4.5.1.4: Water Absorption
- Page ID
- 32038
\( \newcommand{\vecs}[1]{\overset { \scriptstyle \rightharpoonup} {\mathbf{#1}} } \)
\( \newcommand{\vecd}[1]{\overset{-\!-\!\rightharpoonup}{\vphantom{a}\smash {#1}}} \)
\( \newcommand{\id}{\mathrm{id}}\) \( \newcommand{\Span}{\mathrm{span}}\)
( \newcommand{\kernel}{\mathrm{null}\,}\) \( \newcommand{\range}{\mathrm{range}\,}\)
\( \newcommand{\RealPart}{\mathrm{Re}}\) \( \newcommand{\ImaginaryPart}{\mathrm{Im}}\)
\( \newcommand{\Argument}{\mathrm{Arg}}\) \( \newcommand{\norm}[1]{\| #1 \|}\)
\( \newcommand{\inner}[2]{\langle #1, #2 \rangle}\)
\( \newcommand{\Span}{\mathrm{span}}\)
\( \newcommand{\id}{\mathrm{id}}\)
\( \newcommand{\Span}{\mathrm{span}}\)
\( \newcommand{\kernel}{\mathrm{null}\,}\)
\( \newcommand{\range}{\mathrm{range}\,}\)
\( \newcommand{\RealPart}{\mathrm{Re}}\)
\( \newcommand{\ImaginaryPart}{\mathrm{Im}}\)
\( \newcommand{\Argument}{\mathrm{Arg}}\)
\( \newcommand{\norm}[1]{\| #1 \|}\)
\( \newcommand{\inner}[2]{\langle #1, #2 \rangle}\)
\( \newcommand{\Span}{\mathrm{span}}\) \( \newcommand{\AA}{\unicode[.8,0]{x212B}}\)
\( \newcommand{\vectorA}[1]{\vec{#1}} % arrow\)
\( \newcommand{\vectorAt}[1]{\vec{\text{#1}}} % arrow\)
\( \newcommand{\vectorB}[1]{\overset { \scriptstyle \rightharpoonup} {\mathbf{#1}} } \)
\( \newcommand{\vectorC}[1]{\textbf{#1}} \)
\( \newcommand{\vectorD}[1]{\overrightarrow{#1}} \)
\( \newcommand{\vectorDt}[1]{\overrightarrow{\text{#1}}} \)
\( \newcommand{\vectE}[1]{\overset{-\!-\!\rightharpoonup}{\vphantom{a}\smash{\mathbf {#1}}}} \)
\( \newcommand{\vecs}[1]{\overset { \scriptstyle \rightharpoonup} {\mathbf{#1}} } \)
\( \newcommand{\vecd}[1]{\overset{-\!-\!\rightharpoonup}{\vphantom{a}\smash {#1}}} \)
Learning Objectives
- Explain the function of root hairs.
- Define root pressure and explain its mechanism.
- Contrast the three pathways of water movement through the roots and identify each cell type or tissue involved.
Most plants secure the water and minerals they need from their roots. Water moves from the soil to the roots, stems, and ultimately the leaves, where transpiration occurs. The roots absorb enough water to compensate for water lost to transpiration. Rapid absorption is aided by root hairs, which extend from epidermal cells, increasing surface area (Figure \(\PageIndex{1}\)). As discussed earlier in this chapter, roots draw water from the soil because they have lower water potential than the soil. Much of this difference in water potential is an indirect result of transpiration, but roots can also water potential by decreasing solute potential.
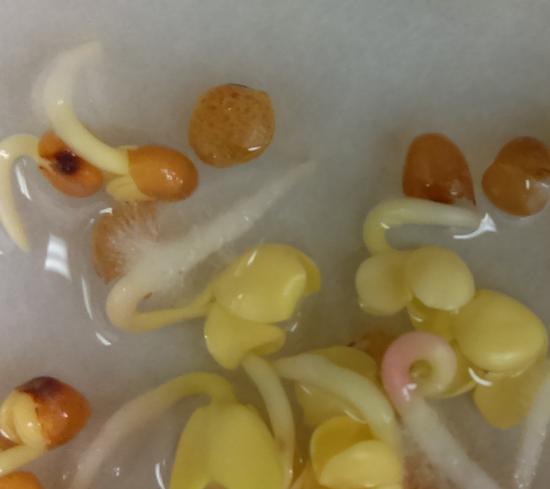
Root Pressure
When a tomato plant is carefully severed close to the base of the stem, sap oozes from the stump (Figure \(\PageIndex{2}\)). The fluid comes out because roots are constantly absorbing water, drawing it into the vascular cylinder, and pushing it up the xylem. This is called root pressure, and it is created by the osmotic pressure of solutes trapped in the vascular cylinder by the Casparian strip.
