4.1.3: Natural Selection and Adaptive Evolution
- Page ID
- 83854
Natural Selection and Adaptive Evolution
- Please read and watch the following Mandatory Resources
- Reading the material for understanding, and taking notes during videos, will take approximately 1.5 hours.
- Optional Activities are embedded.
- To navigate to the next section, use the Contents menu at the top of the page OR the right arrow on the side of the page.
- If on a mobile device, use the Contents menu at the top of the page OR the links at the bottom of the page.
- Explain how natural selection leads to adaptive evolution
- Contrast stabilizing selection, directional selection, and diversifying selection.
- Describe frequency-dependent selection
- Explain the effects of sexual dimorphism on the reproductive potential of an organism
Introduction to Natural Selection
Natural selection drives adaptive evolution by selecting for and increasing the occurrence of beneficial traits in a population. Natural selection only acts on the population’s heritable traits: selecting for beneficial alleles and, thus, increasing their frequency in the population, while selecting against deleterious alleles and, thereby, decreasing their frequency. This process is known as adaptive evolution (Figure \(\PageIndex{1}\)). Natural selection does not act on individual alleles, however, but on entire organisms. An individual may carry a very beneficial genotype with a resulting phenotype that, for example, increases the ability to reproduce. If that same individual also carries an allele that results in a fatal childhood disease, however, the fecundity phenotype will not be passed on to the next generation because the individual will not live to reach reproductive age. Natural selection acts at the level of the individual. It selects for individuals with greater contributions to the gene pool of the next generation, known as an organism’s evolutionary fitness.
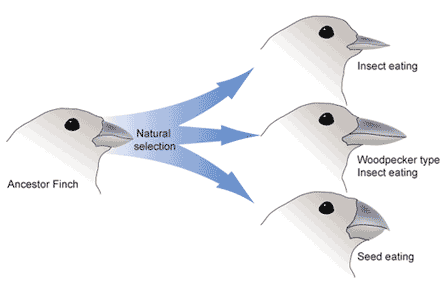
Evolutionary fitness is often quantifiable as the contribution of genetic information by an individual to subsequent generations. However, it is not the absolute fitness of an individual that counts, but rather how it compares to the other organisms in the population. This concept, called relative fitness, allows researchers to determine which individuals are contributing additional offspring to the next generation and, thus, how the population might evolve.
Genetic Variation and Natural Selection
Natural selection and some of the other evolutionary forces can only act on heritable traits, namely an organism’s genetic code. Because alleles are passed from parent to offspring, those that confer beneficial traits or behaviors may be selected for, while deleterious alleles may be selected against. Acquired traits, for the most part, are not heritable. For example, if an athlete works out in the gym every day, building up muscle strength, the athlete’s offspring will not necessarily grow up to be a bodybuilder. If there is a genetic basis for the ability to run fast, on the other hand, this may be passed to a child.
Genetic variation is a measure of the genetic differences (diversity of alleles and genotypes) that exist within a population. The genetic variation of an entire species is often called genetic diversity. Genetic variations are the differences in DNA segments or genes between individuals and each variation of a gene is called an allele. For example, a population with many different alleles at a single chromosome locus has a high amount of genetic variation. Genetic variation is essential for natural selection because natural selection can only increase or decrease the frequency of alleles that already exist in the population.
Genetic variation is caused by:
- mutation (discussed further in Section 2.2.4)
- random mating between organisms (discussed further in Section 2.2.4)
- random fertilization
- genetic recombination during meiosis
Mutations are the only method of creating new alleles; mutations are ultimately the engine that drives evolution. Sexual reproduction (including random mating, random fertilization, and crossing over) are ways to reshuffle and recombine those genes in new ways, giving offspring combinations that differ from their parents and from others.
This 4-minute video provides a brief introduction to variation in humans and a method for identifying traits that are encoded by genetics and those determined by the environment.
Question after watching: In most cases, when a person who has an identical two develops a disease like cancer, their identical twin does not. What does this tell you about the causes of cancer?
Evolution and Adaptation to the Environment
Variation allows some individuals within a population to adapt to the changing environment. Because natural selection acts directly only on phenotypes, more genetic variation within a population usually enables more phenotypic variation. Some new alleles increase an organism’s ability to survive and reproduce, which then ensures the survival of the allele in the population. Other new alleles may be immediately detrimental (such as a malformed oxygen-carrying protein) and organisms carrying these new mutations will die out. Neutral alleles are neither selected for nor against and usually remain in the population. Genetic variation is advantageous because it enables some individuals and, therefore, a population, to survive despite a changing environment (Figure \(\PageIndex{2}\)).
