1.3: Microscopy
\( \newcommand{\vecs}[1]{\overset { \scriptstyle \rightharpoonup} {\mathbf{#1}} } \)
\( \newcommand{\vecd}[1]{\overset{-\!-\!\rightharpoonup}{\vphantom{a}\smash {#1}}} \)
\( \newcommand{\id}{\mathrm{id}}\) \( \newcommand{\Span}{\mathrm{span}}\)
( \newcommand{\kernel}{\mathrm{null}\,}\) \( \newcommand{\range}{\mathrm{range}\,}\)
\( \newcommand{\RealPart}{\mathrm{Re}}\) \( \newcommand{\ImaginaryPart}{\mathrm{Im}}\)
\( \newcommand{\Argument}{\mathrm{Arg}}\) \( \newcommand{\norm}[1]{\| #1 \|}\)
\( \newcommand{\inner}[2]{\langle #1, #2 \rangle}\)
\( \newcommand{\Span}{\mathrm{span}}\)
\( \newcommand{\id}{\mathrm{id}}\)
\( \newcommand{\Span}{\mathrm{span}}\)
\( \newcommand{\kernel}{\mathrm{null}\,}\)
\( \newcommand{\range}{\mathrm{range}\,}\)
\( \newcommand{\RealPart}{\mathrm{Re}}\)
\( \newcommand{\ImaginaryPart}{\mathrm{Im}}\)
\( \newcommand{\Argument}{\mathrm{Arg}}\)
\( \newcommand{\norm}[1]{\| #1 \|}\)
\( \newcommand{\inner}[2]{\langle #1, #2 \rangle}\)
\( \newcommand{\Span}{\mathrm{span}}\) \( \newcommand{\AA}{\unicode[.8,0]{x212B}}\)
\( \newcommand{\vectorA}[1]{\vec{#1}} % arrow\)
\( \newcommand{\vectorAt}[1]{\vec{\text{#1}}} % arrow\)
\( \newcommand{\vectorB}[1]{\overset { \scriptstyle \rightharpoonup} {\mathbf{#1}} } \)
\( \newcommand{\vectorC}[1]{\textbf{#1}} \)
\( \newcommand{\vectorD}[1]{\overrightarrow{#1}} \)
\( \newcommand{\vectorDt}[1]{\overrightarrow{\text{#1}}} \)
\( \newcommand{\vectE}[1]{\overset{-\!-\!\rightharpoonup}{\vphantom{a}\smash{\mathbf {#1}}}} \)
\( \newcommand{\vecs}[1]{\overset { \scriptstyle \rightharpoonup} {\mathbf{#1}} } \)
\( \newcommand{\vecd}[1]{\overset{-\!-\!\rightharpoonup}{\vphantom{a}\smash {#1}}} \)
\(\newcommand{\avec}{\mathbf a}\) \(\newcommand{\bvec}{\mathbf b}\) \(\newcommand{\cvec}{\mathbf c}\) \(\newcommand{\dvec}{\mathbf d}\) \(\newcommand{\dtil}{\widetilde{\mathbf d}}\) \(\newcommand{\evec}{\mathbf e}\) \(\newcommand{\fvec}{\mathbf f}\) \(\newcommand{\nvec}{\mathbf n}\) \(\newcommand{\pvec}{\mathbf p}\) \(\newcommand{\qvec}{\mathbf q}\) \(\newcommand{\svec}{\mathbf s}\) \(\newcommand{\tvec}{\mathbf t}\) \(\newcommand{\uvec}{\mathbf u}\) \(\newcommand{\vvec}{\mathbf v}\) \(\newcommand{\wvec}{\mathbf w}\) \(\newcommand{\xvec}{\mathbf x}\) \(\newcommand{\yvec}{\mathbf y}\) \(\newcommand{\zvec}{\mathbf z}\) \(\newcommand{\rvec}{\mathbf r}\) \(\newcommand{\mvec}{\mathbf m}\) \(\newcommand{\zerovec}{\mathbf 0}\) \(\newcommand{\onevec}{\mathbf 1}\) \(\newcommand{\real}{\mathbb R}\) \(\newcommand{\twovec}[2]{\left[\begin{array}{r}#1 \\ #2 \end{array}\right]}\) \(\newcommand{\ctwovec}[2]{\left[\begin{array}{c}#1 \\ #2 \end{array}\right]}\) \(\newcommand{\threevec}[3]{\left[\begin{array}{r}#1 \\ #2 \\ #3 \end{array}\right]}\) \(\newcommand{\cthreevec}[3]{\left[\begin{array}{c}#1 \\ #2 \\ #3 \end{array}\right]}\) \(\newcommand{\fourvec}[4]{\left[\begin{array}{r}#1 \\ #2 \\ #3 \\ #4 \end{array}\right]}\) \(\newcommand{\cfourvec}[4]{\left[\begin{array}{c}#1 \\ #2 \\ #3 \\ #4 \end{array}\right]}\) \(\newcommand{\fivevec}[5]{\left[\begin{array}{r}#1 \\ #2 \\ #3 \\ #4 \\ #5 \\ \end{array}\right]}\) \(\newcommand{\cfivevec}[5]{\left[\begin{array}{c}#1 \\ #2 \\ #3 \\ #4 \\ #5 \\ \end{array}\right]}\) \(\newcommand{\mattwo}[4]{\left[\begin{array}{rr}#1 \amp #2 \\ #3 \amp #4 \\ \end{array}\right]}\) \(\newcommand{\laspan}[1]{\text{Span}\{#1\}}\) \(\newcommand{\bcal}{\cal B}\) \(\newcommand{\ccal}{\cal C}\) \(\newcommand{\scal}{\cal S}\) \(\newcommand{\wcal}{\cal W}\) \(\newcommand{\ecal}{\cal E}\) \(\newcommand{\coords}[2]{\left\{#1\right\}_{#2}}\) \(\newcommand{\gray}[1]{\color{gray}{#1}}\) \(\newcommand{\lgray}[1]{\color{lightgray}{#1}}\) \(\newcommand{\rank}{\operatorname{rank}}\) \(\newcommand{\row}{\text{Row}}\) \(\newcommand{\col}{\text{Col}}\) \(\renewcommand{\row}{\text{Row}}\) \(\newcommand{\nul}{\text{Nul}}\) \(\newcommand{\var}{\text{Var}}\) \(\newcommand{\corr}{\text{corr}}\) \(\newcommand{\len}[1]{\left|#1\right|}\) \(\newcommand{\bbar}{\overline{\bvec}}\) \(\newcommand{\bhat}{\widehat{\bvec}}\) \(\newcommand{\bperp}{\bvec^\perp}\) \(\newcommand{\xhat}{\widehat{\xvec}}\) \(\newcommand{\vhat}{\widehat{\vvec}}\) \(\newcommand{\uhat}{\widehat{\uvec}}\) \(\newcommand{\what}{\widehat{\wvec}}\) \(\newcommand{\Sighat}{\widehat{\Sigma}}\) \(\newcommand{\lt}{<}\) \(\newcommand{\gt}{>}\) \(\newcommand{\amp}{&}\) \(\definecolor{fillinmathshade}{gray}{0.9}\)- Tell the importance of using microscopes in microbiology.
- Successfully use the metric system for length including unit conversion calculations.
- Define resolution.
- Define magnification.
- Determine/calculate total magnification for each objective lens.
- Identify the structures of a light microscope.
- Identify the functions of the structures of a light microscope.
- Successfully use and care for a light microscope.
- Differentiate between light microscopy and electron microscopy.
- Differentiate between TEM and SEM and the appropriate uses for each.
Early Microscopy
The first microscope was developed in 1590 by Dutch lens grinders Hans and Zacharias Jansen. In 1667, Robert Hooke described the microscopic appearance of cork and used the term cell to describe the compartments he observed. Anton van Leeuwenhoek was the first person to observe living cells under the microscope in 1675—he described many types of cells, including bacteria. Since then more sophisticated and powerful scopes have been developed that allow for higher magnification and clearer images.
Microscopy is used by scientists and health care professionals for many purposes, including diagnosis of infectious diseases, identification of microorganisms (microscopic organisms) in environmental samples (including food and water), and determination of the effect of pathogenic (disease-causing) microbes on human cells. This exercise will familiarize you with the microscopes we will be using to look at various types of microorganisms throughout the semester.
Metric Units & Microscopy
Unlike typical length measurement units used in the United States (inches, feet, yards, miles), microscopy uses the metric system (nanometers, micrometers, millimeters). Scientific measurements use the metric system. The metric system is also used for measurement in every country in the world except in three countries: Liberia, Myanmar, and The United States. Although these measurements are likely new for you, they are very user-friendly since the metric system uses units that are all related to the meter (the base unit) and are different from each other by powers of 10.
