17.3: Osmosis
- Page ID
- 89009
\( \newcommand{\vecs}[1]{\overset { \scriptstyle \rightharpoonup} {\mathbf{#1}} } \)
\( \newcommand{\vecd}[1]{\overset{-\!-\!\rightharpoonup}{\vphantom{a}\smash {#1}}} \)
\( \newcommand{\id}{\mathrm{id}}\) \( \newcommand{\Span}{\mathrm{span}}\)
( \newcommand{\kernel}{\mathrm{null}\,}\) \( \newcommand{\range}{\mathrm{range}\,}\)
\( \newcommand{\RealPart}{\mathrm{Re}}\) \( \newcommand{\ImaginaryPart}{\mathrm{Im}}\)
\( \newcommand{\Argument}{\mathrm{Arg}}\) \( \newcommand{\norm}[1]{\| #1 \|}\)
\( \newcommand{\inner}[2]{\langle #1, #2 \rangle}\)
\( \newcommand{\Span}{\mathrm{span}}\)
\( \newcommand{\id}{\mathrm{id}}\)
\( \newcommand{\Span}{\mathrm{span}}\)
\( \newcommand{\kernel}{\mathrm{null}\,}\)
\( \newcommand{\range}{\mathrm{range}\,}\)
\( \newcommand{\RealPart}{\mathrm{Re}}\)
\( \newcommand{\ImaginaryPart}{\mathrm{Im}}\)
\( \newcommand{\Argument}{\mathrm{Arg}}\)
\( \newcommand{\norm}[1]{\| #1 \|}\)
\( \newcommand{\inner}[2]{\langle #1, #2 \rangle}\)
\( \newcommand{\Span}{\mathrm{span}}\) \( \newcommand{\AA}{\unicode[.8,0]{x212B}}\)
\( \newcommand{\vectorA}[1]{\vec{#1}} % arrow\)
\( \newcommand{\vectorAt}[1]{\vec{\text{#1}}} % arrow\)
\( \newcommand{\vectorB}[1]{\overset { \scriptstyle \rightharpoonup} {\mathbf{#1}} } \)
\( \newcommand{\vectorC}[1]{\textbf{#1}} \)
\( \newcommand{\vectorD}[1]{\overrightarrow{#1}} \)
\( \newcommand{\vectorDt}[1]{\overrightarrow{\text{#1}}} \)
\( \newcommand{\vectE}[1]{\overset{-\!-\!\rightharpoonup}{\vphantom{a}\smash{\mathbf {#1}}}} \)
\( \newcommand{\vecs}[1]{\overset { \scriptstyle \rightharpoonup} {\mathbf{#1}} } \)
\( \newcommand{\vecd}[1]{\overset{-\!-\!\rightharpoonup}{\vphantom{a}\smash {#1}}} \)
\(\newcommand{\avec}{\mathbf a}\) \(\newcommand{\bvec}{\mathbf b}\) \(\newcommand{\cvec}{\mathbf c}\) \(\newcommand{\dvec}{\mathbf d}\) \(\newcommand{\dtil}{\widetilde{\mathbf d}}\) \(\newcommand{\evec}{\mathbf e}\) \(\newcommand{\fvec}{\mathbf f}\) \(\newcommand{\nvec}{\mathbf n}\) \(\newcommand{\pvec}{\mathbf p}\) \(\newcommand{\qvec}{\mathbf q}\) \(\newcommand{\svec}{\mathbf s}\) \(\newcommand{\tvec}{\mathbf t}\) \(\newcommand{\uvec}{\mathbf u}\) \(\newcommand{\vvec}{\mathbf v}\) \(\newcommand{\wvec}{\mathbf w}\) \(\newcommand{\xvec}{\mathbf x}\) \(\newcommand{\yvec}{\mathbf y}\) \(\newcommand{\zvec}{\mathbf z}\) \(\newcommand{\rvec}{\mathbf r}\) \(\newcommand{\mvec}{\mathbf m}\) \(\newcommand{\zerovec}{\mathbf 0}\) \(\newcommand{\onevec}{\mathbf 1}\) \(\newcommand{\real}{\mathbb R}\) \(\newcommand{\twovec}[2]{\left[\begin{array}{r}#1 \\ #2 \end{array}\right]}\) \(\newcommand{\ctwovec}[2]{\left[\begin{array}{c}#1 \\ #2 \end{array}\right]}\) \(\newcommand{\threevec}[3]{\left[\begin{array}{r}#1 \\ #2 \\ #3 \end{array}\right]}\) \(\newcommand{\cthreevec}[3]{\left[\begin{array}{c}#1 \\ #2 \\ #3 \end{array}\right]}\) \(\newcommand{\fourvec}[4]{\left[\begin{array}{r}#1 \\ #2 \\ #3 \\ #4 \end{array}\right]}\) \(\newcommand{\cfourvec}[4]{\left[\begin{array}{c}#1 \\ #2 \\ #3 \\ #4 \end{array}\right]}\) \(\newcommand{\fivevec}[5]{\left[\begin{array}{r}#1 \\ #2 \\ #3 \\ #4 \\ #5 \\ \end{array}\right]}\) \(\newcommand{\cfivevec}[5]{\left[\begin{array}{c}#1 \\ #2 \\ #3 \\ #4 \\ #5 \\ \end{array}\right]}\) \(\newcommand{\mattwo}[4]{\left[\begin{array}{rr}#1 \amp #2 \\ #3 \amp #4 \\ \end{array}\right]}\) \(\newcommand{\laspan}[1]{\text{Span}\{#1\}}\) \(\newcommand{\bcal}{\cal B}\) \(\newcommand{\ccal}{\cal C}\) \(\newcommand{\scal}{\cal S}\) \(\newcommand{\wcal}{\cal W}\) \(\newcommand{\ecal}{\cal E}\) \(\newcommand{\coords}[2]{\left\{#1\right\}_{#2}}\) \(\newcommand{\gray}[1]{\color{gray}{#1}}\) \(\newcommand{\lgray}[1]{\color{lightgray}{#1}}\) \(\newcommand{\rank}{\operatorname{rank}}\) \(\newcommand{\row}{\text{Row}}\) \(\newcommand{\col}{\text{Col}}\) \(\renewcommand{\row}{\text{Row}}\) \(\newcommand{\nul}{\text{Nul}}\) \(\newcommand{\var}{\text{Var}}\) \(\newcommand{\corr}{\text{corr}}\) \(\newcommand{\len}[1]{\left|#1\right|}\) \(\newcommand{\bbar}{\overline{\bvec}}\) \(\newcommand{\bhat}{\widehat{\bvec}}\) \(\newcommand{\bperp}{\bvec^\perp}\) \(\newcommand{\xhat}{\widehat{\xvec}}\) \(\newcommand{\vhat}{\widehat{\vvec}}\) \(\newcommand{\uhat}{\widehat{\uvec}}\) \(\newcommand{\what}{\widehat{\wvec}}\) \(\newcommand{\Sighat}{\widehat{\Sigma}}\) \(\newcommand{\lt}{<}\) \(\newcommand{\gt}{>}\) \(\newcommand{\amp}{&}\) \(\definecolor{fillinmathshade}{gray}{0.9}\)Osmosis is the diffusion of water across membranes from low to high solute concentrations, an essential cellular activity. It allows cells to use water to maintain cellular integrity or to adapt to changes in the solute composition in the extracellular environment. Osmosis relies on the transport of water by aquaporins. The passive diffusion of water molecules can be demonstrated with artificial (e.g., dialysis) membranes. If solute concentrations are higher on one side of the membrane, free water will cross the membrane “trying” to equalize the solute concentrations on both sides of the membrane.
In effect, water movement is away from the side of a membrane where the free water concentration is higher (i.e., where the concentration of solute is lower) and goes to the side where the concentration of free water is lower (i.e., where the concentration of solute is higher).
What do you think is meant by the term free water here?
17.3.1 Osmosis in Plant and Animal Cells
We could present this section in the context of free-water concentrations, but we will do so in the more familiar terms of solute concentrations. Osmosis affects plant and animal cells according to the same principles, but with different effects. The effects of different experimental solute concentrations on animal cells are illustrated in Figure 17.4.

