1.7: Producing Form- Development
( \newcommand{\kernel}{\mathrm{null}\,}\)
A feature of living things, and not just organisms, is that they are dynamic, they change, and they do so in a predictable way that can be described and observed over and over again in a variety of biological entities. This pattern of change through time is termed development and while the term is mostly associated with organisms, it is significant to realize that organized (i.e., controlled in some manner) change through time is seen in cells (e.g., the cell cycle, apoptosis), populations (e.g., logistic and exponential population growth) and communities (e.g., succession).
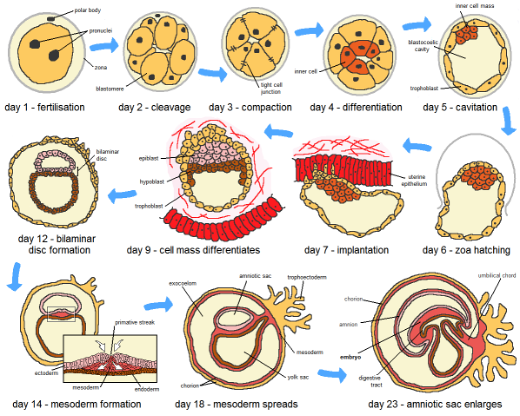
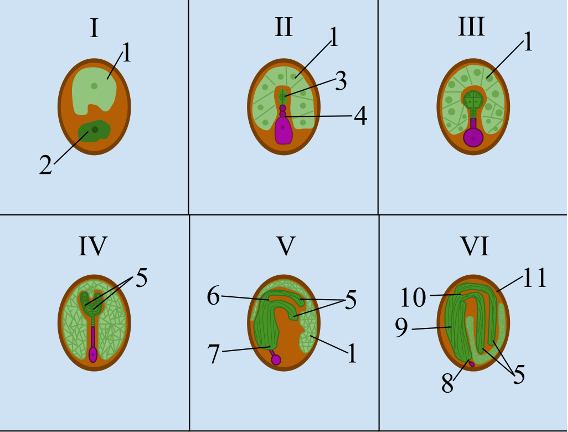
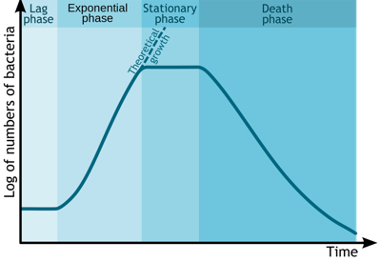
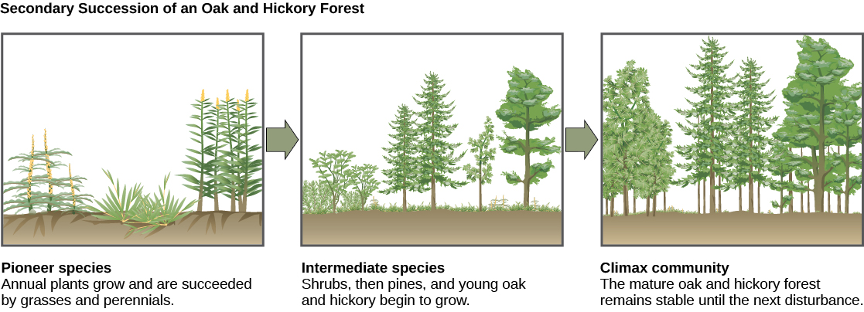
TOPICS
- Organism development
- Development in unicellular organisms
- Development of cell shape
- Development in colonial organisms
- Development in coenocytic/siphonaceous organisms
- Development in multicellular organisms
- Development in plants
Organism development
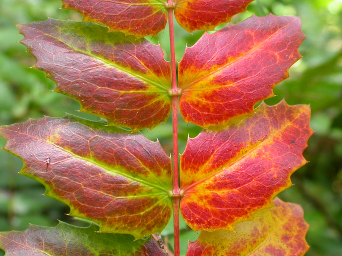
While development may include changes in a variety of aspects of an organism, from structure to physiology to behavior, we will focus here on how development produces and changes the structure and form of an organism throughout its lifetime. We have defined organisms as being distinct in time and space with a starting point and an ending point; thus , aspect s of development are the alterations in size, form and structure occurring during the organism 's lifespan. For most familiar organisms the starting point is a special cell that has the ability to proliferate, develop a complex structure, and grow to produce an ' end point ', a specific form that characterizes that particular creature. Many might think that this is the end of development, but for many organisms there are changes in the adult with time and sometimes the changes are dramatic and end the life of the organism. Some organisms ' self-destruct'as part of its developmental process , just as some cells do. Salmon die after spawning, as do wheat plants. In both cases the endpoint of the development al process is death. A corollary to this is that development does not just include growth and increases in complexity, it may also include, and in fact it often ends with, decreases in size and complexity and ultimately the end of the organism.Figure 6
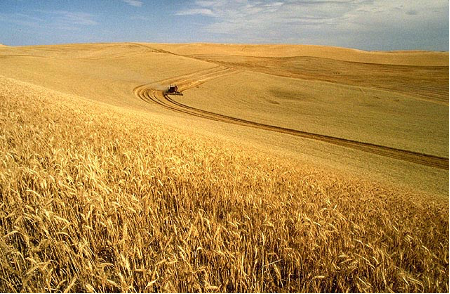
The field of development has exploded in recent years and is being examined from molecular and evolutionary perspectives in great detail. We are focused at a very different level, considering the very basic developmental features that bring about the form and structure of the biological entities we describe as organisms. We have described several different forms of organisms: unicellular, simple multicellular ( filamentous, sheets), coenocytic, complex multicellular. We will briefly consider how each of these forms develops. An important aspect of d evelopment for many organisms is the process of sex, involving the combining the genetic information of two organisms and subsequently halving it. Sex and reproduction are considered later in chapter 11.