Metric Units for Length
Since microscopes are concerned with the size (length) of structures and the magnification of these structures, we will consider the metric system as it relates to length. The base unit of length in the metric system is the meter (abbreviated as m). One meter is about 3.3 feet long. For units that are larger or smaller than meters, a prefix is added to the beginning of "meter" to indicate the magnitude of difference from the meter unit (base unit):
Unit of Length |
Abbreviation |
Size in relationship to a meter (meter is the base unit) |
---|---|---|
kilometer |
km |
1,000 or 103 |
hectometer |
hm |
100 or 102 |
decameter |
dam |
10 or 101 |
meter (base unit) |
m |
1 or 100 |
decimeter |
dm |
0.1 or 10-1 |
centimeter |
cm |
0.01 or 10-2 |
millimeter |
mm |
0.001 or 10-3 |
micrometer |
µm |
0.000001 or 10-6 |
nanometer |
nm |
0.000000001 or 10-9 |
picometer |
pm |
0.000000000001 or 10-12 |
Sizes of Cells & Microbes
Cells are typically measured using the micrometer (µm) unit, but some subcellular structures may be reported in nanometers (nm) measurements. To give you a sense of cell size, a typical human red blood cell is about eight millionths of a meter or eight micrometers (abbreviated as 8 μm) in diameter; the head of a pin of is about two thousandths of a meter (two mm) in diameter. That means about 250 red blood cells could fit on the head of a pin!
*microbes in this interactive tool include: amoeba proteus, paramecium, baker's yeast, E. coli bacterium, measles virus, hiv, phage, influenza virus, hepatitis virus, and rhinovirus
Converting Metric Length Units
Converting Units to Meters (the Base Unit)
Using the table above that distinguishes units by prefix, to convert unit measurements back to the base unit, simply multiply the number you are converting with the number in the "size relationship to a meter" column. For example:
Using your microscope you measure a cell as 20 µm ("twenty micrometers"). To convert 20 µm to meters, multiply 20 µm by 0.000001:
20 µm x 0.000001 = 0.00002 m
or
20 µm x 10-6 = 0.00002 m
You measure the distance it takes you to get home from school as 19 km ("nineteen kilometers"). To convert 19 km to meters, multiply 19 km by 1,000:
19 km x 1,000 = 19,000 m
or
19 km x 103 = 19,000 m
Converting Meters (the Base Unit) to Other Units
Using the table above that distinguishes units by prefix, to convert the base unit to another unit, simply divide the number you are converting with the number in the "size relationship to a meter" column. For example:
You are told that a certain very large cell is 0.0003 m long. To convert 0.0003 m to micrometers (µm), divide 0.0003 m by 0.000001:
0.0003 m ÷ 0.000001 = 300 µm
or
0.0003 m ÷ 10-6 = 300 µm
You read that running a marathon means running 42,000 m. To convert 42,000 m to kilometers (km), divide 42,000 m by 1,000:
42,000 m ÷ 1,000 = 42 km
or
42,000 m ÷ 103 = 42 km
Light Microscopes vs. Electron Microscopes
Most microscopes used in college biology laboratories are classified as light microscopes (see the figure, part (a) below) and may also be called compound microscopes since they use two lenses whose magnifications compound (multiply). Visible light passes through the specimen and is bent through the lens system to enable the user to see the specimen. Light microscopes are advantageous since they are capable of viewing living organisms. However, since individual cells are generally transparent, their components are not distinguishable unless they are colored with special stains (colored chemicals that makes cells appear to have color such as pink, blue, or purple). Stained cells are dead cells, but staining is an important approach to better see cells and cell structures.
Due to the magnification limits of light microscopes, in order to gain a better understanding of cellular structure and function, scientists typically use electron microscopes that use electrons (subatomic particles) to carry the microscopic images instead of using light particles (photons). Electron microscopes also typically require staining the sample with heavy metals to be able to visualize the sample.
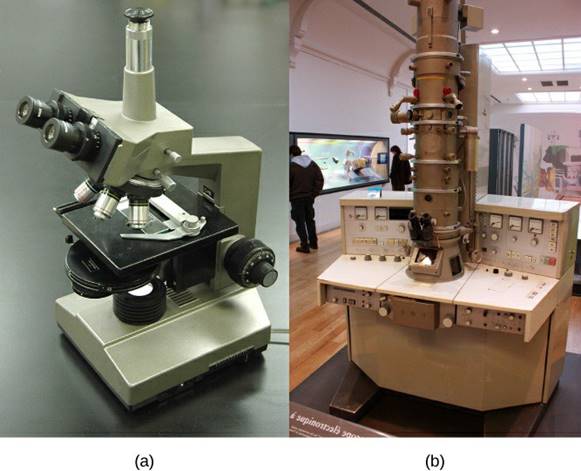
To see the difference in magnification and detail possible when comparing light microscopes and electron microscopes, compare the images of Salmonella (a type of bacteria) in the figure below.