If the solute concentration inside and outside the cell is the same, there is no net water movement into or out of the cells. The extracellular medium and cytosol are said to be isotonic to each other. When water diffuses into the cells from a low-solute medium, the medium is said to by hypotonic to (less concentrated than) the cytosol. In this case, movement of water into a cell lowers the cytosol-solute concentration. Animal cells swell and burst in a hypotonic solution. Animal cells in hypertonic solutions (with higher solute concentrations than the cytosol) shrivel up as water leaves the cell. From this brief description, you should conclude that water crosses from the hypotonic to the hypertonic side of a membrane. As with animal cells, exposure of plant cells to hypotonic or hypertonic solutions causes the same directional water movements, but with some key differences due to their cell walls. Figure 17.5 (below) shows the effects of different solutions on plant cells.
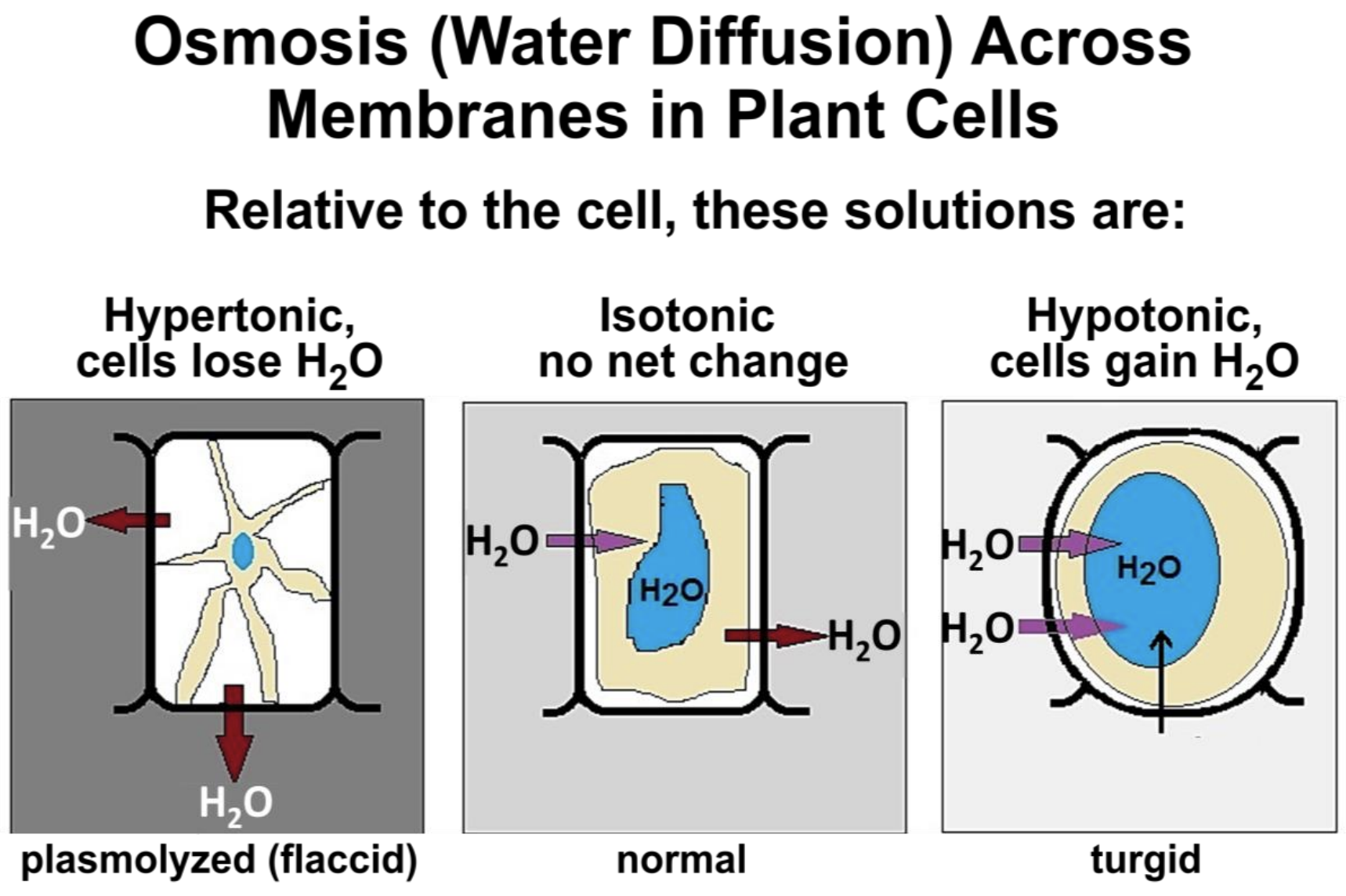
In hypotonic solutions, water enters plant cells, moving into the cytosol and then into water vacuoles called tonoplasts. This results in higher osmotic pressure (water pressure) in the tonoplasts. The expanding tonoplast creates turgor pressure, compressing the cytosol against the cell wall. Rather than bursting, the cells and therefore the plant tissues stiffen and become turgid. Since water cannot enter plant cells indefinitely, water stops entering the cells when the osmotic pressure outside the cells and the turgor pressure inside the cells are at equilibrium. You’ve would have seen this phenomenon if you ever overwatered houseplants. Turgor pressure stiffens the leaves and stems that then become brittle and easily snapped or broken.