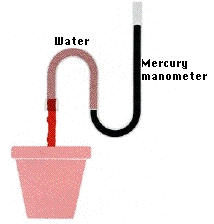
Although root pressure plays a small role in the transport of water in the xylem in some plants and in some seasons, it does not account for most water transport. As evidence, few plants develop root pressures greater than ~0.2 kPa, and some develop no root pressure at all. Additionally, the volume of fluid transported by root pressure is not enough to account for the measured movement of water in the xylem of most trees and vines. Furthermore, the highest root pressures occur in the spring, but water moves through the xylem most rapidly in the summer (when transpiration is high).
As discussed in the Cohesion-Tension Theory section, transpiration, rather than root pressure, is typically the driving force for upward water movement in a plant. However, when transpiration rates are very low, such as in cool and humid weather, root pressure pushes water up the xylem faster than water is lost through the stomata. As a result water droplets are forced out of openings on the leaf margin, a phenomenon called guttation (Figure \(\PageIndex{3}\)). Droplets resulting from guttation are not to be confused with dew droplets, which result from the condensation of water vapor when the air becomes colder and has less capacity to hold water. In other words, guttation results from water that was inside of the plant, but dew droplets form from water vapor that was in the surrounding air.
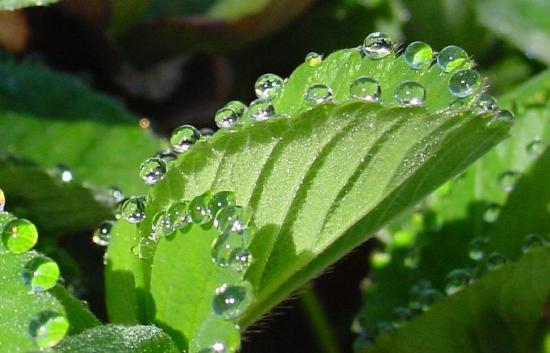
Pathways of Water Movement
Water can move through the roots by three separate pathways: apoplast, symplast, and transmembrane (transcellular). In the apoplast pathway (apoplastic route), water moves through the spaces between the cells and in the cells walls themselves. In the symplast pathway (symplastic route), water passes from cytoplasm to cytoplasm through plasmodesmata (Figure \(\PageIndex{4}\)). In the transmembrane pathway, water crosses plasma membranes, entering and exiting each cell. Water moving through the transmembrane pathway thus moves through both the symplast (interconnected cytoplasms) and apoplast (cell walls and spaces in between cells). Water may also cross the tonoplast, entering the central vacuole as part of the transmembrane pathway.
Water from the soil is absorbed by the root hairs of the epidermis and then moves through the cortex through one of the three pathways. However, the inner boundary of the cortex, the endodermis, is impervious to water due to the Casparian strip. Regardless of how the water moved up to this point (apoplast, symplast, transmembrane), it must enter the cytoplasms of the endodermal cells. From here it can pass via plasmodesmata into the cells of the vascular cylinder (stele). Once inside, water is again free to move through the apolast, the symplast, or both (transmembrane).