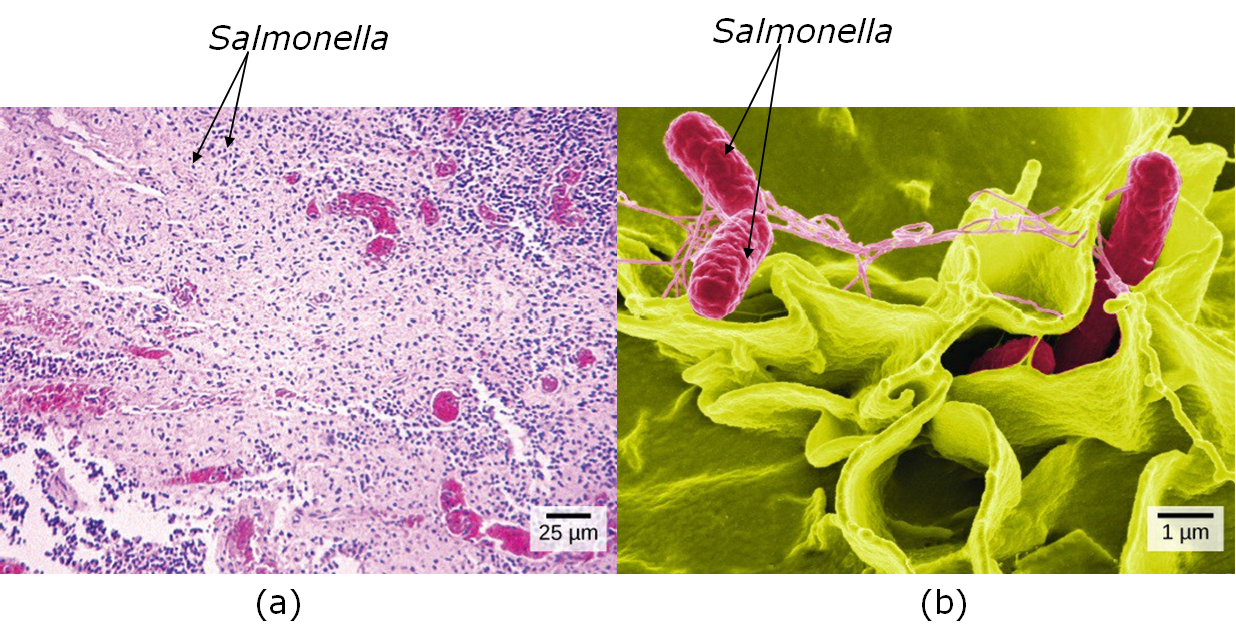
The Light Microscope
What does it mean to be microscopic? Objects are said to be microscopic when they are too small to be seen with the unaided eye—they need to be magnified (enlarged) for the human eye to be able to see them. This includes human cells and many other types of cells that you will be studying in this class. The microscope you will be using uses visible light and two sets of lenses to produce a magnified image. The total magnification will depend on which objective lens you are using—the highest magnification possible on these microscopes is typically 1000X—meaning that objects appear 1000X larger than they actually are.
Resolution vs. Magnification
Magnification refers to the process of making an object appear larger than it is; whereas resolution is the ability to see objects clearly enough to tell two distinct objects apart. Although it is possible to magnify above 1000X, a higher magnification would result in a blurry image. (Think about magnifying a digital photograph beyond the point where you can see the image clearly). This is due to the limitations of visible light (details that are smaller than the wavelength of light used cannot be resolved).
The limit of resolution of the human eye is about 0.1 mm ("zero point one millimeters"), or 100 µm ("one-hundred micrometers"). Objects that are smaller than this cannot be seen clearly without magnification. Since most cells are much smaller than 100 µm, we need to use microscopes to see them.
The limit of resolution of a standard brightfield light microscope, also called the resolving power, is ~0.2 µm, or 200 nm. Biologists typically use microscopes to view all types of cells, including plant cells, animal cells, protozoa, algae, fungi, and bacteria. The nucleus and chloroplasts of eukaryotic cells can also be seen—however smaller organelles and viruses are beyond the limit of resolution of the light microscope.
Resolution is the ability of the lenses to distinguish between two adjacent objects as distinct and separate.
A compound light microscope has a maximum resolution of 0.2 µm, this means it can distinguish between two points ≥ 0.2 µm, any objects closer than 0.2um will be seen as 1 object. Shorter wavelengths of light provide greater resolution. This is why we often have a blue filter over our light source in the microscope, it helps to increase resolution since its wavelength is the shortest in the visible light spectrum. Without resolution, no matter how much the image is magnified, the amount of observable detail is fixed, and regardless of how much you increase the size of the image, no more detail can be seen. At this point, you will have reached the limit of resolution or the resolving power of the lens. This property of the lens is fixed by the design and construction of the lens. To change the resolution, a different lens is often the only answer.
