3.4.6: Kingdom Animalia - Adaptations
- Page ID
- 108077
\( \newcommand{\vecs}[1]{\overset { \scriptstyle \rightharpoonup} {\mathbf{#1}} } \)
\( \newcommand{\vecd}[1]{\overset{-\!-\!\rightharpoonup}{\vphantom{a}\smash {#1}}} \)
\( \newcommand{\id}{\mathrm{id}}\) \( \newcommand{\Span}{\mathrm{span}}\)
( \newcommand{\kernel}{\mathrm{null}\,}\) \( \newcommand{\range}{\mathrm{range}\,}\)
\( \newcommand{\RealPart}{\mathrm{Re}}\) \( \newcommand{\ImaginaryPart}{\mathrm{Im}}\)
\( \newcommand{\Argument}{\mathrm{Arg}}\) \( \newcommand{\norm}[1]{\| #1 \|}\)
\( \newcommand{\inner}[2]{\langle #1, #2 \rangle}\)
\( \newcommand{\Span}{\mathrm{span}}\)
\( \newcommand{\id}{\mathrm{id}}\)
\( \newcommand{\Span}{\mathrm{span}}\)
\( \newcommand{\kernel}{\mathrm{null}\,}\)
\( \newcommand{\range}{\mathrm{range}\,}\)
\( \newcommand{\RealPart}{\mathrm{Re}}\)
\( \newcommand{\ImaginaryPart}{\mathrm{Im}}\)
\( \newcommand{\Argument}{\mathrm{Arg}}\)
\( \newcommand{\norm}[1]{\| #1 \|}\)
\( \newcommand{\inner}[2]{\langle #1, #2 \rangle}\)
\( \newcommand{\Span}{\mathrm{span}}\) \( \newcommand{\AA}{\unicode[.8,0]{x212B}}\)
\( \newcommand{\vectorA}[1]{\vec{#1}} % arrow\)
\( \newcommand{\vectorAt}[1]{\vec{\text{#1}}} % arrow\)
\( \newcommand{\vectorB}[1]{\overset { \scriptstyle \rightharpoonup} {\mathbf{#1}} } \)
\( \newcommand{\vectorC}[1]{\textbf{#1}} \)
\( \newcommand{\vectorD}[1]{\overrightarrow{#1}} \)
\( \newcommand{\vectorDt}[1]{\overrightarrow{\text{#1}}} \)
\( \newcommand{\vectE}[1]{\overset{-\!-\!\rightharpoonup}{\vphantom{a}\smash{\mathbf {#1}}}} \)
\( \newcommand{\vecs}[1]{\overset { \scriptstyle \rightharpoonup} {\mathbf{#1}} } \)
\( \newcommand{\vecd}[1]{\overset{-\!-\!\rightharpoonup}{\vphantom{a}\smash {#1}}} \)
Unit 3.4.6 - Kingdom Animalia
- Please read and watch the following Learning Resources
- Reading the material for understanding, and taking notes during videos, will take approximately 90 minutes.
- Optional Activities are embedded.
- Bolded terms are located at the end of the unit in the Glossary. There is also a Unit Summary at the end of the Unit.
- To navigate to Unit 3.4.7, use the Contents menu at the top of the page OR the right arrow on the side of the page.
- If on a mobile device, use the Contents menu at the top of the page OR the links at the bottom of the page.
- Explain the processes of animal reproduction and embryonic development
- Describe the roles that Hox genes play in development
- Explain the differences in animal body plans that support basic animal classification
- Compare and contrast the embryonic development of protostomes and deuterostomes
Introduction to Animal Reproduction and Development
Most animals are diploid organisms, meaning that their body (somatic) cells are diploid and haploid reproductive (gamete) cells are produced through meiosis. Some exceptions exist: For example, in bees, wasps, and ants, the male is haploid because it develops from unfertilized eggs. Most animals are able to undergo sexual reproduction. This fact distinguishes animals from fungi, protists, and bacteria, where asexual reproduction is common or exclusive. During sexual reproduction, the haploid gametes of the male and female individuals of a species combine in a process called fertilization. Typically, the small, motile male sperm fertilizes the much larger, sessile female egg. This process produces a diploid fertilized egg called a zygote. After fertilization, a series of developmental stages occur during which primary germ layers are established and reorganize to form an embryo. During this process, animal tissues begin to specialize and organize into organs and organ systems, determining their future morphology and physiology. The series of developmental stages of the embryo remains largely the same for most members of the animal kingdom.
