1.21: Metabolic diversity
- Page ID
- 70340
\( \newcommand{\vecs}[1]{\overset { \scriptstyle \rightharpoonup} {\mathbf{#1}} } \)
\( \newcommand{\vecd}[1]{\overset{-\!-\!\rightharpoonup}{\vphantom{a}\smash {#1}}} \)
\( \newcommand{\id}{\mathrm{id}}\) \( \newcommand{\Span}{\mathrm{span}}\)
( \newcommand{\kernel}{\mathrm{null}\,}\) \( \newcommand{\range}{\mathrm{range}\,}\)
\( \newcommand{\RealPart}{\mathrm{Re}}\) \( \newcommand{\ImaginaryPart}{\mathrm{Im}}\)
\( \newcommand{\Argument}{\mathrm{Arg}}\) \( \newcommand{\norm}[1]{\| #1 \|}\)
\( \newcommand{\inner}[2]{\langle #1, #2 \rangle}\)
\( \newcommand{\Span}{\mathrm{span}}\)
\( \newcommand{\id}{\mathrm{id}}\)
\( \newcommand{\Span}{\mathrm{span}}\)
\( \newcommand{\kernel}{\mathrm{null}\,}\)
\( \newcommand{\range}{\mathrm{range}\,}\)
\( \newcommand{\RealPart}{\mathrm{Re}}\)
\( \newcommand{\ImaginaryPart}{\mathrm{Im}}\)
\( \newcommand{\Argument}{\mathrm{Arg}}\)
\( \newcommand{\norm}[1]{\| #1 \|}\)
\( \newcommand{\inner}[2]{\langle #1, #2 \rangle}\)
\( \newcommand{\Span}{\mathrm{span}}\) \( \newcommand{\AA}{\unicode[.8,0]{x212B}}\)
\( \newcommand{\vectorA}[1]{\vec{#1}} % arrow\)
\( \newcommand{\vectorAt}[1]{\vec{\text{#1}}} % arrow\)
\( \newcommand{\vectorB}[1]{\overset { \scriptstyle \rightharpoonup} {\mathbf{#1}} } \)
\( \newcommand{\vectorC}[1]{\textbf{#1}} \)
\( \newcommand{\vectorD}[1]{\overrightarrow{#1}} \)
\( \newcommand{\vectorDt}[1]{\overrightarrow{\text{#1}}} \)
\( \newcommand{\vectE}[1]{\overset{-\!-\!\rightharpoonup}{\vphantom{a}\smash{\mathbf {#1}}}} \)
\( \newcommand{\vecs}[1]{\overset { \scriptstyle \rightharpoonup} {\mathbf{#1}} } \)
\( \newcommand{\vecd}[1]{\overset{-\!-\!\rightharpoonup}{\vphantom{a}\smash {#1}}} \)
\(\newcommand{\avec}{\mathbf a}\) \(\newcommand{\bvec}{\mathbf b}\) \(\newcommand{\cvec}{\mathbf c}\) \(\newcommand{\dvec}{\mathbf d}\) \(\newcommand{\dtil}{\widetilde{\mathbf d}}\) \(\newcommand{\evec}{\mathbf e}\) \(\newcommand{\fvec}{\mathbf f}\) \(\newcommand{\nvec}{\mathbf n}\) \(\newcommand{\pvec}{\mathbf p}\) \(\newcommand{\qvec}{\mathbf q}\) \(\newcommand{\svec}{\mathbf s}\) \(\newcommand{\tvec}{\mathbf t}\) \(\newcommand{\uvec}{\mathbf u}\) \(\newcommand{\vvec}{\mathbf v}\) \(\newcommand{\wvec}{\mathbf w}\) \(\newcommand{\xvec}{\mathbf x}\) \(\newcommand{\yvec}{\mathbf y}\) \(\newcommand{\zvec}{\mathbf z}\) \(\newcommand{\rvec}{\mathbf r}\) \(\newcommand{\mvec}{\mathbf m}\) \(\newcommand{\zerovec}{\mathbf 0}\) \(\newcommand{\onevec}{\mathbf 1}\) \(\newcommand{\real}{\mathbb R}\) \(\newcommand{\twovec}[2]{\left[\begin{array}{r}#1 \\ #2 \end{array}\right]}\) \(\newcommand{\ctwovec}[2]{\left[\begin{array}{c}#1 \\ #2 \end{array}\right]}\) \(\newcommand{\threevec}[3]{\left[\begin{array}{r}#1 \\ #2 \\ #3 \end{array}\right]}\) \(\newcommand{\cthreevec}[3]{\left[\begin{array}{c}#1 \\ #2 \\ #3 \end{array}\right]}\) \(\newcommand{\fourvec}[4]{\left[\begin{array}{r}#1 \\ #2 \\ #3 \\ #4 \end{array}\right]}\) \(\newcommand{\cfourvec}[4]{\left[\begin{array}{c}#1 \\ #2 \\ #3 \\ #4 \end{array}\right]}\) \(\newcommand{\fivevec}[5]{\left[\begin{array}{r}#1 \\ #2 \\ #3 \\ #4 \\ #5 \\ \end{array}\right]}\) \(\newcommand{\cfivevec}[5]{\left[\begin{array}{c}#1 \\ #2 \\ #3 \\ #4 \\ #5 \\ \end{array}\right]}\) \(\newcommand{\mattwo}[4]{\left[\begin{array}{rr}#1 \amp #2 \\ #3 \amp #4 \\ \end{array}\right]}\) \(\newcommand{\laspan}[1]{\text{Span}\{#1\}}\) \(\newcommand{\bcal}{\cal B}\) \(\newcommand{\ccal}{\cal C}\) \(\newcommand{\scal}{\cal S}\) \(\newcommand{\wcal}{\cal W}\) \(\newcommand{\ecal}{\cal E}\) \(\newcommand{\coords}[2]{\left\{#1\right\}_{#2}}\) \(\newcommand{\gray}[1]{\color{gray}{#1}}\) \(\newcommand{\lgray}[1]{\color{lightgray}{#1}}\) \(\newcommand{\rank}{\operatorname{rank}}\) \(\newcommand{\row}{\text{Row}}\) \(\newcommand{\col}{\text{Col}}\) \(\renewcommand{\row}{\text{Row}}\) \(\newcommand{\nul}{\text{Nul}}\) \(\newcommand{\var}{\text{Var}}\) \(\newcommand{\corr}{\text{corr}}\) \(\newcommand{\len}[1]{\left|#1\right|}\) \(\newcommand{\bbar}{\overline{\bvec}}\) \(\newcommand{\bhat}{\widehat{\bvec}}\) \(\newcommand{\bperp}{\bvec^\perp}\) \(\newcommand{\xhat}{\widehat{\xvec}}\) \(\newcommand{\vhat}{\widehat{\vvec}}\) \(\newcommand{\uhat}{\widehat{\uvec}}\) \(\newcommand{\what}{\widehat{\wvec}}\) \(\newcommand{\Sighat}{\widehat{\Sigma}}\) \(\newcommand{\lt}{<}\) \(\newcommand{\gt}{>}\) \(\newcommand{\amp}{&}\) \(\definecolor{fillinmathshade}{gray}{0.9}\)Lessons from eccentrics
Sometimes understanding a process is aided by looking at variations on the process. We are studying how organisms satisfy their energetic and material needs. The primary material we are considering right now is carbon, but it generally brings with it hydrogen and oxygen as a carbohydrate molecule. We will get to other materials in a subsequent chapter, but carbon, hydrogen and oxygen make up 95% of most organisms. We will first review the common patterns found in most organisms. We will then consider several groups of organisms whose energy and carbon processing is eccentric in various ways.
TOPICS
- Review: 'normal' patterns involving matter and energy
- Acquiring energy—cellular respiration
- Acquiring food—heterotrophs and photosynthetic autotrophs
- Acquiring carbon—heterotrophs and photosynthetic autotrophs
- 'Eccentric' organisms
- Obtaining energy when there is no oxygen
- fermentors
- using alternative electron acceptors
- Chemoautotrophs, making food (carbohydrates) without light
- Photoautotrophs, separating matter and energy
- Obtaining energy when there is no oxygen
'The norm' — the most common processes
1. Acquiring metabolic energy
Nearly all organisms utilize the reactions discussed in the chapter on cellular respiration to supply their energetic needs: cellular respiration provides energy in the form of ATP as long as a supply of carbohydrates and oxygen is available. Oxygen is readily available in all terrestrial habitats due to the fact that the atmosphere is 20% oxygen and local depletions are rare because the atmosphere is continually mixed by winds. In contrast, in some aquatic situations (including soils saturated with water), oxygen may be in short supply. Present in such situations are a number of organisms, both prokaryotic (bacteria or archaea) and eukaryotic, that can obtain ATP energy in the absence of oxygen, in a manner that utilizes only the glycolysis part of cellular respiration. Alternatively, there also are organisms, solely prokaryotes, that have mechanisms to make ATP that don't rely at all on the pathways of cellular respiration.