Parts of a Light Microscope
The microscope is one of the microbiologist's most important tools. It allows for the visualization of small particles, including microbes, which are too small to be seen with the human eye. With the help of proper illumination, a microscope can magnify a specimen and optically resolve fine detail. This introduction to microscopy will include an explanation of features and adjustments of a compound brightfield light microscope, which magnifies images using a two lens system.
These microscopes combine an ocular lens (located in the eyepiece) and an objective lens (located above the stage and attached to a revolving nosepiece). Objective lenses can be selected based on the amount of magnification needed. Objective lenses are changed by turning the revolving nosepiece. The ocular lens remains constant and is not changed during normal microscope operation.
To view a sample placed on the microscope stage, the course focus knob and the fine focus knob are used to move the stage up and down. This will enable focusing on the sample.
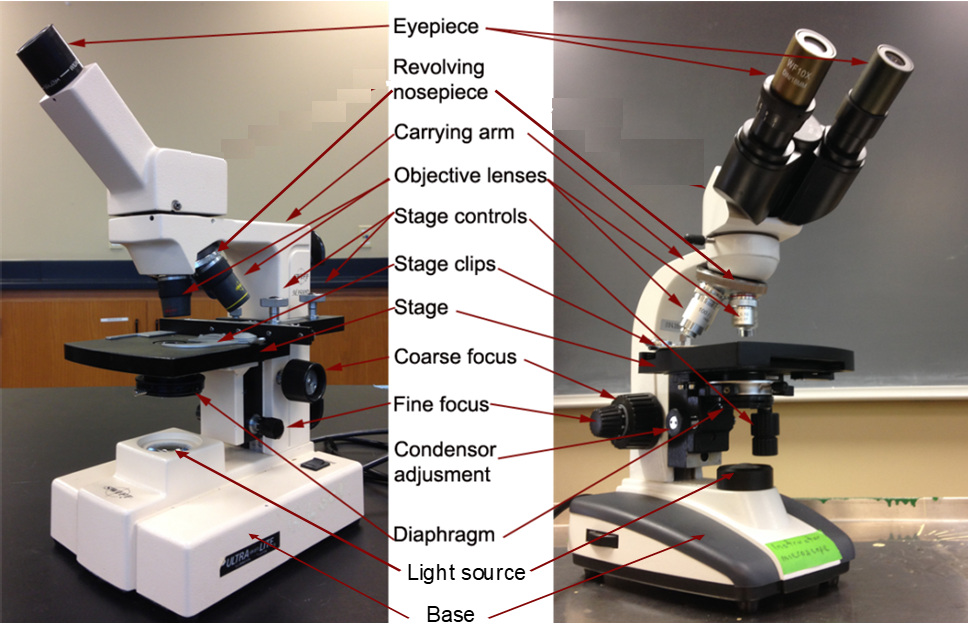
Microscope Part |
Description and Function of Microscope Part |
---|---|
eyepiece |
location for looking into the microscope; holds ocular lenses (lenses magnify the image; typically have 10X magnification) |
revolving nosepiece |
contains the objective lenses; the nosepiece turns to change the objective lenses (changes the magnification) |
objective lenses |
mounted in the revolving nosepiece; typically microscopes have four objective lenses: 4X, 10X, 40X and 100X |
stage clips |
holds the microscope slide in place; located on the stage |
stage |
a platform located below the objectives where the slide is placed |
stage controls |
knobs that move the slide around the stage by moving the stage clips to position the specimen in the desired position |
mechanical stage |
the mechanism that moves the stage clips around the stage to move the slide; the stage control knobs control the mechanical stage |
diaphragm |
located beneath the condenser; regulates the amount of light by opening or closing its aperture |
light source/ illuminator |
a light source; usually a bulb built in the base of the microscope; there may be a dial to regulate the intensity of light depending on the model of microscope |
condenser |
a system that focuses the light coming up from the illuminator onto the specimen on the slide |
arm |
a structure of the microscope that connects the base to the head; it may be straight or curved depending on the model of microscope |
base |
the wide bottom of the microscope; supports the microscope on a bench or table |
coarse focus |
large knob located on both sides of the microscope below the stage; focuses the image by moving the stage rapidly; should only be used when using the 4X or 10X objective lenses; using the coarse focus with the 40X or 100X objective lenses could damage the lens or crack the slide |
fine focus |
small knob located on both sides of the microscope below the stage; focuses the image by moving the stage in small increments; used most with the 40X objective lens and 100X objective lens but can be used to fine-tune focus focus at lower objective lenses (4X and 10X) |
Tips for Success Using a Light Microscope
- Center the sample over the light shining up through the stage.
