2: Fertilization and Cortical Rotation
( \newcommand{\kernel}{\mathrm{null}\,}\)
Fertilization
For sexually reproducing species, the completion of fertilization marks the start of embryogenesis. You are already aware that species have different reproductive isolation mechanisms that prevent interspecific fertilization. In the case of sea urchins (links below) you will see that there are several steps that can mediate species-specific recognition. These include chemo-attractants (chemicals secreted by the egg that the sperm responds to) and sticky molecules held by the sperm (bindins) that only interact with the same species eggs.
The sperm-entry point is also significant in many species in that it marks the first break in egg symmetry. Imagine a fairly symmetric ball. Now draw a single dot on that ball, this dot is now a landmark. You can be opposite the dot, close to the dot, or right on the dot. The sperm-entry point, like the dot, acts as a landmark to break symmetry. It does so in at least two related ways, by donating a centrosome to the egg, and by triggering cytoskeletal rearrangements. The sperm centrosome, a tiny organelle that acts as a seed for microtubule formation, can act as an organizer of the spindle apparatus. By putting one side of the spindle near the sperm-entry point, it can define the axis of the first cleavage (Figure 1).
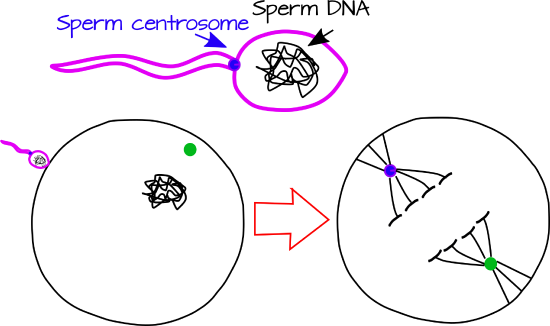
Additionally, the sperm-entry point can be a trigger for cytoskeletal shifting, including a process called cortical rotation, wherein the outer layer of the cell (the cortex) rotates relative to the inner cytoplasm. While cortical rotation is limited to a few species (that we know about), studying it has led to breakthroughs in EvoDevo and developmental biology in general.
Cortical rotation's significance as a symmetry breaking event is underlined when we see what happens if we prevent it. Brief treatments of frog (Xenopus laevis) fertilized eggs with agents that affect microtubule polymerization prevent cortical rotation and result in a "ventralized" embryo - that is, it has ventral structures but lacks dorsal structures including those that would build a spinal cord. We call an embryo like this a "belly piece." What is it about cortical rotation that turns a frog into a frog, rather than into a mass of belly tissue?
Cortical rotation as an EvoDevo example
Even though cortical rotation is a process found in only a few animal species, it is an example of several fundamental concepts in EvoDevo
1) Establishment of organizers. Organizers are parts of the embryo that produce signals that pattern nearby or distant tissues. In Drosophila, Bicoid mRNA marks the head organizer, for example. Depletion of Bicoid leads to headless flies, too much Bicoid leads to big-headed larvae. Later in the semester we will examine the ZPA organizer in vertebrate limbs.
Frogs have a Spemann-Mangold organizer (named after student Hilde Mangold and her advisor Hans Spemann who discovered and characterized it)2. Removal of the organizer tissue leads to a ball of belly tissue. Addition of an organizer to another location on the embryo leads to twinned tadpoles.
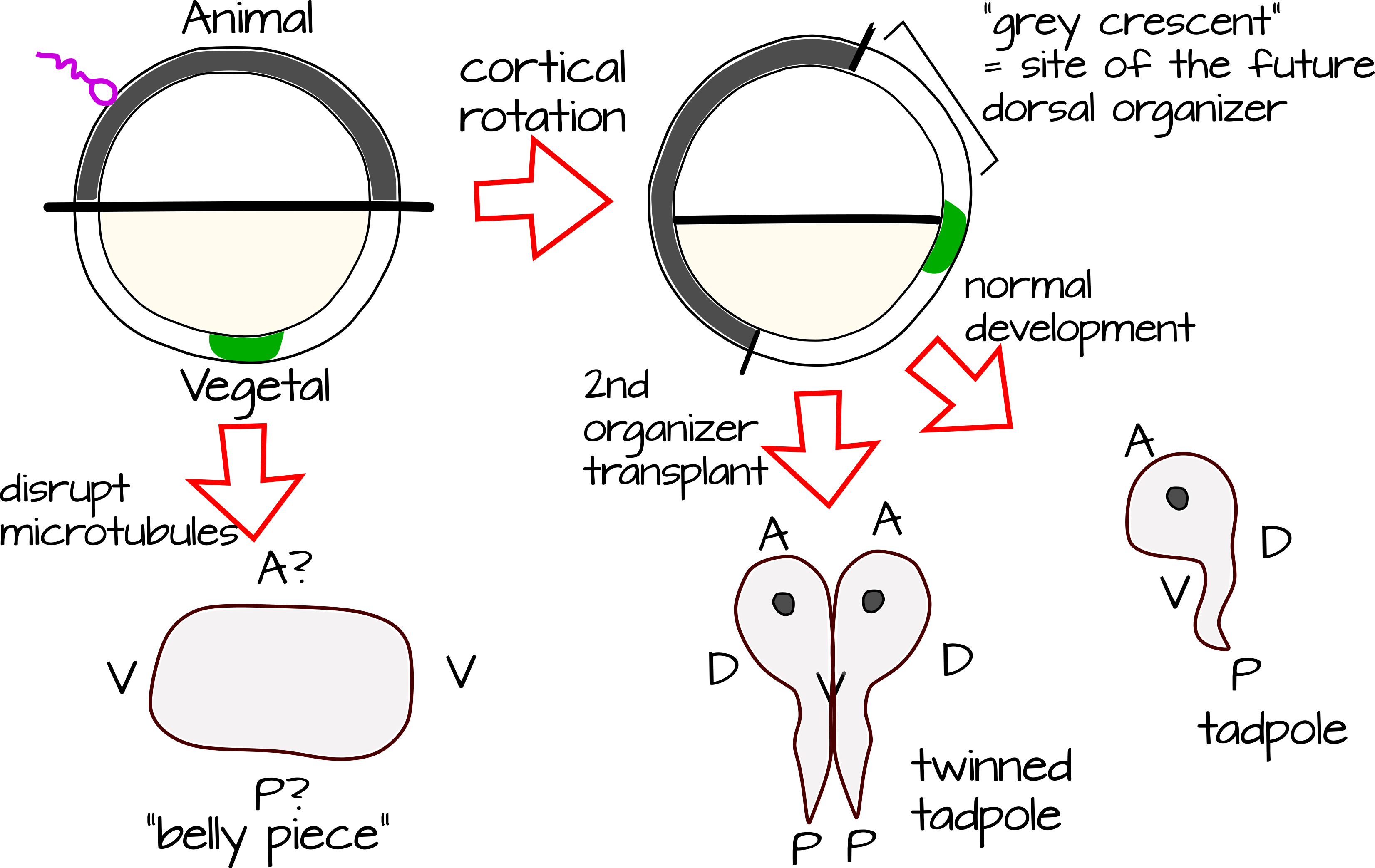
2) Induction of cellular differentiation. Many cells have a "default" path that they will follow over time if they are not given additional instructions. One of the main roles of an organizer is to make and send off those additional instructions. Organizers do this through cell-signaling molecules and gene regulation. The Bicoid example in Drosophila is an example of gene regulation. The frog example demonstrates both gene regulation and cell-signaling, as I will soon describe.