2. Making your own food: energy flow in photosynthetic autotrophs
If you consider it closely, the energetics of photosynthetic organisms seems unnecessarily involved: the light reactions produce ATP and NADPH in order to synthesize carbohydrates from carbon dioxide. Then the carbohydrates are broken down in cellular respiration to produce NADH (for our purposes the same as NADPH) and ATP. Why bother making carbohydrates; why not just use the light reactions to obtain 'energy currency, ' ATP and NADH? T o a limited extent, photosynthetic organisms DO satisfy some of their energetic needs directly off of the light reactions, i.e., not all of the ATP and NADPH produced is used to make carbohydrates, some power other processes. But for the most part, the flow of energy in photosynthetic organisms goes from light to ATP and NADPH, to carbohydrates and then back to ATP and NADH; and the amount of energy available is diminished along the way because the energy transfers are not 100% efficient. There are several justifications to this behavior of photosynthetic organisms:
- cellular respiration evolved first and photosynthesis appeared as a means to allow organisms to avoid having to 'beg and steal for food'
- the need for energetic compounds is continuous but nighttime prevents photosynthesis from happening., ATP and NADH cannot be readily be stored and, while they are constantly recycled, the absolute amounts of ADP/ATP and NADP/NADPH are small. In contrast, carbohydrates can stored and can be present in much greater amounts.
- for vascular plants, the below-ground parts need energetic compounds yet can't photosynthesize. The labile nature of ATP and NADH means that they cannot be transported, while carbohydrates can.
But we will see that there are some organisms who use light in a strictly energetic role, not using it to make carbohydrates that are subsequently be used to obtain energy.
3. Material needs, specifically carbon
As to material needs, for the most part (or you might say 'for most of their parts' !), organisms are composedof carbohydrates, or molecules that are made from carbohydrates, e.g., the amino acids of proteins, which basically consist of a carbohydrate with nitrogen group(s) and occasionally also a sulfur group attached, or fats, which are made from carbohydrates that have had most of their oxygens removed. Thus anyorganism must obtain carbohydrates to construct more of themselves, i.e., to grow. For photosynthetic autotrophs, carbohydrate needs are supplied by photosynthesis but note that whatever carbohydrates directed towards material needs are not available to be used for energetic needs. For heterotrophs, carbohydrate needs are satisfied by appropriating part of what is consumed to whatever 'building projects' a heterotroph may require, but, as a result, reducing the amount of energy that can be obtained. Nearly all organisms are either heterotrophs, whose consumption of organic material (bodies or parts of bodies of organisms) provides them with both energy and with carbon materials or photosynthetic autotrophs who use light to make their own carbohydrates out of carbon dioxide and water and then, like heterotrophs, use this 'food' for both material and energetic needs. However, there are some organisms (all prokaryotic) who are non-photosynthetic autotrophs, i.e., they are able to make carbohydrates without sunlight, using chemicals as an energy supply. There also are organisms, again prokaryotes, whose dietary habits include or require some materials that are not obviously 'organic' , i.e., made by organisms, e.g., formaldehydeor 'plastic' (polyethylene terephthalate). There also are some organisms that 'eat' solely for carbon nutrition and have other mechanisms, that are not based on carbohydrate food, to obtain energy.
Below are some groups of eccentric organisms, organisms that differ from the more usual patterns discussed above.
Eccentric organisms I: anaerobic organisms—use of alternate electron acceptors
Remember that oxygen 's role in cellular respiration is to be an ' electron magnet ', a low point to which electrons flow. Electrons obtained from carbohydrates are transferred to form NAD+ (forming NADH) and from it electrons flow through a series of carriers of the electron transport chain. Oxygen' s role is essential both because it 'drives' the electron flow that in turn allows ATP to be synthesized, and also because the process regenerates NAD + which is required for both glycolysis and the citric acid cycle to continue. I n most of the earth 's habitats, oxygen is plentiful and has been ever since photosynthesis became popular roughly 2 billion years ago. But there are situations where oxygen becomes scarce, generally as the result of cellular respiration coupled with physical factors, e.g., waterlogged soils, that make oxygen replenishment unlikely (Chapter 26). One solution to an oxygen deficit is to find another atom or molecule that will serve the same role, i.e., be a ' downhill situation' to which electrons can flow.