Some animal groups are also able to reproduce asexually—including cnidarians, roundworms, echinoderms, as well as some insects, reptiles, and fish. The most common forms of asexual reproduction for stationary aquatic animals include budding and fragmentation, where part of a parent individual can separate and grow into a new individual. In contrast, a form of asexual reproduction found in certain insects and vertebrates is called parthenogenesis (or “virgin beginning”), where unfertilized eggs can develop into new offspring. This type of parthenogenesis is called haplodiploidy. These types of asexual reproduction produce genetically identical offspring, which is disadvantageous from the perspective of evolutionary adaptability because of the potential buildup of deleterious mutations. However, for animals that are limited in their capacity to attract mates, asexual reproduction can ensure genetic propagation.
In this 7-minute time lapse video of embryogenic development, you will see a fertilized cell move through cell division and rearrangement of tissues to create a multicellular salamander.
Question after watching: What do you notice about how a one-celled organism becomes a whole animal? What appears to be stages of development?
Watch the following 4-minute video to see how human embryonic development (after the blastula and gastrula stages of development) reflects evolution.
Question after watching: This video shows an animation of human development from a one-celled organism. Compare it to the time lapse video of a salamander's development taken with a microscope. At 0:38 seconds into this video, the animation shows the development of the notochord. When does this happen in the time lapse video of the salamander development? What does this become once the organism is fully formed?
The Role of Homeobox (Hox) Genes in Animal Development
Since the early 19th century, scientists have observed that many animals, from the very simple to the complex, shared similar embryonic morphology and development. A human embryo and a frog embryo, at a certain stage of embryonic development, look remarkably alike. For a long time, scientists did not understand why so many animal species looked similar during embryonic development but were very different in adults. They wondered what dictated the developmental direction that a fly, mouse, frog, or human embryo would take. Near the end of the 20th century, a particular class of genes was discovered that had this very job. These genes that determine animal structure are called “homeotic genes,” and they contain DNA sequences called homeoboxes. The animal genes containing homeobox sequences are specifically referred to as Hox genes. This family of genes is responsible for determining the general body plan, such as the number of body segments of an animal, the number and placement of appendages, and animal head-tail directionality. The first Hox genes to be sequenced were those from the fruit fly (Drosophila melanogaster). A single Hox mutation in the fruit fly can result in an extra pair of wings or even appendages growing from the “wrong” body part.
While there are a great many genes that play roles in the morphological development of an animal, what makes Hox genes so powerful is that they serve as master control genes that can turn on or off large numbers of other genes. Hox genes are well conserved in the animal kingdom. That is, the genetic sequences of Hox genes and their positions on chromosomes are remarkably similar across most animals because they were strongly selected for in a common ancestor to nearly all and are not selected against today (Figure \(\PageIndex{1}\)). One of the contributions to increased animal body complexity is that Hox genes have undergone at least two duplication events during animal evolution (humans have undergone four duplication events), with the additional genes allowing for more complex body types to evolve.

Figure \(\PageIndex{1}\): If a Hox 13 gene in a mouse was replaced with a Hox 1 gene, how might this alter animal development?
- Answer
-
The animal might develop two heads and no tail.
Classifying Animalia
Complex Tissue Structure
As multicellular organisms, animals differ from plants and fungi in several ways. Animal cells do not have cell walls, their cells may be embedded in an extracellular matrix (such as bone, skin, or connective tissue), and their cells have unique structures for intercellular communication (such as gap junctions). In addition, animals possess unique tissues, absent in fungi and plants, which allow coordination (nerve tissue) of motility (muscle tissue). Animals are also characterized by specialized connective tissues that provide structural support for cells and organs. This connective tissue constitutes the extracellular surroundings of cells and is made up of organic and inorganic materials. The complex bodies and activities of vertebrates demand such supportive tissues.
The different types of tissues in the Eumetazoa are responsible for carrying out specific functions for the organism. This differentiation and specialization of tissues is part of what allows for such incredible animal diversity. For example, the evolution of nerve tissues and muscle tissues has resulted in animals’ unique ability to rapidly sense and respond to changes in their environment. This allows animals to survive in environments where they must compete with other species to meet their nutritional demands.