- Begin with the lowest magnification objective lens in place (usually the 4X objective lens). Make sure it clicks into place over the sample and the light shining up through the stage.
- Turn the course focus to bring the stage up as close as it can go to the objective lens. At this point, you should be close to being in focus.
- Look into the eyepiece and slowly turn the course focus until you can see the sample. Many samples have been stained to have color (usually you can look for color - often pink, purple, or blue - you may be able to see the color on the slide with your naked eye).
- Increase the objective lens magnification one step at a time. Focus the microscope each step (with course focus at 4X and 10X objective lenses and with fine focus at 40X and 100X objective lenses).
- If you lose the sample, return back to the lowest magnification objective. This may seem like you are wasting time, but in reality it is the fastest way to find your sample again.
Detailed Instructions for Using a Light Microscope
Learning microscopy is very important in microbiology to examine the microscopic organisms that we are studying (bacteria, protozoa, fungi, etc.). Here is how we work with these microscopes:
Carrying a Compound Light Microscope
To take the microscope out of the cabinet or off of the cart using the following instructions:
- Use your dominant hand to hold the arm of the microscope, and your non-dominant hand to hold the base of the microscope.
- Carry the microscope upright. That way the ocular lenses located in the eyepiece do not fall out.
- Make sure the electrical cord is wrapped or removed from the microscope, avoid tripping on the cord.
To return the microscope to the cabinet or to the cart use the following instructions:
- Turn off the microscope.
- Turn the revolving nosepiece to have lowest magnification objective, usually the 4X objective lens.
- Disconnect from power source.
- Wrap cord around the microscope.
- If you used oil immersion, (the 100X objective), use lens paper only to remove any remnant of immersion oil on the 100X objective lens.
- Place the microscope back in the cabinet or on the cart using the transport instructions above.
Focusing a Compound Light Microscope
- Place the microscope on a table in front of you and plug it in such that the cord does not create a trip hazard for you or others in the laboratory.
- Turn the revolving nosepiece to have lowest magnification objective in place, usually 4X. The objective should click when properly in place.
- Place the slide on the stage of the microscope and properly position the stage clips such that the clips "hug" the slide on either side and are not on top of the slide since this could crack the slide (NOTE: there are some models of microscopes where stage clips do sit on top of the slide - ask your instructor about proper use of the stage clips for the model of microscope you are using).
- Turn on the microscope and confirm that the light is on.
- Move the stage control knobs to position the specimen on the slide directly over the path of the light.
- With the 4X objective in place, turn the course focus knob to bring the stage as close to the objective lens as possible.
- Look through the microscope and use the course focus knob to focus on the sample. Make sure the slide is in very sharp focus before moving on. Check with your instructor if you are uncertain.
- To focus on the specimen using higher magnification than with the 4X objective, use the next steps:
- With the specimen focused with the 4X objective, re-center the specimen using the stage adjustment knobs. DO NOT CHANGE THE FOCUS!
- Change to the next highest objective lens without changing any other settings on the microscope.
- Use ONLY the fine focus knob to modify the focus. The specimen should mostly be in focus and only minor adjustments should be necessary. Continue focusing until the image is sharp and clear.
- Re-center the specimen using the stage adjustment knobs. DO NOT CHANGE THE FOCUS!
- If moving to the next highest objective lens, change to the objective lens to the next highest without changing any other settings on the microscope.
- Use ONLY the fine focus knob to adjust the focus. Continue focusing until the image is sharp and clear.
**If you get lost on your sample, go back to 40X magnification (objective says ‘4X’), re-focus, and follow the steps above again.**
Video Walkthrough for Using a Light Microscope
Magnification
The optical system of a compound microscope consists of two lens systems: one found in the objective(s) lens(es) (Fig. 2, part 3); the other in the ocular (eyepiece) (Fig. 2 part 1). The objective lens system is found attached to a rotating nosepiece (Fig. 2, part 2). A microscope usually has three or four objectives that differ in their magnification and resolving power. Magnification is the apparent increase in size of an object. Resolving power is the term used to indicate the ability to distinguish two objects as separate. The most familiar example of resolving power is that of car headlights at night: at a long distance away, the headlights appear as one light; as the car approaches, the light becomes oblong, then barbell-shaped, and finally it becomes resolved into two separate lights. Both resolution and magnification are necessary in microscopy in order to give an apparently larger, finely detailed object to view.