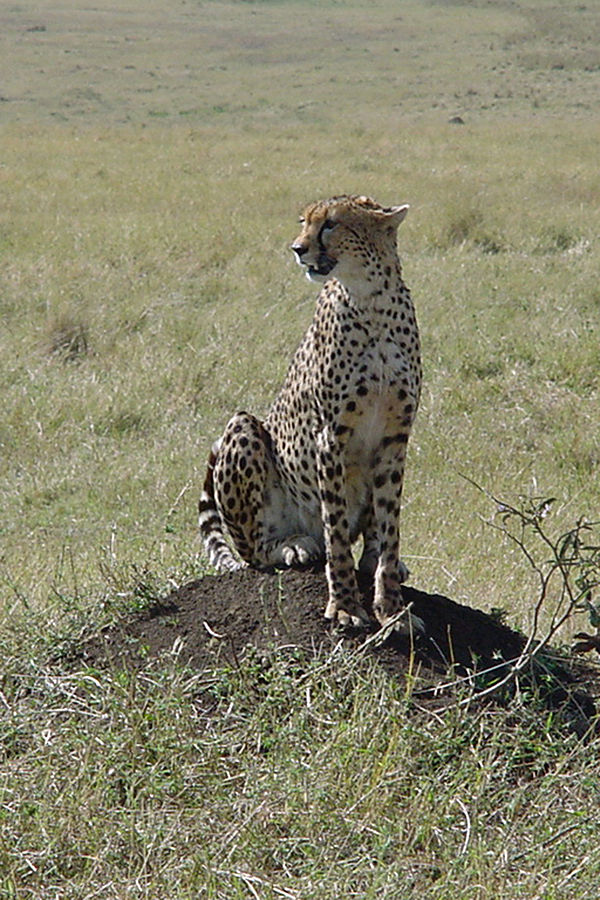
Geographic Variation
Some species display geographic variation as well as variation within a population. Geographic variation, or the distinctions in the genetic makeup of different populations, often occurs when populations are geographically separated by environmental barriers or when they are under selection pressures from a different environment. One example of geographic variation are clines, gradual changes in a trait across an organism's geographic distribution.
Species of warm-blooded animals, for example, tend to have larger bodies in the cooler climates closer to the earth’s poles, allowing them to better conserve heat. This is considered a latitudinal cline (Figure \(\PageIndex{3}\)). Alternatively, flowering plants tend to bloom at different times depending on where they are along the slope of a mountain, known as an altitudinal cline.
Environmental Variance
Genes are not the only players involved in determining population variation. Phenotypes are also influenced by other factors, such as the environment (Figure \(\PageIndex{4}\)). Some major characteristics, such as gender, are determined by the environment for some species. For example, some turtles and other reptiles have temperature-dependent sex determination (TSD). TSD means that individuals develop into males if their eggs are incubated within a certain temperature range or females at a different temperature range.
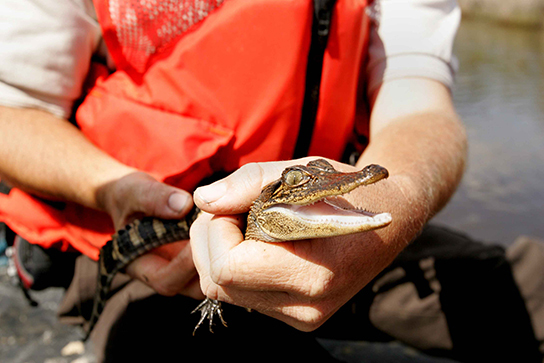
Types of Natural Selection
There are several ways selection can affect population variation (each is described in detail below) (Figure \(\PageIndex{9}\)):
- stabilizing selection
- directional selection
- diversifying selection
- frequency-dependent selection
- sexual selection
As natural selection influences the traits in a population, individuals can either become more or less genetically similar to one another and the phenotypes displayed can become more similar or more disparate. In the end, natural selection cannot produce perfect organisms from scratch, it can only generate populations that are better adapted to survive and successfully reproduce in their environments through the aforementioned selections.