Development in organisms that are unicellular
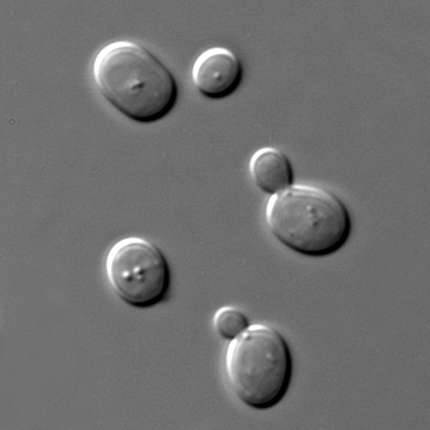
A number of organisms under consideration here (e.g., many bacteria , archaea, diatoms, dinoflagellates, and many green algae) are unicellular and, while their devel opment is quite different than for multicellular organisms, they do change over time and show definite patterns of development. Universally, development in unicellular organisms has two visible manifestations (there are many more developmental events that are not visible): cell division (Figure 7) and cell growth. These processes are components of the 'cell cycle' , a repeating series where cells acquire materials, synthesize molecules from them , and partition these materials into two daughter cells in the process of mitosis. While the most obvious molecule that needs to be made is DNA, there are a host of others: molecules for membranes, ribosomes and (for eukaryotic cells) for mitochondria and plastids. The construction of these materials requires that the cell obtain the elements that they are made of: carbon, nitrogen, phosphorus, etc., and part of the cell cycle involves the acquisition of these materials and the construction of the requisite molecules.
The relationship between cell division and cell growth is not the same in all organisms. Cells may divide repeatedly without any growth (e.g., in the early development of many animals). In this situation the original cell has plenty of 'materials' and the cell ular divisions simply partition these materials into daughter cells resulting in a multicellular organism that is basically the same size as the original fertilized egg. But this cannot go on forever, eventually there has to be material acquisition, reflected in an increase in mass and size (i.e., growth), to allow for continued cell division.
The main component of all cells is water and its acquisition is generally what drives cellular growth (i.e., expansion in size). For organisms with cell walls, water entry into the cell is affected one of two ways: by weakening the cell wall strength or by increasing the concentration of solutes, leading to an increase in internal pressure. This is discussed more in chapter 22, but for now one should appreciate that both these processes can be controlled by cellular activity. Consequently w ater absorption and cellular growth may occur as a distinct phase that occurs before or after other materials are obtained, or it can occur gradually. That is, the cell can: (1) grow steadily as both water and other materials are obtained; (2) divide, acquire materials other than water while remaining about the same size, and once enough materials other than water have been obtained then rapidly acquire water and grow or (3) divide, grow quickly by absorbing water and show little growth as it acquires materials other than water, and then divide again. In the first case there are a range of cell sizes and the age of a cell is correlated with its size.. In the second case, cells that are about to divide are recognizable because of their large size. In the third, case a population of cells would all be the same size except for some small cells that have just been produced by cell division.