There are a number of bacteria that do this, utilizing a number of different molecules as substitutes for oxygen. A particularly important group to plant nutrition is called denitrifying bacteria. They use nitrate (NO3–) as the electron acceptor that receives the electrons from NADH produced in glycolysis and the citric acid cycle. In the process, they convert nitrate, a form of nitrogen that most plants can assimilate (i.e., utilize) into dinitrogen gas (N2), a form of nitrogen that plants cannotassimilate (although some can with the aid of a symbiont). Moreover, N2is volatile and can escape the soil; in contrast, nitrate is an ion and consequently unable to leave the soil solution, although the soil solution itself may leave the soil, taking ions with it (i.e., leaching). The process of converting nitrate to dinitrogen gas is called denitrification and in some situations, it causes a substantial loss of nitrogen from soils. Another substitute for oxygen is sulfate (SO42-), which, after accepting electrons, is converted to hydrogen sulfide. As was the case with denitrification, these reactions adversely affect plant mineral nutrition by eliminating a form that the plant can assimilate (sulfate) and putting sulfur into a form that plants are less able to acquire and also a form that is volatile and can be lost from the plant's habitat.
Eccentric organisms II: anaerobic organisms—fermentation
One solution to a scarcity of oxygen, and one that is the closest to the normal patterns, is a process called fermentation ( 'anaerobic respiration' ) which involves an addendum to glycolysis, the first stage of cellular respiration, and necessarily the elimination of the remaining parts of cellular respiration. The pyruvate produced by glycolysis does not go through the citric acid cycle but instead is used directly or indirectly to accept electrons from NADH, allowing NAD+ (Figure 1), which is essential to glycolysis, to be regenerated. Significant to the fermenting organism is that this allows glycolysis to proceed, although much less ATP is obtained in the process than would be formed if the citric acid cycle and oxidative phosphorylation were able to occur. Also significant is that, while the end products of cellular respiration are water and carbon dioxide, benign substances that are easily dispersed, the end products of fermentation, commonly ethanol or lactic acid, are more toxic and more difficult to eliminate. Fermentation is extremely important in human affairs, both in the production of alcoholic beverages (generally by fungi but occasionally by bacteria) and in the production of desirable food products, e.g., sourdough (Figure 2), sauerkraut, sour cream, yogurt, all of which are influenced by lactic acid production. Some fermenting organisms are facultative anaerobes (i.e., they can live with or without oxygen). Most of these switch to fermentation if oxygen is not present but some carry out fermentation regardless of oxygen availability. Some are obligate anaerobes, meaning that they cannot live in the presence of oxygen.
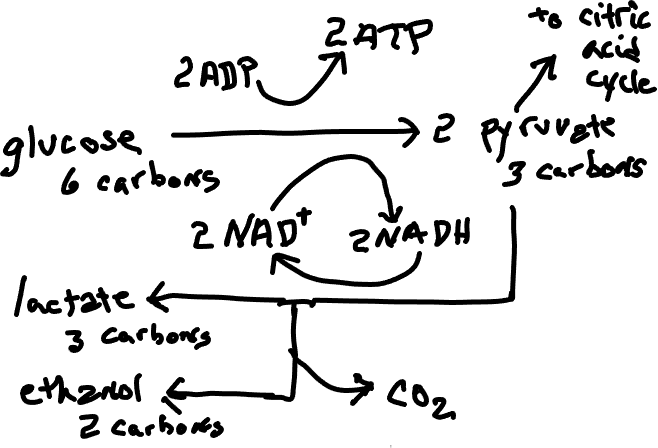