In this 3-minute video, David Attenborough explains how iguana evolved to swim on the Galpagos islands.
Question after watching: Propose a series of steps that could have led to this evolution in the iguana population in the Galpagos. Specifically, explain how they evolved to live for short periods underwater from their land ancestor.
Stabilizing, Directional, and Diversifying Selection
Stabilizing Selection
If natural selection favors an average phenotype by selecting against extreme variation, the population will undergo stabilizing selection (Figure \(\PageIndex{5}\)).
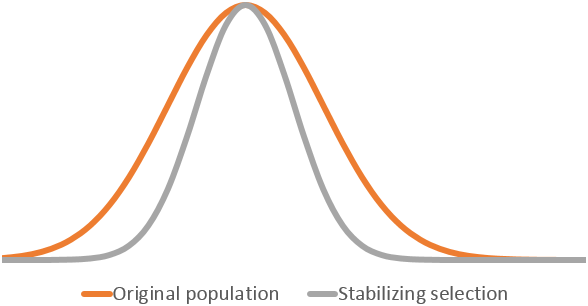
For example, in a population of mice that live in the woods, natural selection will tend to favor individuals that best blend in with the forest floor and are less likely to be spotted by predators. Assuming the ground is a fairly consistent shade of brown, those mice whose fur most closely matches that color will most probably survive and reproduce, passing on their genes for their brown coat. Mice that carry alleles that make them slightly lighter or slightly darker will stand out against the ground and will more probably die from predation. As a result of this stabilizing selection, the population’s genetic variance will decrease.
Directional Selection
When the environment changes, populations will often undergo directional selection, which selects for phenotypes at one end of the spectrum of existing variation (Figure \(\PageIndex{6}\)).

A classic example of this type of selection is the evolution of the peppered moth (Biston betularia) in eighteenth- and nineteenth-century England. Prior to the Industrial Revolution, the moths were predominately light in color, which allowed them to blend in with the light-colored trees and lichens in their environment. As soot began spewing from factories, the trees darkened and the light-colored moths became easier for predatory birds to spot.
Over time, the frequency of the melanic (dark) form of the moth increased because their darker coloration provided camouflage against the sooty tree; they had a higher survival rate in habitats affected by air pollution (Figure \(\PageIndex{7}\)). Similarly, a hypothetical mouse population may evolve to take on a different coloration if their forest floor habitat changed. The result of this type of selection is a shift in the population’s genetic variance toward the new, fit phenotype.
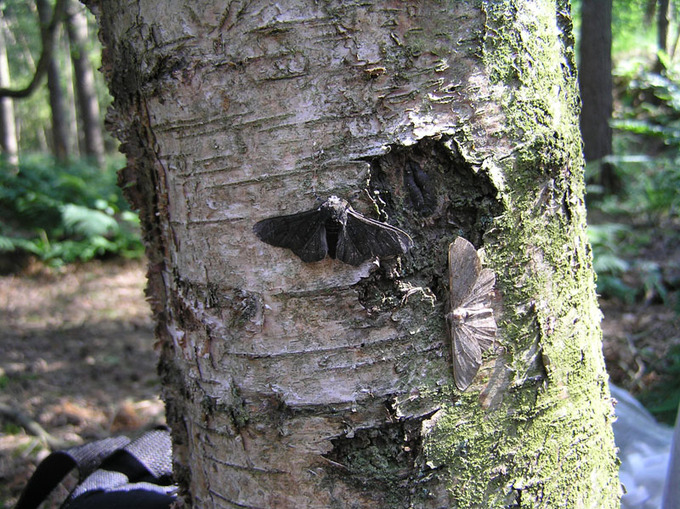
Diversifying (or Disruptive) Selection
Sometimes natural selection can select for two or more distinct phenotypes that each have their advantages in diversifying (disruptive) selection. In these cases, the intermediate phenotypes are often less fit than their extreme counterparts (Figure \(\PageIndex{8}\)).

This is seen in many populations of animals that have multiple male mating strategies, such as lobsters. Large, dominant alpha males obtain mates by brute force, while small males can sneak in for furtive copulations with the females in an alpha male’s territory. In this case, both the alpha males and the “sneaking” males will be selected for, but medium-sized males, which cannot overtake the alpha males and are too big to sneak copulations, are selected against.
