% chance of getting the disorder, but daughters have little chance of getting the disorder (unless the father also has it), and will instead have a 50% chance of being carriers.
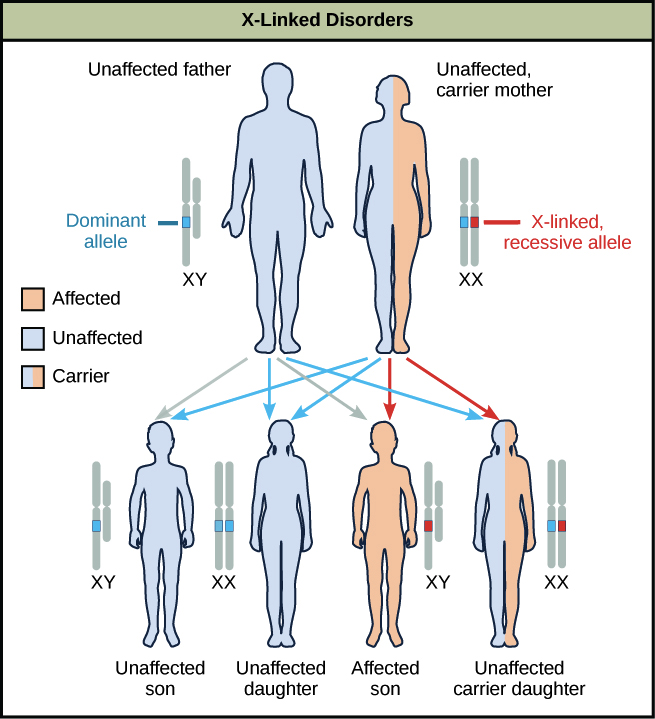
Why is this the case? Recessive X-linked traits appear more often in males than females because, if a male receives a "bad" allele from his mother, he has no chance of getting a "good" allele from his father (who provides a Y) to hide the bad one. Females, on the other hand, will often receive a normal allele from their fathers, preventing the disease allele from being expressed.
Case study: Hemophilia
Let's look at a Punnett square example using an X-linked human disorder: hemophilia, a recessive condition in which a person's blood does not clot properly13. A person with hemophilia may have severe, even life-threatening, bleeding from just a small cut.
Hemophilia is caused by a mutation in either of two genes, both of which are located on the X chromosome. Both genes encode proteins that help blood clot14. Let's focus on just one of these genes, calling the functional allele \(\text{X}^H\) and the disease allele \(\text{X}^h\).
In our example, a woman who is heterozygous for normal and hemophilia alleles (\(\text{X}^H\text{X}^h\)) has children with a man who is hemizygous for the normal form (\(\text{X}^H\text{Y}\)). Both parents have normal blood clotting, but the mother is a carrier. What is the chance of their sons and daughters having hemophilia?

Since the mother is a carrier, she will pass on the hemophilia allele (\(\text{X}^h\)) on to half of her children, both boys and girls.
- None of the daughters will have hemophilia (zero chance of the disorder). That's because, in order to have the disorder, they must get a \(\text{X}^h\) allele from both their mother and their father. There is 0 chance of the daughters getting an \(\text{X}^h\) allele from their father, so their overall chance of having hemophilia is zero.
- The sons get a Y from their father instead of an X, so their only copy of the blood clotting gene comes from their mother. The mother is heterozygous, so half of the sons, on average, will get an \(\text{X}^h\) allele and have hemophilia (1/2 chance of the disorder).
- [Can a woman ever have hemophilia?]
-
Yes, it's possible for a woman to have a recessive, X-linked condition such as hemophilia. However, she must get two recessive copies of the X-linked gene (one from each parent) in order to have the condition. The odds of this are much lower than the odds of a man getting just one recessive disease allele from his mother.
Check your understanding
Query \(\PageIndex{1}\)
- [Hint]
-
Because hemophilia is a recessive disorder, a woman must receive two disease alleles (one on each X chromosome) in order to display the disease. Thus, she must get a disease allele from both her mother and her father.