The pattern shown by certain unicellular diatoms illustrates additional divers e possibilities. All d iatoms are enclosed in a silica shell with two halves; some are structured like a petri dish with a circular top half having a diameter slightly larger than that of the bottom half , allowing it to be a cover the bottom. When the cytosol divides (cytokinesis) the top half produces one daughter cell by grow ing a new bottom half and th is newly produced cell is 'fully grown' at its inception, i.e., it shows no growth. This cell, like all cells, goes through the G1 and G2 stages and during these stages 'materials are acquired and put together into biomolecules that can subsequently be partitioned between two daughter cells , but these phases , although often described as ' growth 1 ' and ' growth 2 ' do not involve an increase in cell size. Now consider the second daughter cell, the one that is associated with the old bottom dish, the smaller dish. This bottom dish becomes a ' top dish' as the cell generates a new bottom half. Like its sister cell it does not grow; it actually ends up slightly smaller than the parent cell because the bottom half of the original cell has become the top half of the daughter cell; consequently, this cell is slightly smaller than its parent (remember the bottom shell has to fit inside the top shell and hence must be smaller). Thus, after cell division, two cells are produced; one is the same size as the original cell and one is slightly smaller. Growth of individual cells is not occurring. Through time, the mean cell size of the diatom population becomes smaller and smaller until some critical minimum size is reached when sexual reproduction is triggered and results, among other things, in cells that are the same size as the original.
Exactly what triggers a cell to divide is a tightly controlled process that has been extensively studied because of its connection to cancer. But the phenomenon is important in other situations as well. F or example , in 'algal blooms' , whe re a population of alga e starts reproducing rapidly, producing very large populations whose existence often has very significant consequences. In both cancer and algal blooms, the importance of control mechanisms , other than nutrients, is indicated by the fact that nutrients alone may not trigger population/cancer growth : nutrients are necessary but not sufficient for growth. T he control of the process is not simply that cells divide when they acquire enough materials to form a second cell.
Development of cell shape
Part of the development process, for both unicellular organisms and the cells of multicellular organisms, involves the acquisition of a characteristic shape. For some cells, growth proceeds equally in all dimensions and small cells have a very similar shape to large ones, but for many cells growth is decidedly different in different directions and this produces 'adult' cells with characteristic shapes, distinct from the typically spherical/cuboidal shape of the newly produced cells. The attainment of this shape is an important feature of their development. For organisms with cell walls the shape of cells is determined by the relative strength of the cell wall in different directions. The cells grow by having an internal pressure that exceeds the cohesive strength of the cell wall. The wall yields and cellular growth occurs. If the strength of the cell wall is uniform then the cell expands uniformly (think of a typical balloon). But if the wall has less strength in a particular direction, then the expansion will occur in that direction, think of the specialty balloons that become long and skinny, this is a result of the balloon being much more resistant (higher cohesive strength) to radial expansion than to extension. The strength of cell walls is determined by the orientation of the cellulose microfibrils and this orientation is determined as they are deposited.
Development in organisms that are colonial
An essential aspect to unicellular development is that the daughter cells separate, a result of the fact that the junction between the 'new' cell and the 'old' one is weak and can be broken by forces in the environment or by forces that accompany the expansion of one or both of the daughter cells. If this does not happen cell division brings about an accumulation of cells that can drastically affect the functioning of each by changing the environment that they are exposed to and by changing form. Clusters of cells are considered colonies exhibiting 'simple multicellularity' . They are biological structures that do not nicely fit into the organism category, being somewhere between an organism and a population. Often colonies have characteristic shapes that develop as a consequence of patterns of cell division. Three basic patterns were described earlier that result from the control of the plane of cell division: filaments , sheets and spherical colonies . Each of these patterns has consequences for individual cells and their interaction with the environment. Spherical colonies result in some cells (those in the interior) that have very little contact with their environment, sheets and filaments represent situations where the colony of cells has more interaction with their environment although never as much as would be the case if the cell s had separated from their parent. While most colonial organisms are indeterminate, with no specific endpoint, growing to sizes that are dictated by biotic and abiotic conditions, there are some colonies that are determinate, producing a colony with a set number of cells and usually with a specific form.