In hypertonic medium, plant cells (like animal cells) lose water. The resulting shrinkage of the plasma membrane away from the cell walls is called plasmolysis, in which bits of plasma membrane remain tightly attached to the plant cell wall at several points. You may have seen under-watered plants with floppy or droopy stems and leaves. These have become flaccid due to loss of water and thus the loss of turgor pressure needed to keep leaves and stems upright. Formally, osmotic or turgor pressure is defined as the force per unit area (i.e., pressure) required to prevent the passage of water across a semipermeable membrane from a hypotonic to a hypertonic solution.
17.3.2 Osmosis in Plant Life
While individual plant cells respond to changes in solute concentrations, these changes are rapidly communicated to adjacent cells through plasmodesmata. These structures connect the plasma membranes of adjacent cells through their cell walls, allowing rapid, direct sharing of physical and chemical information. A plasmodesma is illustrated in Figure 17.6.
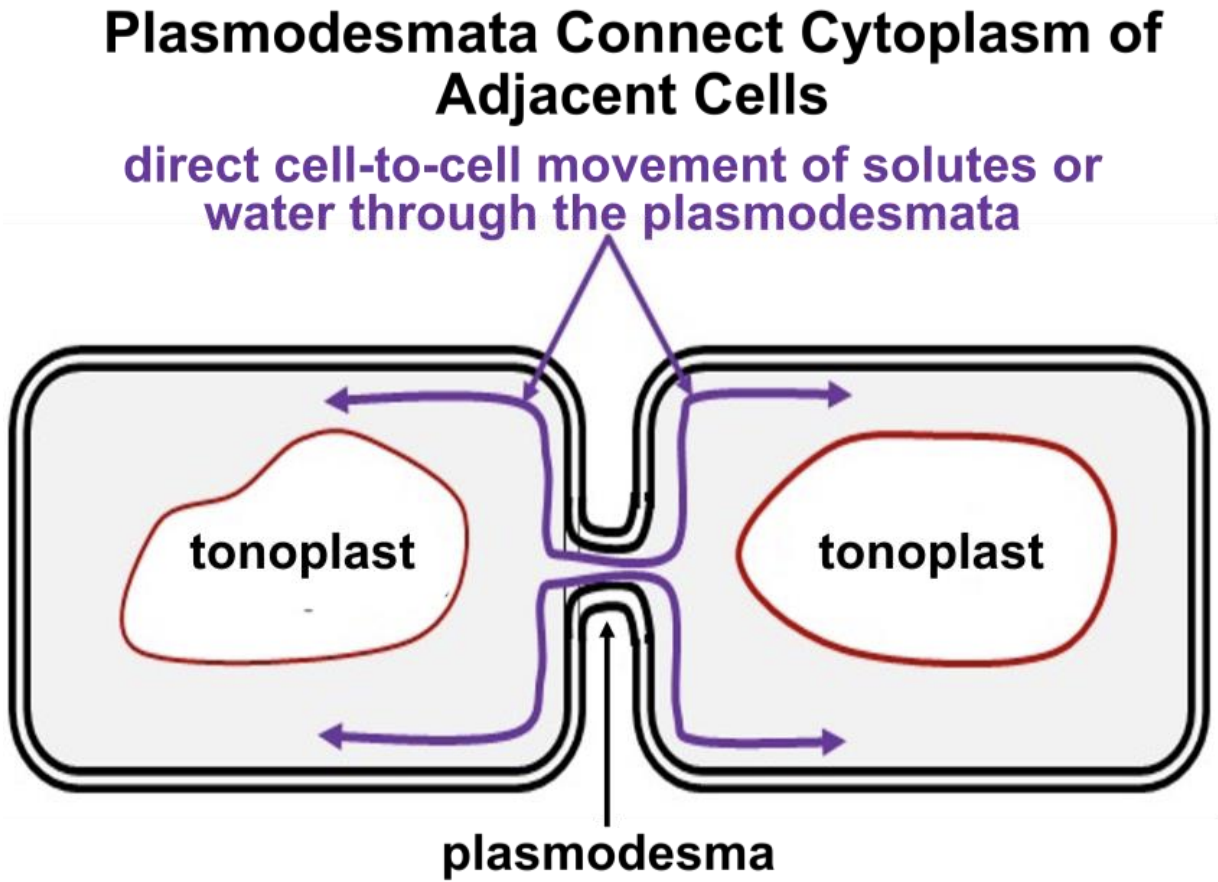
In this way, the effects on osmotic pressure in a few cells—effects created by changes in water availability—are transmitted to adjacent cells, affecting turgor pressure in those cells and, ultimately, in plant tissues. Finally, plant life depends on water! Recall that plant cells require a continual supply of water for use in photosynthesis, to provide hydrogen to reduce CO2 to glucose. Photosynthesis, as well as the loss of excess water from plant tissues (especially leaves) by transpiration, lowers cellular osmotic pressure. As water moves up from the roots to replace water used and lost by leaf cells, the osmotic pressure drops in the fine root-hair cells (with their high surface area). This draws water into the root cells by osmosis. Thus, osmotic pressure is the main force driving water into plants and, defying gravity, moving it up from the roots to the rest of the plant.
17.3.3 Osmosis in Animal Life