Look at the engravings on the objective lenses and note both the magnification (for example: 10X, 40X, 100X) and the resolution given as N.A. = numerical aperture, from which the limit of resolution can be calculated:
microscope resolution limit = (wavelength)/(numerical aperture x 2)
At a wavelength of 550 nm (0.55 µm), the 100X objective lens with a N.A. of 1.25 has a resolving power of 0.22 µm. Visible light has of wavelength from about 400-750 nanometers (nm). Since the limit of resolution decreases at the shorter wavelengths, microscopes are usually fitted with a blue filter. The resolving power of the lens separates the details of the specimen, and the magnification increases the apparent size of these details so that they are visible to the human eye. Without both resolution and magnification, you would either see nothing (good resolution, no magnification) or a big blur (poor resolution, good magnification).
The objective lens system produces an image of the specimen, which is then further magnified by the ocular lens (eyepiece). The magnification of this lens is engraved on the ocular. The total magnification of the microscope is determined by the combination of the magnification of the objective lens and ocular lens that is in use, that is:
total magnification = (objective lens magnification) x (ocular lens/eyepiece magnification)
For example, with a 10X objective lens and a 10X ocular, the total magnification of the microscope is 100X. If the objective lens is changed to a 20X objective, then the total magnification is now 200X, whereas if a 10X objective is used with a 12.5X ocular lens, the total magnification is now 125X. When viewing a sample, magnifications are reported as the total magnification. Therefore, if making an illustration or taking a photograph of a magnified sample, report the magnified view as the total magnification (not as the magnification of the objective lens). Likewise, if you are examining a magnified image taken by another person, the magnification they report is the total magnification (not the objective lens). When reading laboratory instructions for viewing a sample with the microscope, total magnification will be given (not the objective lens).
The use of objective and ocular lenses with different magnifications allows greater flexibility when using the compound microscope. Due to the size of most bacteria (ranges widely from ~1 µm to over 100 µm), generally we require the use of the 100X oil immersion lens with a 10X ocular lense to view bacteria in a standard brightfield light microscope.
Sample Illumination
The objective lens and ocular lens systems can only perform well under optimal illumination conditions. To achieve these conditions, the light from the light source (bulb) must be centered on the specimen. The parallel light rays from the light source are focused on the specimen by the condenser lens system. The condenser can move up and down to affect this focus. Finally, the amount of light entering the condenser lens system is adjusted using the condenser diaphragm. It is critical that the amount of light be appropriate for the size of the objective lens receiving the light. This is important to give sufficient light, while minimizing glare from stray light, which could otherwise reduce image detail. The higher the magnification and resolving power of the lens, the more light is needed to view the specimen.
Oil Immersion
Objective lenses used for observing very small objects such as bacteria are almost always oil immersion lenses. With an oil immersion lens, a drop of oil is placed between the specimen and the objective lens so that the image light passes through the oil. Without the oil, light passing through the glass microscope slide and specimen would be refracted (bent) when it entered the air between the slide and the objective lens. This refracted light might still be able to contribute to the image of the specimen if the objective lens is large. However, at the higher magnification, the objective lens is small, so is unable to capture this light. The loss of this light leads to loss of image detail. Therefore, at higher magnifications, the area between the slide and the lens is modified to have the same (or nearly the same) refracting qualities (refractive index) as the glass and specimen by the addition of immersion oil. Watch the video below and read this article for more information about oil immersion.
To use an oil immersion lens, place a drop of oil on top of the dried specimen on the slide and carefully focus the microscope so that the objective lens is immersed in the oil. Any lens, which requires oil, is marked "oil" or "oil immersion." Conversely, any lens not marked "oil" should not be used with oil and is generally not sealed against oil seeping into and ruining the objective.
Electron Microscopes (EM)
In contrast to light microscopes, electron microscopes use a beam of electrons instead of a beam of light. Not only does this allow for higher magnification and, thus, more detail, it also provides higher resolving power. The method used to prepare the specimen for viewing with an electron microscope kills the specimen. Electrons have short wavelengths (shorter than photons of light) that move best in a vacuum, so living cells cannot be viewed with an electron microscope.
The best type of EM to examine a cell's internal structures such as vesicles, storage granules, ribosomes, mitochondria, and nuclei is transmission electron microscopy (TEM) since it is designed to allow electrons to pass through a thin sample. Samples must be prepared for TEM by embedding the sample, such as a cell, in a tiny plastic block, and using either a glass knife or a diamond knife to thin-section the sample using a microtome (laboratory equipment that makes thin sections). As a result, thin slices of cells can be observed with TEM, exposing intracellular details. An example of a thin-sectioned skin cell magnified by TEM is shown in the figure, part (a) below.