Since hemophilia is an X-linked disorder, males are hemizygous for the hemophilia-related gene (have only one allele and display the phenotype associated with that allele). In order to have a hemophilia allele that can be passed on to offspring, a male must himself be hemophiliac. Pairs of parents in which the male is not hemophiliac cannot produce a hemophiliac daughter, barring rare events such as spontaneous mutations in the the germline or during development of the embryo.
Women who are either homozygous for the hemophilia allele (hemophiliac) or heterozygous for the hemophilia allele (unaffected carriers) may pass on a hemophilia allele to their offspring. Pairs of parents in which the female is neither hemophiliac nor a carrier cannot produce a hemophiliac daughter (again, barring rare spontaneous mutation events).
Of the pairs above, a carrier mother and a hemophiliac father are the most likely to have a hemophiliac daughter.
Contributors and Attributions
Khan Academy (CC BY-NC-SA 3.0; All Khan Academy content is available for free at www.khanacademy.org)
- [Attribution and references]
-
Attribution:
This article is a modified derivative of "Characteristics and traits," by OpenStax College, Biology, CC BY 4.0. Download the original article for free at http://cnx.org/contents/185cbf87-c72e-48f5-b51e-f14f21b5eabd@10.53.
This article is licensed under a CC BY-NC-SA 4.0 license.
Works cited:
-
X chromosome. (2015, December 11). Retrieved December 11, 2015 from Wikipedia: https://en.wikipedia.org/wiki/X_chromosome.
-
Reece, J. B., Urry, L. A., Cain, M. L., Wasserman, S. A., Minorsky, P. V., and Jackson, R. B. (2011). The chromosomal basis of sex. In Campbell biology (10th ed., pp. 296-297). San Francisco, CA: Pearson.
-
X chromosome. (2012). In Genetics home reference. Retrieved from http://ghr.nlm.nih.gov/chromosome/X.
-
Y chromosome. (2010). In Genetics home reference. Retrieved from http://ghr.nlm.nih.gov/chromosome/Y.
-
Meiosis. (2015). In HHMI biointeractive. Retrieved from http://www.hhmi.org/biointeractive/meiosis.
-
SRY. (2015). In Genetics home reference. Retrieved from http://ghr.nlm.nih.gov/gene/SRY.
-
46,XX testicular disorder of sex development. (2008). In Genetics home reference. Retrieved from https://ghr.nlm.nih.gov/condition/46xx-testicular-disorder-of-sex-development.
-
Swyer syndrome. (2015). In Genetics home reference. Retrieved from https://ghr.nlm.nih.gov/condition/swyer-syndrome.
-
King, V., Goodfellow, P. N., Pearks Wilkerson, A. J., Johnson, W. E., O'Brien, S. J., and Pecon-Slattery, J. Evolution of the male-determining gene SRY within the cat family Felidae. Genetics, 175(4), 1855-1867. http://dx.doi.org/10.1534/genetics.106.066779.
-
Dimorphism. (2015). In Fine dictionary. Retrieved from http://www.finedictionary.com/dimorphism.html.
-
Bachtrog, Doris. (2013). Y chromosome evolution: Emerging insights into processes of Y chromosome degeneration. Nature Reviews Genetics, 14(2), 113-124. http://dx.doi.org/10.1038/nrg3366. Retrieved from http://www.ncbi.nlm.nih.gov/pmc/articles/PMC4120474/.
-
Gilbert, S. F. (2000). Chromosomal sex determination in Drosophila. In _Developmental Biology (6th ed.). Sunderland, MA: Sinauer Associates. Retrieved from www.ncbi.nlm.nih.gov/books/NBK10025/.
-
Hemophilia. (2015). In Genetics home reference. Retrieved from https://ghr.nlm.nih.gov/condition/hemophilia.
-
Kimball, J. W. (2015, December 23). Sex chromosomes. In Kimball's biology pages. Retrieved July 27, 2016 from http://www.biology-pages.info/S/SexChromosomes.html.
Additional references:
Bachtrog, Doris. (2013). Y chromosome evolution: Emerging insights into processes of Y chromosome degeneration. Nature Reviews Genetics, 14(2), 113-124. http://dx.doi.org/10.1038/nrg3366. Retrieved from http://www.ncbi.nlm.nih.gov/pmc/articles/PMC4120474/.