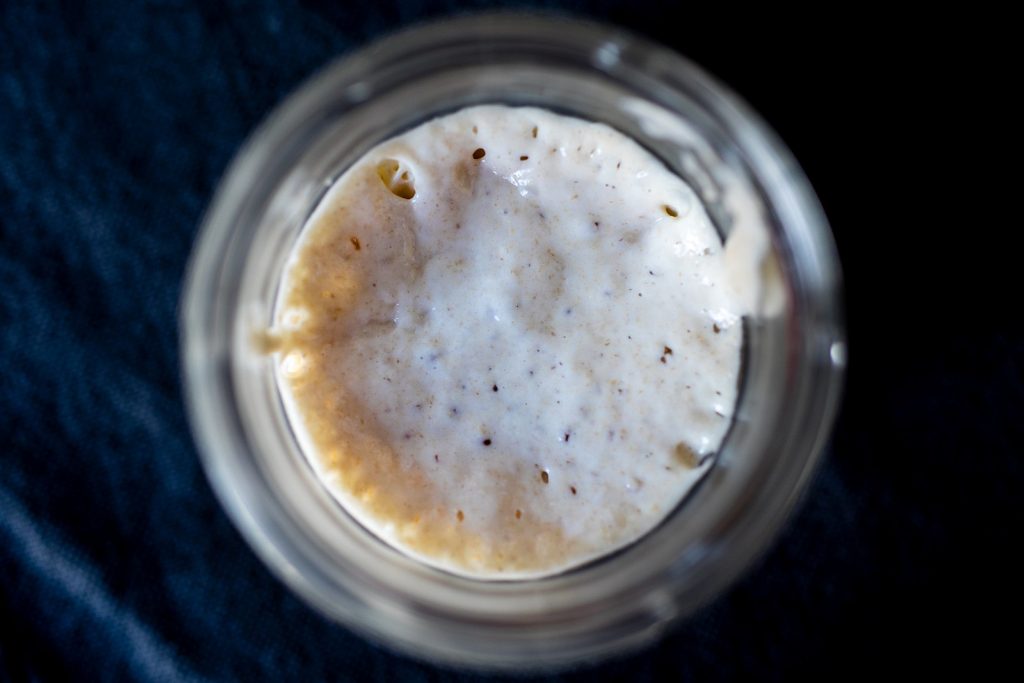
Eccentric organisms III: making food without sunlight- chemosynthetic organisms
Most autotrophs ( 'self-eaters' ) make food through photosynthesis and then eat themselves. The energy of light is what makes an unfavorable reaction, the reduction of carbon dioxide by water, more likely. A vague but common description of the process is that some of the energy of light is captured by the plant and stored as carbohydrates. A more specific description is that light is able, in the organized structure of a membrane, to move electrons in a way that NADPH is formed from NADP+using electrons derived from water. In the process, ATP is formed as a result of the electron flow. In the Calvin cycle, these products, NADPH and ATP, can cause carbon to be reduced with electrons provided by NADPH and with ATP promoting the reactions. Light is essential to photosynthesis because it provides a mechanism to obtain NADPH and ATP. But these metabolites can be made in other ways and there are non-photosynthetic autotrophs that do exactly that. They are described as chemosynthetic organisms and they make their own food in ways that do not require light. What is needed is a chemical that can donate electrons to reduce NADP+to NADPH and a membrane system that allows ATP to be synthesized as the electrons flow from the donor to NADP+. Chemosynthetic organisms are uncommon and are only found within bacteria and archaea, but they can be very significant in certain habitats and in carrying out processes that are important to global biogeochemical cycles. From a plant perspective, the most important group of these organisms are the nitrifying bacteria, a group that oxidizesammonia (NH3) to nitrite (NO2–), and a group that oxidizes nitrite to nitrate (NO3–). The result ofthe two reactionsis that ammonia is converted to nitrate, which is the preferred nitrogen source for most plants, and, unlike ammonia, cannot escape the soil as a gas. Note that both these reactions are oxidations(this is obvious in the NH3to NO2–reaction as hydrogens are removed from nitrogen, but is also true in the NO2–to NO3–conversion). The electrons removed from the nitrogen compound are used to reduce NADP+to NADPH and provide for an electron flow (through a membrane) that allows ATP to be synthesized. These reagents are then used to reduce carbon dioxide in the reactions of the Calvin cycle, 'fixing' it into a carbohydrate form. The nitrifying bacteria, like photosynthetic plants, make their own food and then use it for synthetic reactions (as a building material) or as a source of energy (as it is oxidized to carbon dioxide in cellular respiration). There are other chemosynthetic organisms besides the nitrifying bacteria, including bacteria in deep-sea vents, that utilize hydrogen sulfide as an energy source to fix carbon, methanogens, that use energy from dihydrogen gas (H2) to fix carbon (and at the same time producing methane), and methane 'eaters' that oxidize methane to dinitrogen (N2) while reducing carbon. Many, but not all, chemosynthetic organisms are archaebacteria, although nitrifying bacteria are not.