Diversifying selection can also occur when environmental changes favor individuals on either end of the phenotypic spectrum. Imagine a population of mice living at the beach where there is light-colored sand interspersed with patches of tall grass. In this scenario, light-colored mice that blend in with the sand would be favored, as well as dark-colored mice that can hide in the grass. Medium-colored mice, on the other hand, would not blend in with either the grass or the sand and, thus, would more probably be eaten by predators. The result of this type of selection is increased genetic variance as the population becomes more diverse.
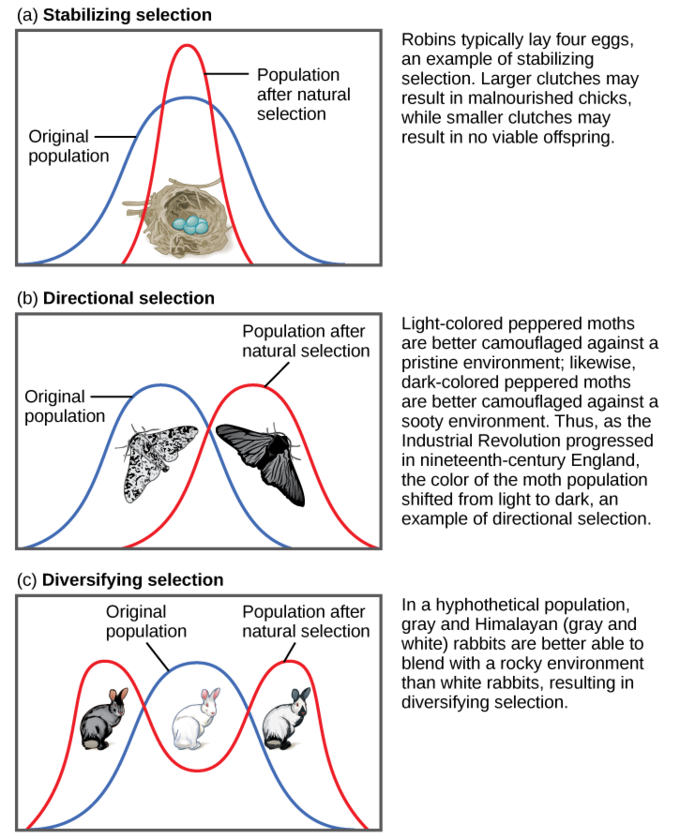
This 2.5-minute video highlights directional, stabilizing, and disruptive selection.
Question after watching: In the video, the narrator speaks of disruptive selection (this textbook called it diversifying selection). What is being disrupted?
Frequency-Dependent Selection
Another type of selection, called frequency-dependent selection, favors phenotypes that are either common (positive frequency-dependent selection) or rare (negative frequency-dependent selection).
Negative Frequency-Dependent Selection
Negative frequency-dependent selection increases the population’s genetic variance by selecting for rare phenotypes. An interesting example of this type of selection is seen in a unique group of lizards in the deserts of the United States. Male common side-blotched lizards come in three throat-color patterns: orange, blue, and yellow. Each of these forms has a different reproductive strategy: orange males are the strongest and can fight other males for access to their females; blue males are medium-sized and form strong pair bonds with their mates; and yellow males are the smallest and look a bit like female, allowing them to sneak copulations.
Like a game of rock-paper-scissors, orange beats blue, blue beats yellow, and yellow beats orange in the competition for females. The big, strong orange males can fight off the blue males to mate with the blue’s pair-bonded females; the blue males are successful at guarding their mates against yellow sneaker males; and the yellow males can sneak copulations from the potential mates of the large, polygynous orange males (Figure \(\PageIndex{10}\)).
In this scenario, orange males will be favored by natural selection when the population is dominated by blue males, blue males will thrive when the population is mostly yellow males, and yellow males will be selected for when orange males are the most populous. As a result, populations of side-blotched lizards cycle in the distribution of these phenotypes. In one generation, orange might be predominant and then yellow males will begin to rise in frequency. Once yellow males make up a majority of the population, blue males will be selected for. Finally, when blue males become common, orange males will once again be favored. So, orange (strong) > blue (cooperation) > yellow (sneaky) > orange (strong)...etc.