Body Symmetry
At a very basic level of classification, true animals can be largely divided into three groups based on the type of symmetry of their body plan: radially symmetrical, bilaterally symmetrical, and asymmetrical. Asymmetry is a unique feature of Parazoa (Figure \(\PageIndex{2}\)). Only a few animal groups display radial symmetry. All types of symmetry are well suited to meet the unique demands of a particular animal’s lifestyle.
Radial symmetry is the arrangement of body parts around a central axis, as is seen in a drinking glass or pie. It results in animals having top and bottom surfaces but no left and right sides, or front or back. The two halves of a radially symmetrical animal may be described as the side with a mouth or “oral side,” and the side without a mouth (the “aboral side”). This form of symmetry marks the body plans of animals in the phyla Ctenophora and Cnidaria, including jellyfish and adult sea anemones (Figure \(\PageIndex{2}\)). Radial symmetry equips these sea creatures (which may be sedentary or only capable of slow movement or floating) to experience the environment equally from all directions.

Bilateral symmetry involves the division of the animal through a sagittal plane, resulting in two mirror images, right and left halves, such as those of a butterfly (Figure \(\PageIndex{3}\)), crab, or human body. Animals with bilateral symmetry have a “head” and “tail” (anterior vs. posterior), front and back (dorsal vs. ventral), and right and left sides. Most animals are bilaterally symmetrical. The evolution of bilateral symmetry that allowed for the formation of anterior and posterior (head and tail) ends promoted a phenomenon called cephalization, which refers to the collection of an organized nervous system at the animal’s anterior end. In contrast to radial symmetry, which is best suited for stationary or limited-motion lifestyles, bilateral symmetry allows for streamlined and directional motion. In evolutionary terms, this simple form of symmetry promoted active mobility and increased the sophistication of resource-seeking and predator-prey relationships.
Animals in the phylum Echinodermata (such as sea stars, sand dollars, and sea urchins) display radial symmetry as adults, but their larval stages exhibit bilateral symmetry. This is termed secondary radial symmetry. They are believed to have evolved from bilaterally symmetrical animals; thus, they are classified as bilaterally symmetrical.

In this quick 1-minute video, you will review the three types of body symmetry.
Question after watching: What might be an advantage of bilateral symmetry? Why did it evolve? The video mentions that the left and right parts of our bodies are not identical. What are some examples of ways in which our two sides are not symmetrical?
Embryological Development
Most animal species undergo a separation of tissues into germ layers during embryonic development. Germ layers are formed during gastrulation and they are predetermined to develop into the animal’s specialized tissues and organs. Animals develop either two or three embryonic germ layers (Figure \(\PageIndex{4}\)). The animals that display radial symmetry develop two germ layers, an inner layer (endoderm) and an outer layer (ectoderm). These animals are called diploblasts. Diploblasts have a non-living layer between the endoderm and ectoderm. More complex animals (those with bilateral symmetry) develop three tissue layers: an inner layer (endoderm), an outer layer (ectoderm), and a middle layer (mesoderm). Animals with three tissue layers are called triploblasts.
Each of the three germ layers is programmed to give rise to particular body tissues and organs. In humans, the endoderm gives rise to the lining of the digestive tract (including the stomach, intestines, liver, and pancreas), as well as to the lining of the trachea, bronchi, and lungs of the respiratory tract, along with a few other structures. The ectoderm develops into the outer epithelial covering of the body surface, the central nervous system, and a few other structures. The mesoderm is the third germ layer; it forms between the endoderm and ectoderm in triploblasts. This germ layer gives rise to all muscle tissues (including the cardiac tissues and muscles of the intestines), connective tissues such as the skeleton and blood cells, and most other visceral organs such as the kidneys and the spleen.
This 11.5-minute video provides an overview of human development from a fertilized egg until birth and highlights how tissues rearrange to create tubes as a strategy to form the body plan.
Question after watching: How is your body plan really just a tube? How does a tube come to be during animal development?
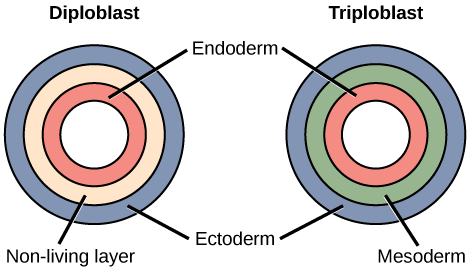
Figure \(\PageIndex{5}\): Which of the following statements about diploblasts and triploblasts is false?
- Animals that display radial symmetry are diploblasts.
- Animals that display bilateral symmetry are triploblasts.