Eccentric organisms IV: separating matter and energy
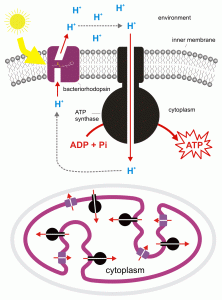
For most organisms 'food' , i.e., what heterotrophs absorb or ingest and for photosynthesizers, the carbohydrates that they make, plays a dual role: as an energy source (producing ATP and NADH) and as a carbon source, providing 'reduced carbons' that are used in a variety of biosynthetic reactions that ultimately can make the organism bigger. The pathways for the two processes are initially the same but if the carbon is to be utilized as building material much less energy is obtained and less carbon dioxide is produced. If used for energy, 'food' i.e., carbohydrates, ends up as carbon dioxide and the amount of energy obtained is maximal; if used for material, the carbons of the carbohydrate end up in any one of the thousands of biomolecules found in the organism, in fats, proteins, nucleic acids, etc., and the amount of energy obtained is reduced compared to what would happen if all the carbons were totally oxidized to CO2. However, there are some organisms who have distinct pathways for obtaining energy, pathways that generally don 't involve carbon at all and their' eating 'is solely to obtain carbon atoms for biosynthesis. The easiest group to understand is photoheterotrophs. They include both archaea and bacteria that are capable of using sunlight in a process that allows them to synthesize ATPbut not in a manner that produces carbohydrates.Thus they must' eat food'(absorb carbohydrates/organic molecules) NOT necessarily for their energetic needs (i.e., to supply ATP) but rather to satisfytheir carbonneeds. This lifestyle is found in a few Archaea, for example, Halobacteriumwhich possesses a pigment, bacteriorhodopsin, related to the rhodopsinfound in vertebrate eyes. Bacteriorhodopsin is a membrane-spanning protein that can acquire protons in the cytosol, change conformation due to the absorption of light, and release protons on the outside of the membrane (Figure 3). Protons then flow into the cell, down their electrochemical gradient and, as is the case in the light reactions of photosynthesis and oxidative phosphorylation in cellular respiration, the proton movement causes ATP to be synthesized from ADP and inorganic phosphate. Most of the photoheterotrophs are bacteria that use a form of chlorophyll to absorb light energy and again create a proton gradient that can be used to synthesize ATP but need a source of reduced carbon for material needs.
In a similar manner there are chemoheterotrophs, organisms that 'eat' for their material (i.e., reduced carbon needs) but generate ATP by chemical means that doesNOT involve the cellular respirationand oxidation of carbohydrates.
Table 1 below summarizes the different modes of acquiring matter (carbon) and energy (reducing power and ATP).
Table 1.
energy |
carbon source |
groups |
|
heterotroph |
energy (reducing power and ATP) from oxidation of collected biomolecules |
carbon (reduced) from collected biomolecules |
all animals, all fungi, slime molds, water molds, some dinoflagellates, most bacteria, most archea |
photosynthetic autotroph |
energy (reducing power and ATP) from oxidation of self-made biomolecules |
carbon from carbon dioxide reduced in photosynthesis and used to synthesize biomolecules |
all plants, green algae, red algae, brown algae, diatoms, some dinoflagellates, cryptomonads, cyanobacteria, green sulfur bacteria |
chemosynthetic autotroph |
energy (reducing power and ATP) from oxidation of self-made biomolecules |
carbon from carbon dioxide reduced in photosynthesis used to synthesize biomolecules |
some, but not many, bacteria (nitrifying bacteria, sulfur-oxidizing bacteria, iron-oxidizing bacteria, some methanogens) and some archaea (methanogens). |
photoheterotroph |
energy (some reducing power but mostly ATP) from light-driven reactions |
carbon (reduced) from collected biomolecules |
Some, but not many, archaea (Halobacterium), some green non-sulfur bacteria, some purple non-sulfur bacteria. |
chemosynthetic heterotroph |
energy (reducing power and ATP) from electron flow driven by inorganic sources of electrons |
carbon (reduced) from collected biomolecules |
some, but not many, bacteria (Beggiatoa) and some archaea (methanogens). |