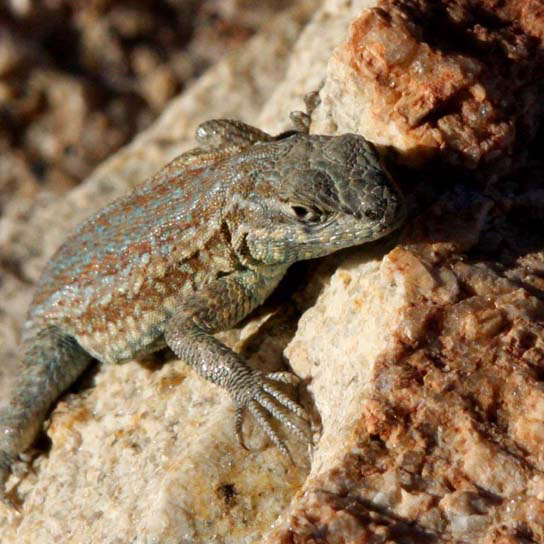
This 3.5-minute video illustrates the side-blotched lizard case study of negative frequency-dependent selection.
Question after watching: The video mentions that the blues can be altruistic, which is to say exhibit behavior that would benefit not themselves but another animal. Propose a hypothesis about the evolutionary advantage of altruism in this case.
Positive Frequency-dependent Selection
Positive frequency-dependent selection usually decreases genetic variance by selecting for common phenotypes. An example of positive frequency-dependent selection is the mimicry of the warning coloration of dangerous species of animals by other species that are harmless.
The scarlet kingsnake (Lampropeltis elapsoides), a harmless species, mimics the coloration of the eastern coral snake (Micrurus fulvius), a venomous species typically found in the same geographical region (Figure \(\PageIndex{11}\)). Predators learn to avoid both species of snake due to the similar coloration. As a result, the scarlet kingsnake becomes more common and its coloration phenotype becomes more variable due to relaxed selection. This phenotype is, therefore, more “fit” as the population of species that possess it (both dangerous and harmless) becomes more numerous. In geographic areas where the coral snake is less common, the pattern becomes less advantageous to the kingsnake, and much less variable in its expression, presumably because predators in these regions are not “educated” to avoid the pattern.
In this short 1-minute narrated by David Attenborough, you will see several examples of biomimicry, where animals evolved shapes and colors that make them look like something else. This is a type of positive frequency-dependent selection.
Question after watching: What appears to be the purpose of biomimicry in most organisms? At which step in a process of natural selection would mimicry provide an advantage?
Sexual Selection
Sexual selection, the selection pressure on males and females in mating, can result in traits designed to maximize sexual success. In species with sexual selection, typically one sex is less-limited due to their ability to produce an overabundance of gametes and the other is more-limited due to their higher parental investment (e.g. produce eggs, gestate a fetus, raise young, etc.). The more-limited sex typically reproduces fewer times and has higher parental investment than the less-limited sex; they are typically more discerning about who they mate with in order to optimize those reproductive events.
Sexual selection in animals takes two major forms: intersexual selection in which, the less-limited sex (typically males) are chosen by the more-limited sex (typically females) based on certain characteristics and intrasexual selection in which members of the less-limited sex compete aggressively among themselves for access to the more-limited sex (Figure \(\PageIndex{12}\)).
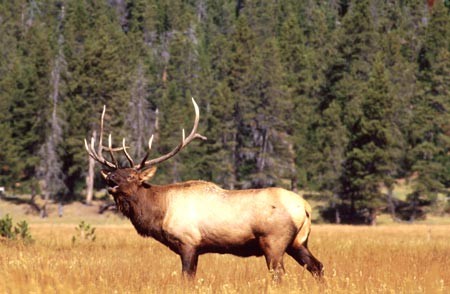
This 3-minute video highlights extreme behaviors that arise from intense sexual selection pressure.
Question after watching: What can you surmise about which of the sexes are 'choosier' in most mammals, based on morphological and behavioural dimorphism?
Sexual Dimorphism in Animals
Males and females of certain animal species are often quite different from one another in ways beyond the reproductive organs. Male vertebrates are often larger, for example, and display many elaborate colors, adornments, and behaviors such as seen in the figure below, while females of the same species tend to be smaller and less attention-seeking. These differences are called sexual dimorphisms and arise from the variation in reproductive success (Figure \(\PageIndex{13}\)).