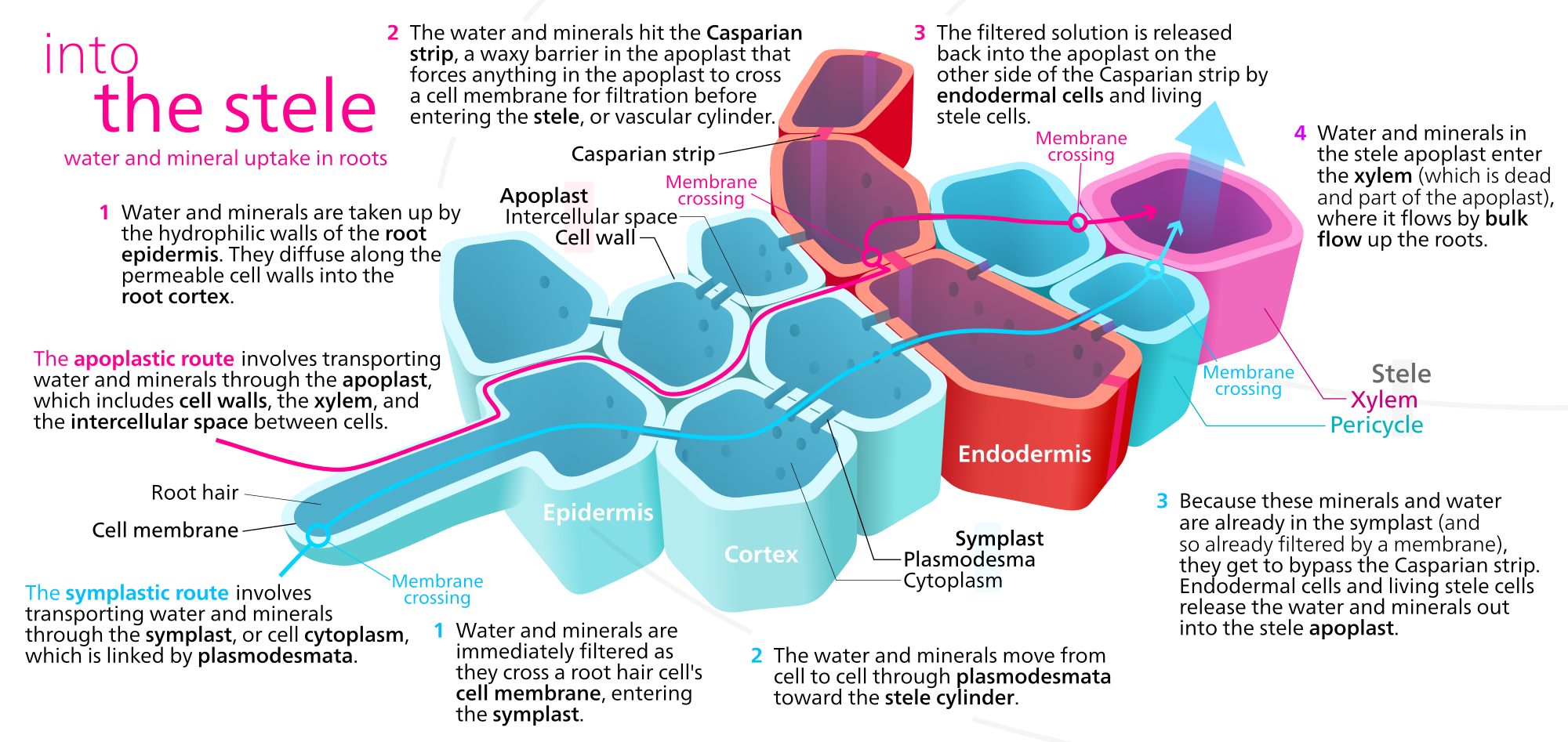
Once in the xylem, water with the minerals that have been deposited in it (as well as occasional organic molecules supplied by the root tissue) move up in the vessel elements and tracheids. At any level, the water can leave the xylem and pass laterally to supply the needs of other tissues. At the leaves, the xylem passes into the petiole and then into the veins of the leaf. Water leaves the finest veins and enters the cells of the spongy and palisade layers. Here some of the water may be used in metabolism, but most is lost in transpiration.
Figure \(\PageIndex{4}\) illustrates minerals moving through the apoplast or symplast, but minerals typically move through the symplast. Minerals enter the root by active transport into the symplast of epidermal cells and move toward and into the vascular cylinder through the plasmodesmata connecting the cells. They enter the conducting cells of the xylem from the pericycle and other parenchyma cells via active transport through specialized transmembrane channels.
Attributions
Curated and authored by Melissa Ha using the following sources:
- 16.2A Xylem from Biology by John. W. Kimball (licensed CC-BY)
- 30.5 Transport of Water and Solutes in Plants from Biology 2e by OpenStax (licensed CC-BY). Access for free at openstax.org.