Development in organisms that are coenocytic /siphonaceous
The vast majority of organisms that are large (a mm or more in length) are made of cells that are organized in a particular, repeatable way to produce form, i.e., they are multicellular and their development from a single cell involves the creation of cells in particular places to create a form. But there are a few of 'large' organisms whose form is not a consequence of an accumulation of cells but rather they are a single large cell that has developed to considerable size. A remarkable example of this type of growth is Acetabularia , a green algae that ranges up to several cm in size and is shaped like a parasol. Acetabularia , and most organisms that are coenocytic/siphonaceous, possess a cell wall and have internal pressures that develop as a result of osmotic forces. The shape that such cells produce are a result of the pattern of cellular expansion, which , as discussed above, is a consequence of relative strength of the wall in different directions. Bread mold produces parts (stolons, rhizoids, sporangiophores) that are all outgrowths of a single cell and there must be developmental controls that direct where and when outgrowths are produced and what structure is to be formed. Similarly, some siphonaceous green algae (e.g. Caulerpa )
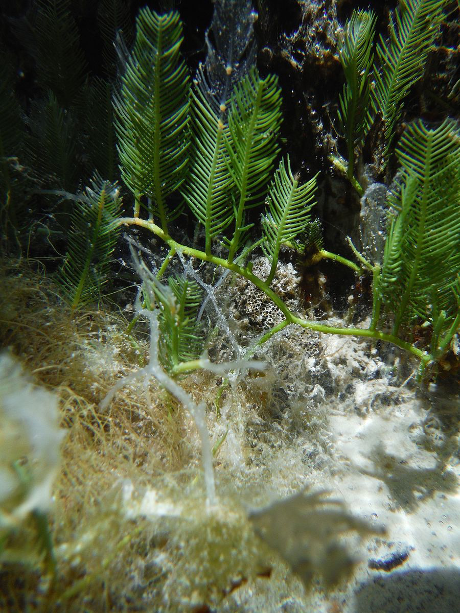
are capable of producing complex and large forms by controlling the form and direction of extensions off of a single cell (Figure 8). Some siphonaceous colonial forms are partly cellular in their construction: in Hydrodictyon individual cells are large (up to a centimeter) and multinucleate but are joined together into rings, commonly five or six-membered, to form a polygonal network.
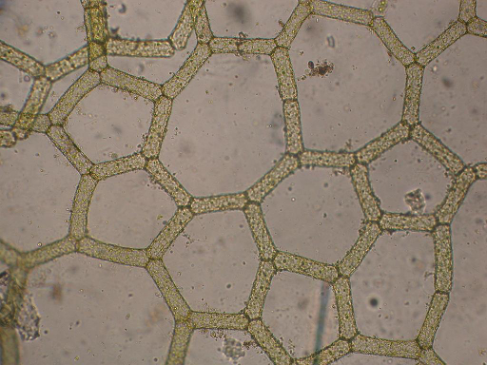
Development in the coenocytic plasmodial slime molds are particularly dramatic. Within a period of 20 hours a giant, multinucleate cell flowing in a network of channels and moving at rates of up to 1 cm per hour can transform into a rigid structure bounded with a cell wall and in the form of a miniature forest (~ 1-2 mm tall) of stalked structures that bear sporangia at their summit (Figure 10).
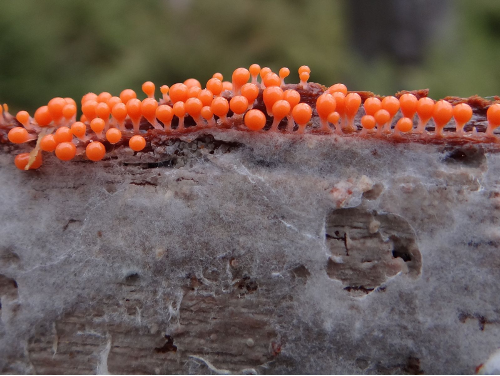
Development in multicellular organisms
Development in multicellular organisms is a much more complicated process. Multicellularity requires that an organism produce more than one type of cell. Except in the unusual case of cellular slime molds ( Dictyostelium ), all the cells of multicellular organisms have the same genetic makeup. T his means that there must control processes that dictate that some cells follow one set of instructions while other cells follow different instructions. Unicellular and colonial organisms may have the ability to do this to a limited extent. A lthough the majority of cells of these organisms are all the same, they may produce specialized cells to affect the sexual process, to carry specialized metabolic functions (e.g., the akinetes of cyanobacteria), or to accomplish dispersal. Complex multicellularity requires an additional significant feature besides the ability to produce different types of cells: the ability to organize multiple cell types in a three-dimensional pattern to form tissues and with tissues organized to form organs and with the organs organized to form organisms. This organization requires a developmental process that dictates what types of cells are produced, where they are produced, and to what extent and in what direction each cell expands.