Animal females do not have trouble finding mates for reproduction, while reproduction is not guaranteed for males. The bigger, stronger, or more decorated males usually obtain the vast majority of the total reproductive events, while other males receive none. This can occur because the males are better at fighting off other males (intrasexual selection), or because females will choose to mate with the bigger, brasher, or more decorated males (intersexual selection). In either case, this variation in reproductive success generates a strong selection pressure among males to compete for mates, resulting in the evolution of bigger body sizes or elaborate ornaments in order to increase their chances of mating. Females, on the other hand, tend to get a handful of selected matings; therefore, they are more likely to be selective in their mates.
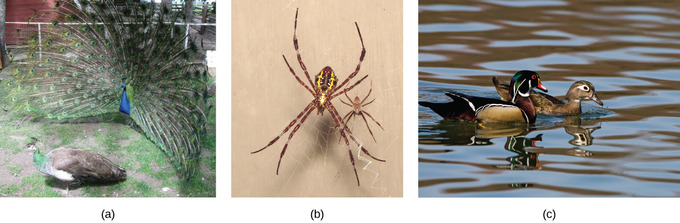
Sexual dimorphism varies widely among species; some species are even sex-role reversed. In such cases, females tend to have a greater variation in their reproductive success than males and are, correspondingly, selected for the bigger body size and elaborate traits usually characteristic of males.
The Handicap Principle
Sexual selection can be so strong that it selects for traits that are actually detrimental to the individual’s survival because they maximize its reproductive success. For example, while the male peacock’s tail is beautiful, and the male with the largest, most colorful tail will more probably win a female's attention, it is not practical or advantageous (Figure \(\PageIndex{14}\)). In addition to making the males more visible to predators, it slows down any needed escapes. There is some evidence that this risk is why females like the big, bright tails. Because large tails carry risk, only the best males can survive that risk, and therefore the bigger the tail the more fit the male must be. This idea is known as the handicap principle.
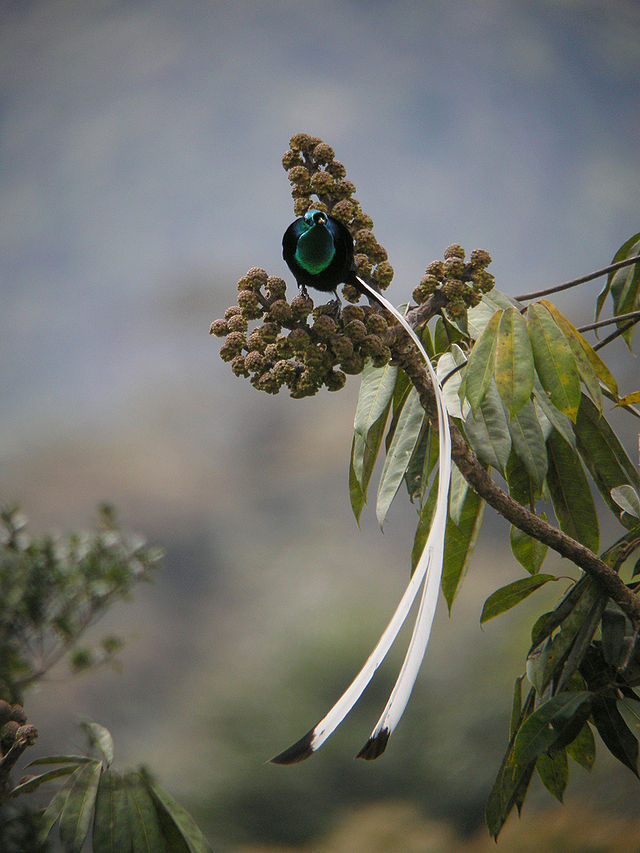
The Good Genes Hypothesis
The good genes hypothesis states that males develop these impressive ornaments to show off their efficient metabolism or their ability to fight disease. Females then choose males with the most impressive traits because it signals their genetic superiority, which they will then pass on to their offspring. Though it might be argued that females should not be so selective because it will likely reduce their number of offspring, if better males father more fit offspring, it may be beneficial. Fewer, healthier offspring may increase the chances of survival more than many, weaker offspring.
This 8.5-minute video is an overview of natural and sexual selection.
Questions after watching: How is sexual selection an example or type of natural selection? Explain the following statement: “evolution is just a numbers game”