Another common type of EM is scanning electron microscopy (SEM). SEM works much differently than TEM since SEM radiates electrons onto a sample’s surface and these electrons are reflected back by the sample to elucidate the surface details of the sample. As a result, SEM is an excellent technique for examining the three-dimensional surface of an un-sectioned specimen and viewing only the external surfaces of cells and tissues. An example of an SEM image of skin cells with yeast infecting them is shown in the figure, part (b), below.
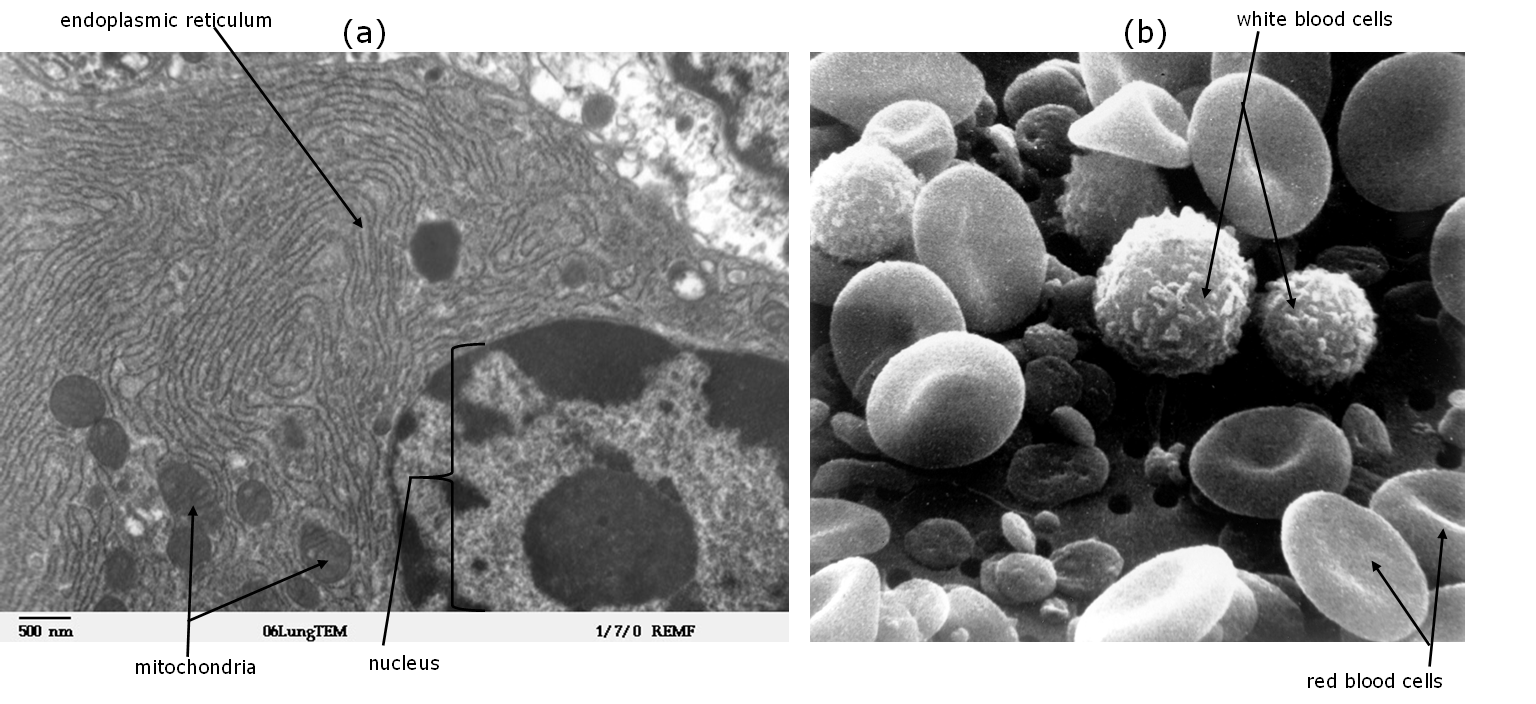
Attributions
- Anatomy and Physiology I Lab" by Victoria Vidal is licensed under CC BY 4.0
- BIOL 250 Human Anatomy Lab Manual SU 19 by Yancy Aquino, Skyline College is licensed under CC BY-NC-SA 4.0
- Biology 2e by Mary Ann Clark, Matthew Douglas, Jung Choi, OpenStax is licensed under CC BY-NC 4.0
- Chapter Image: Researcher looks through microscope.jpg by Rhoda Baer (Photographer) and National Cancer Institute is in the public domain.
- Clara cell lung - TEM by Louisa Howard is in the public Domain
- MB352 General Microbiology Laboratory 2021 (Lee) by Alice_Lee@ncsu.edu has an undeclared license.
- SEM blood cells by Bruce Wetzel, Harry Schaefer is in the public Domain