A significant developmental distinction between animals and most of the organisms covered here results from the presence of the cell wall. Animal development includes the possibility of cell migration, with the movement of cells allowing for particular arrangements and forms to be produced. Outside of the animal kingdom cell migration occasionally occurs but only in organisms that lack cell walls. C ells of cellular slime mold s must migrate in order to aggregate and subsequently move relative to each other, and cellular slime mold development involves a physical rearrangement of cells. When a 'final' form of a cellular slime mold is produced the individual cells are no longer capable of moving because they have developed cell walls and are stuck to neighboring cells. Almost all of the multicellular organisms dealt with in this book possess cell walls and a newly produced cell is 'stuck' to the cell that produced it. Moreover, for all plants, cell division occurs in a region called a meristem, a region that produces new cells with a three-dimensional organization. The new cells that are produced are not only attached to their parental cell, but are also attached to multiple cells that are being simultaneously produced around them. In contrast, as discussed previously, fungi and some red, green and brown algae produce three-dimensional multicellular structures in ways that do not involve a meristem, generally by having individual filaments (one-dimensional structures) glued together in ways to form a three-dimensional form.
Development in plants
Plants (here used to include mosses, liverworts, hornworts and vascular plants) have several developmental features that are not found in familiar animals:
- Plant growth is generally indeterminate, i.e., without a defined endpoint
- Plants possess meristems, embryonic regions, throughout their life.
- Plants exhibit modular growth, a pattern also found in some less-familiar animals (e.g., hydroids, corals, sponges), but not in familiar animals.
- Plants show alternation of generations, meaning that there are two forms of the organism, one haploid and one diploid. Alternation of generations (Chapter 11) is also present in some green algae, brown algae, red algae and a few chytrids (fungi).
The first three points are considered below with the final point considered in a later section of the book.
Determinate and indeterminate growth and the growth of plants
By far the most familiar pattern of organismal development is that shown by mammals who grow for a short period of time (relative to the entire life of the organism) and then spend most of their life as an adult form. This type of development can be termed 'determinate' because the final form is 'determined' , there is an endpoint (an adult) to the development process. While some of the organisms covered in this course, in particular many of the unicellular forms, show this type of development, most 'non-animals' , and in particular plants and fungi, show a very different patterns of development, one that is described as indeterminate, where there is no endpoint and the organism is, in essence, everlasting. The key to its everlasting nature comes from the fact that that plants retain portions of their body that are permanently embryonic . In the familiar mammalian pattern of development an organism is an embryo for a portion of its life and then transforms into a juvenile and eventually an adult. An embryo is often defined as a 'young organism' but what makes it special is not its age but the fact that it cells are capable of cell division, cell differentiation and cellular growth. In animals these cellular abilities are only found for a short period of time. The whole animal is basically the same age and through time it transforms from an embryo into an adult. In order to observe development in animals one observes an embryo through time. While the adult does replace cells, in terms of development it is not changing in form. In contrast, plants always have regions that are embryonic and capable of dividing, differentiating and growing; and at any one point in time one can see the developmental process by moving from the embryonic parts of the plant to the older parts of the plant, i.e., the entire plant is NOT all the same age. If you planted an acorn ten years ago you might consider the structure that you now see is 'ten-years old' , but most of it is considerably younger and one can even find parts in the embryonic regions that were 'born yesterday' . At any point in time one can observe the entire development al process, from embryo to 'adult' , by moving distally, from the embryonic regions, located at the tips of roots and shoots , to progressively older tissues further from the tips.
The embryonic regions of plants are called meristems. There are two basic features that distinguish embryonic regions : (1) cells are dividing and thereby producing more cells, (2) cells are small, undifferentiated and not yet committed to a final cell type. The activity of meristems may vary seasonally , it often has periods of quiescence or dormancy, but its ability to produce more cells that develop into a variety of cells is intrinsic to the meristem region. Very early in the life of the plant the entire plant is embryonic but soon some of the cells become developmentally programmed to mature into particular types of cells and , along with this, lose their ability to divide. In seed plants this transformation takes place within the seed and a mature seed has within it a small plant with two embryonic regions on opposite ends of a very short root/shoot axis. Throughout the life of the plant this axis will extend because of the expansion of the cells produced at the two ends. The meristem s produce more and more cells and the expansion of these cells pushes the two meristem s further away from each other and elongates the root and shoot. The se embryonic regions are called primary meristems (they are the first ones formed) and also apical meristems (they are located at the tips, apices, of roots and shoots). Root apical meristems divide to produce cells that extend the root axis. Shoot apical meristems serve a similar function for shoots but while producing new shoot material they are also producing leaves. Growth from apical meristems is called primary growth . In general, the oldest part of the plant is at the soil surface and one encounters younger and younger tissues as one moves towards the tips of shoots or tips of roots. The actual region of growth is generally restricted to a small portion, typically a centimeter or less, just below the meristematic regions.