Bergmann, D. C. (2011). Genetics lecture notes. Biosci41, Stanford University.
Bourke, A. F. G. and Franks, N. R. (1995). Relatedness and reproductive value in the social Hymenoptera. In Social evolution in ants (p. 78). Princeton, NJ: Princeton University Press.
Bowen, R. A. (2000, August 17). Preparing a karyotype. In General and medical genetics. Retrieved from http://www.vivo.colostate.edu/hbooks/genetics/medgen/chromo/cytotech.html.
Carvalho, A. B., Koerich, L. B., and Clark, A. G. (2009). Origin and evolution of Y chromosomes: Drosophila tales. Trends in Genetics, 25(6), 270-277. http://dx.doi.org/10.1016/j.tig.2009.04.002. Retrieved from http://www.ncbi.nlm.nih.gov/pmc/articles/PMC2921885/.
Deeb, S. S. and Motulsky, A. G. (2015, February 5). Red-green color vision defects. In GeneReviews. Retrieved from http://www.ncbi.nlm.nih.gov/books/NBK1301/.
Genetic factors and hormones that determine gender. (2007, June 27). In Human embryology: Organogenesis. Retrieved from http://www.embryology.ch/anglais/ugenital/molec02.html.
Haplodiploidy. (2015, November 7). Retrieved December 11, 2015 from Wikipedia: https://en.wikipedia.org/wiki/Haplodiploidy.
Kimball, J. W. (2015, December 23). Sex chromosomes. In Kimball's biology pages. Retrieved July 27, 2016 from http://www.biology-pages.info/S/SexChromosomes.html.
Krempels, D. M. (n.d.). The genetics of calico cats. Retrieved from www.bio.miami.edu/dana/dox/calico.html.
OpenStax College, Biology. (2015, May 13). Chromosomal basis of inherited disorders. In OpenStax CNX. Retrieved from http://cnx.org/contents/185cbf87-c72e-48f5-b51e-f14f21b5eabd@9.85:65/Chromosomal-Basis-of-Inherited.
OpenStax College, Biology. (2015, May 13). Chromosomal theory and genetic linkage. In OpenStax CNX. Retrieved from http://cnx.org/contents/185cbf87-c72e-48f5-b51e-f14f21b5eabd@9.85:64/Chromosomal-Theory-and-Genetic
OPN1LW. (2015). In Genetics home reference. Retrieved from http://ghr.nlm.nih.gov/gene/OPN1LW.
Pseudoautosomal region. (2015, September 2). Retrieved December 16, 2015 from Wikipedia: https://en.wikipedia.org/wiki/Pseudoautosomal_region.
Purves, W. K., Sadava, D. E., Orians, G. H., and Heller, H.C. (2003). Sex determination and sex-linked inheritance. In Life: The science of biology (7th ed., pp. 125-144). Sunderland, MA: Sinauer Associates.
Reece, J. B., Urry, L. A., Cain, M. L., Wasserman, S. A., Minorsky, P. V., and Jackson, R. B. (2011). Sex-linked genes exhibit unique patterns of inheritance. In Campbell biology (10th ed., pp. 205-209). San Francisco, CA: Pearson.
Testis determining factor. (2015, November 10). Retrieved December 11, 2015 from Wikipedia: https://en.wikipedia.org/wiki/Testis_determining_factor.
X0 sex-determination system (2015, July 20). Retrieved December 11, 2015 from Wikipedia: https://en.wikipedia.org/wiki/X0_sex-determination_system.
XY sex-determination system. (2015, November 19). Retrieved December 11, 2015 from Wikipedia: https://en.wikipedia.org/wiki/XY_sex-determination_system.
ZW sex-determination system. (2015, November 16). Retrieved December 11, 2015 from Wikipedia: https://en.wikipedia.org/wiki/ZW_sex-determination_system.
Zygosity. (2015, November 29). Retrieved December 11, 2015 from Wikipedia: https://en.wikipedia.org/wiki/Zygosity.
-