- The endoderm gives rise to the lining of the digestive tract and the respiratory tract.
- The mesoderm gives rise to the central nervous system.
- Answer
-
D. The mesoderm gives rise to the central nervous system.
Coelom: Presence or Absence
Further subdivision of animals with three germ layers (triploblasts) results in the separation of animals that may develop an internal body cavity derived from mesoderm, called a coelom, and those that do not. This epithelial cell-lined coelomic cavity represents a space, usually filled with fluid, which lies between the visceral organs and the body wall. It houses many organs such as the digestive system, kidneys, reproductive organs, and heart, and contains the circulatory system. In some animals, such as mammals, the part of the coelom called the pleural cavity provides space for the lungs to expand during breathing. The evolution of the coelom is associated with many functional advantages. Primarily, the coelom provides cushioning and shock absorption for the major organ systems. Organs housed within the coelom can grow and move freely, which promotes optimal organ development and placement. The coelom also provides space for the diffusion of gases and nutrients, as well as body flexibility, promoting improved animal motility.
Triploblasts that do not develop a coelom are called acoelomates, and their mesoderm region is completely filled with tissue, although they do still have a gut cavity. Examples of acoelomates include animals in the phylum Platyhelminthes, also known as flatworms. Animals with a true coelom are called eucoelomates (or coelomates) (Figure \(\PageIndex{5}\)). A true coelom arises entirely within the mesoderm germ layer and is lined by an epithelial membrane. Annelids, mollusks, arthropods, echinoderms, and chordates are all eucoelomates. A third group of triploblasts has a slightly different coelom derived partly from mesoderm and partly from endoderm, which is found between the two layers. Although still functional, these are considered false coeloms, and those animals are called pseudocoelomates. The phylum Nematoda (roundworms) is an example of a pseudocoelomate.

Embryonic Development of the Mouth
Finally, true coelomates can be further characterized based on certain features of their early embryological development. Bilaterally symmetrical, tribloblastic eucoelomates can be further divided into two groups based on differences in their early embryonic development. Protostomes include arthropods, mollusks, and annelids. Deuterostomes include more complex animals such as chordates but also some simple animals such as echinoderms. These two groups are separated based on which opening of the digestive cavity develops first: mouth or anus. The word protostome comes from the Greek word meaning “mouth first,” and deuterostome originates from the word meaning “mouth second” (in this case, the anus develops first).
The mouth or anus develops from a structure called the blastopore (Figure \(\PageIndex{6}\)). The blastopore is the indentation formed during the initial stages of gastrulation. In later stages, a second opening forms, and these two openings will eventually give rise to the mouth and anus. It has long been believed that the blastopore develops into the mouth of protostomes, with the second opening developing into the anus; the opposite is true for deuterostomes. Recent evidence has challenged this view of the development of the blastopore of protostomes, however, and the theory remains under debate.
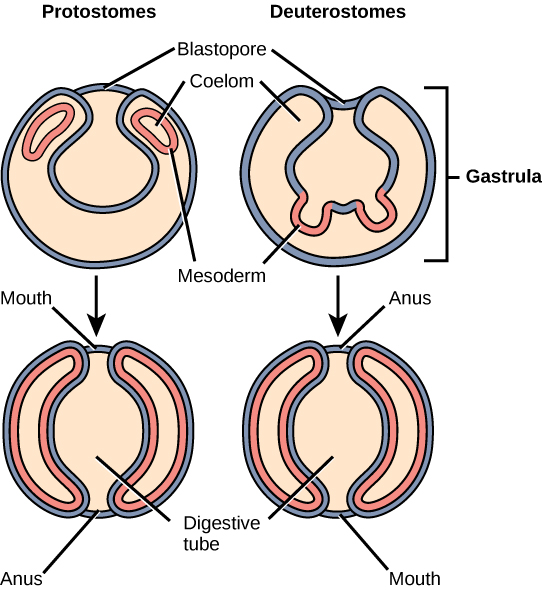
Which of the following is not a feature common to most animals?
- development into a fixed body plan
- asexual reproduction
- specialized tissues
- heterotrophic nutrient sourcing
- Answer
-
B. asexual reproduction
Which of the following is not possible?