Plant growth is modular
In addition to being indeterminate, plant growth is modular, producing structures that are fundamentally self-similar and recursive. There are two basic modules to a vascular plant: roots and shoots, and both can produce new modules, branch roots and branch shoots, that are replicas of the original shoot axis and root axis. With time, a seemingly complex entity is produced, yet the rules governing its construction are very simple: the shoot can generate branches and these can generate more branches and these can generate even more branches. The same thing is true for roots. The growth of all the modules results from the activities of apical meristems and all such growth is considered primary growth.
Figure 11 Modular plant growth: Plants grow in a modular fashion with two basic modules: shoots (which bear leaves) and roots. Both of these modules have at their tip a meristematic zone (shoot apical meristem and root apical meristem). These modules are capable of producing additional modules, branch roots and branch shoots and these in turn can produce more branches, i.e., the branches have branches. It is a recursive structure. The original root and shoot meristems are created in the seed. Branch shoots originate in specific places, adjacent to where the leaf was or is attached to the stem. A group of meristematic cells, called a bud (branch) primordium is left behind by the apical meristem each time that it produces a leaf. These generally do not develop immediately and although a potential branch is produced at every leaf junction, many of them never develop. The production of branch roots is not as rigidly determined. The growth produced by apical meristems is considered 'primary growth' whether it occurs on the original root or shoot or occurs on branch roots or shoots.
Leaf development: In contrast to the roots and shoots, whose growth is indeterminate, leaves are determinate, producing a defined form, the product of a structure with a defined (determined) development process. The shoot apical meristem produces mounds of embryonic tissue, called leaf primordia, that divide for a period of time, grow for a period of time and then exist in a static form until the process of senescence is triggered that culminates with the leaf becoming detached from the rest of the plant.
Origin of new modules. To create a new module, one needs to create a new apical meristem. These originate differently in roots and shoots. In roots, new apical meristems form as the result of the activity of certain cells in the outermost layer of the central core of root vascular tissue (more details in the next chapter). Some of these cells are stimulated to start dividing and become organized to form a root apical meristem. Growth of cells produced by this meristem pushes the branch root apical meristem out of the original root and into the soil, where it continues to grow in a fashion similar to the original root.
Branch shoots are created in a differently and originate from a shoot apical meristem. In addition to extending the shoot and producing embryonic leaves, the shoot apical meristem also produces a new shoot apical meristems in the 'axil' of each leaf. These are new shoot apical meristems and are called bud primordia. They are produced in a dormant state and need to be stimulated to start dividing and produce new cells that will allow the branch to elongate. Most bud primordia are never stimulated to grow; if they all were stimulated a very 'branchy' structure would result, with a branch being produced at every position where there was or is a leaf. Often, but certainly not always, branch shoots are only stimulated to grow after the leaf they are adjacent to has fallen off the plant.
Plants are also capable of producing new modules in non-standard ways. Roots and shoots thus produced are termed 'adventitious' . Sometimes roots are produced from stem tissues and these would be described as adventitious roots because all 'standard' roots are formed off existing roots. Similarly, sometimes root tissues can develop shoots; obviously these are not originating in the axil of a leaf, thus these would be termed adventitious shoots. The ability of some plants to produce adventitious roots or shoots allows for the vegetative propagation of plants: if detached stems can be stimulated to produce roots then one can propagate plants from 'cuttings' ; similarly, roots can be used to propagate plants. And for some plants leaf tissue can be stimulated to produce adventitious roots and shoots; thus, propagation is sometimes possible from leaves. In all of these situations, certain cells, called parenchyma cells, are stimulated to start dividing and organize to form an apical meristem. Once one module (a root or a shoot) is started, new modules can be formed from it. A unique feature of parenchyma cells is their ability to 'de-differentiate' and resume an embryonic condition, capable of dividing and producing a variety of cell types, even after they have differentiated to become parenchyma cells.
Cell types of vascular plants, along with the anatomy of roots, stems and leaves will be discussed in greater detail in the next chapter.