3) Development happens in 4D. Not only do we have to think about complex 3D objects when we are thinking about development, but we also need to consider time. Over the course of time, cells and parts of cells change their positions. This changes the "local environment" - changing the types of interactions that can happen. This will be more important in gastrulation, but we will see the start of it here in cortical rotation. In the case of cortical rotation, the rotation changes the locations of mRNAs and proteins. These will eventually be inherited by different cells and will give these cells starting instructions for differentiation. These cells will later change their position and, as they come into contact with new neighbors, will differentiate further and give out new instructions.
Sperm entry triggers changes in the egg cell
An unfertilized frog oocyte is not perfectly symmetrical. It has radial symmetry, that is you can stick a pin from top to bottom and it will be symmetric around the pin. However, the top and bottom sides differ. The bottom (vegetal pole) is enriched with dense yolk granules while the top (animal pole) is enriched with less dense cytoplasm and pigment. Similar animal/vegetal symmetry is seen in many species. Frog eggs are also asymmetric in that they are made of two layers. An outer cortex and an inner cell mass. The animal and vegetal poles differ in the mRNAs and proteins (see Briefly, Genetics for a refresher on molecular biology terms)3 and so do the inner and outer parts of the oocyte.
Sperm entry induces a change in the way cytoskeletal microtubules interact with each other. They go from being a loosely organized network across the cell to a parallel array with highest density in the cortex and at the cortex/inner cell mass border. These microtubules rotate the cortex relative to the inner cell mass, taking with them the asymetrically localized cortical determinants2. Because the pigmentation in the egg was unevently distributed, this creates a "grey crescent" where the vegetal cortex now lies over the animal inner cell mass (Figure 2)
The interaction between vegetal cortex molecular determinants and animal inner-cell mass molecular determinants makes this grey crescent unique compared to either the animal or vegetal pole of the fertilized egg. The grey crescent marks the future dorsal side of the frog. You can see now why elimination of cortical rotation or elimination of the molecular determinants that populate the grey crescent lead to ventralization of the frog. Duplication of the grey crescent, either through transplantation or genetic manipulation, leads to an extra dorsal axis and "twinning" of the frog.
In the next chapter, Cleavage and Gastrulation, we will examine the development and genetic of this organizer and think about the consequences of organizing tissues in evolution.
References
- "Mitosis in the human embryo: the vital role of the sperm centrosome (centriole)", 1997, Histology and Histopathology, A. Henry Sathananthan PMID:
9225167 - “Induction of Embryonic Primordia by Implantation of Organizers from a Different Species,” 1924, Archiv für Mikroskopische Anatomie und Entwicklungsmechanik, Hans Spemann and Hilde Mangold., PMID: 11291841
- Reviewed in: "Cortical rotation and messenger RNA localization in Xenopus axis formation", 2012, WIREs Developmental Biology, Douglas W. Houston doi: 10.1002/wdev.29
Further Reading: Developmental Biology
www.ncbi.nlm.nih.gov/books/NBK9983/
- Introduction to fertilization
- 1. Sperm and Egg
- 2. How do you do? Sperm and egg recognition
- 3. One sperm per egg: the cortical granule reaction
- 4. OPTIONAL: The activation of egg metabolism
- 5. OPTIONAL: Two become one, fusion of genetic material
- 6. Breaking symmetry: Rearrangement of the egg cytoplasm
- 7. Summary