- radially symmetrical diploblast
- diploblastic eucoelomate
- protostomic coelomate
- bilaterally symmetrical deuterostome
- Answer
-
B. diploblastic eucoelomate
'Namgis Treaty researcher Diana Jacobson reminds us of traditional knowledge embedded in story:
There’s one woman who is a twin and she turns into a dog salmon. She told all about the fish carcasses that they had to be thrown back into the river. This way our old people knew that the carcasses fed the future fry by coming up when they emerged. This knowledge was passed on with the stories so anytime they cut up the fish and heads that they used, it went back into the river and it rotted …and it fed the fish. (personal interview, July 16, 2008)
Despite fishing since time immemorial, Indigenous fisheries management in British Columbia was replaced with state regulation (Indigenous Foundations, 2009):
When the first salmon canneries appeared on the BC coast in the 1870s, Indigenous peoples had already built flourishing economies based on salmon. The one-way spawning migrations of adult salmon to the stretch of river where they were born has always brought a crucial source of energy and nutrients from the ocean to freshwater and terrestrial environments, allowing people to preserve vast quantities of fish for winter use, and for trade with other Indigenous groups. Salmon were received by First Nations peoples as gift-bearing relatives, and were treated with great respect. Nick Claxton describes his community’s traditional reef-net fishery as a successful collaboration between the fish and the people:
The WSÁNEC people successfully governed their traditional fisheries for thousands of years, prior to contact. This was not just because there were laws and rules in place, and that everybody followed them, but there was also a different way of thinking about fish and fishing, which included a profound respect. At the end of the net, a ring of willow was woven into the net, which allowed some salmon to escape. This is more than just a simple act of conservation (the main priority and narrow vision of the Department of Fisheries and Oceans). It represents a profound respect for salmon. It was believed that the runs of salmon were lineages, and if some were allowed to return to their home rivers, then those lineages would always continue. The WSÁNEC people believe that all living things were once people, and they are respected as such. The salmon are our relatives. Out of respect, when the first large sockeye was caught, a First Salmon Ceremony was conducted. This was the WSÁNEC way to greet and welcome the king of all salmon. The celebration would likely last up to ten days. Taking time to celebrate allowed for a major portion of the salmon stocks to return to their rivers to spawn, and to sustain those lineages or stocks. (2008)
As lineages or stocks, salmon populations are enormously diverse, with many different life-history adaptations, such as the length of time spent in freshwater and in the ocean, the timing of spawning, and age and size at maturity. In addition, since fat content decreases with migration distance, processing techniques, such as drying and smoking, needed to be closely adapted to individual runs. Local ecology and culture worked together to produce highly specialized salmon food products for food and trade. Wind-drying of salmon in the Fraser Canyon, for example, depended on the temperature and humidity conditions created by this upriver canyon environment. In early July, when hot, dry winds blew into the canyon, salmon were fished from particular, family-owned spots, and then hung on giant racks perched on rock bluffs, where they could be dried before the arrival of blowflies and wasps (Carlson, 2001). Despite government intervention, and changes in technology to fishing by boat with gill nets, the practice of wind-drying continues to this day among some Stó:lō families, and wind-drying is consciously regarded as a traditional practice in need of protection (Butler, 1998).
Source: Adapted from Isaac (2016).
References
Butler, C. (1998). Regulating tradition: Sto:lo wind drying and Aboriginal rights [Unpublished master's thesis]. University of British Columbia.
Carlson, K. T. (2001). History wars: Considering contemporary fishing site disputes. In K. T. Carson (Ed.), A Sto:lo Coast Salish historical atlas (p. 58). Douglas & McIntyre.
Claxton, N. X. (2008). ISTÁ SĆIÁNEW, ISTÁ SXOLE: ‘To fish as formerly:’ The Douglas Treaties and the WSÁNEĆ reef-net fisheries. In L. Simpson (Ed.), Lighting the eighth fire: The liberation, resurgence, and protection of Indigenous nations (pp. 54–55). Arbeiter Ring.
Indigenous Foundations, First Nations Studies Program. (2009). Aboriginal fisheries in British Columbia. First Nations & Indigenous Studies, University of British Columbia. https://indigenousfoundations.arts.u...itish_columbia
Isaac, W. I. (2016). Storytelling is our textbook and curriculum guide. In G. Snively & W. L. Williams (Eds.), Knowing home: Braiding Indigenous science with Western science, book 1. University of Victoria. https://pressbooks.bccampus.ca/knowi...ter/chapter-14
Thumbnail: Capybara (Hydrochoerus hydrochaeris). (CC BY-SA 4.0; Charlesjsharp)