Changes in osmotic environment can stress or kill an organism. For example, freshwater organisms (protozoa or fish) placed in sea water will die. Likewise, salt-water fish placed in freshwater. But cells and organisms can osmoregulate (i.e., control the osmotic pressure in their cells), at least to a point. For example, as a paramecium takes on water, a contractile vacuole expels excess water to prevent the cell from bursting (Figure 17.7, below).

Water constantly enters these freshwater protists because the solute concentration in the cytosol is always higher than the fresh water they live in. To cope with a constant uptake of water, their contractile vacuoles collect excess water and then contract to expel the water. At a high energy cost, paramecia constantly pump water out of the cell to maintain water balance (i.e., correct osmotic pressure).
Another protist strategy to cope with changes in environmental solute concentrations (e.g., salinity) is to pump salts (or suitable salt-solute substitutes into or out of the cell, as needed. (For some details, see Protist Osmoregulation Genes from Bacteria?.)
Larger organisms, like freshwater fish, cope with their hypotonic environment by urinating a lot! At the other end of the spectrum, salt-water fish cope with the high solute concentration of solutes (salts) in their environment by excreting excess salt. Salmon spend time in seawater growing to maturity and later swim upstream in fresh water to spawn. You can imagine how salmon and similar organisms have to osmoregulate to adapt to their changing, very different environments. In this case, osmoregulation begins when hormonal changes respond to changes in living circumstance and dictate a compensatory response.
17.3.4 Summing Up Osmosis
Osmosis is the movement of water across membranes to the side where solutes are at a higher concentration. At the same time, solutes diffuse across membranes, moving into or out of cells toward the side where they are at lower concentration, either passively or with the help of transporter proteins. We’ve evolved different facilitated diffusion proteins specific for the cross membrane transport of different solutes. And finally, most water crosses membranes by facilitated diffusion through aquaporin proteins that serve as pores in cellular membranes.