1.10: DNA and Restriction Enzymes
- Page ID
- 75797
Deoxyribonucleic acid (DNA) is located in the nucleus of eukaryotic cells (animals, plants, fungi, and protists). DNA contains information to direct the cell in the manufacture of proteins. Proteins control development, organ function, metabolism, enzymatic reactions, photosynthesis, muscle action, brain activity, and many other cellular processes. DNA is often referred to as the “blueprint for life”.
DNA is a polymer composed of the nucleotide bases guanine (G), adenine (A), thymine (T), and cytosine (C), and two deoxyribose sugar/phosphate backbones (Fig. 1). Two DNA strands are twisted to form a double helix. DNA is wound tightly around histone proteins to form the thread-like structures called chromosomes. Cells of eukaryotic organisms contain multiple linear chromosomes. Prokaryotic organisms, such as single-celled bacteria like Escherichia coli (E. coli), contain a single circular chromosome.
A gene is a sequence of nucleotide bases (DNA) that codes for a specific protein. Every species has genes that code for proteins, but different species have varying numbers of genes. Human DNA, for example, contains about 20,000 genes, while the cells of the rice plant contain over 40,000 genes. The 3 billion nucleotide base pairs in the human genome are located on 46 chromosomes. The Human Genome Project has determined the order of the nucleotides on each chromosome, and thus the location of each gene. Despite the differences between the structure and number of chromosomes and genes in organisms, the DNA functions the same way in all organisms to encode proteins.
Exercise 1: DNA EXTRACTION
The first step in working with nucleic acids (DNA and RNA) is to remove the molecules from inside the cell. Different types of cells need to be processed differently in order to release nucleic acids. All cells have a cell membrane, a phospholipid bilayer that separates the internal environment of the cell from the external environment. In eukaryotes, DNA is housed inside the nucleus of the cell which is surrounded by the nuclear membrane, a second double-layered membrane, also composed largely of lipid molecules. When extracting DNA from plant cells, the cell wall must also be considered; some types of plant tissue require grinding or flash-freezing in order to break the tough cell wall.
In the DNA isolation procedure, plant cell walls and cell membranes are broken down by blending or mashing and heating the cells. Detergent in the extraction solution dissolves phospholipids in the cell membrane causing the cells to lyse. When cells undergo lysis, the cellular components, including the DNA, are released. The technique of filtration uses a medium, in this case, cheesecloth, to separate solids from liquids. The resultant material is referred to as filtrate. When cold ethanol is added to the filtrate, DNA precipitates at the water/ethanol interface. Although an individual DNA molecule is not visible with the naked eye, DNA isolated from large quantities of cells can be observed.
Strawberry fruit tissue is an excellent type of tissue to use for the demonstration of DNA extraction. First, ripe strawberries are soft and juicy; as the fruit matures, the cells fill up with water and sugar, which make the fruit so delicious! Second, as the strawberry ripens, a series of chemical reactions take place within the cells that lead to the breakdown of long-chain polysaccharides, like cellulose and pectin, that make the cell wall tough. Lastly, cultivated strawberries (Fragaria x ananassa) are the product of a hybridization between two other strawberry species, and they have an octoploid genome, meaning they have eight sets of chromosomes (Fig. 2) inside their cells and just under 1 billion DNA base pairs. Similarly, wheat (Triticum aestivum) is hexaploid but is likely the product of several hybridization events between three different related species and contains about 17 billion DNA base pairings! These translate to lots of molecules of DNA, which increases our yield and makes the DNA easier to visualize.
Materials:
- Isopropyl alcohol 91% (rubbing alcohol) or 95% ethanol, Chilled in the freezer
- Graduated cylinders
- Salt
- DI Water
- Cheesecloth
- Dishwashing liquid (preferably, Dawn)
- Wide-mouth glass test tubes
- Funnel
- Disposable plastic cups
- Microcentrifuge tubes
- Wooden stirrer or glass rod
- Resealable plastic bag
- 100 or 200 mL beaker
- Ice buckets with ice
- A DNA source (about 1 cubic inch of food)
- strawberries
- bananas
- ground flax seed
- ground wheat germ
- peas
- broccoli
- spinach
Preparation
- Have isopropyl alcohol (rubbing alcohol) or ethanol cooled in an ice bucket
- Prepare your food item if needed (i.e. remove green strawberry tops, peel banana, grind wheat germ, etc)
- If not already made, prepare DNA extraction (lysis) buffer by combining the following:
• 45 mL DI water
• 5mL liquid dish soap
• 0.75 g NaCl (table salt)
Procedure
- Place the food item (i.e 2 large strawberries) into the plastic zipper bag. Seal it and gently smash the food with your hands for about 2 minutes. Completely crush the food (strawberries) to disrupt the cells.
- Open the plastic zipper bag and add 10mL of the DNA extraction (lysis) buffer. Squeeze the bag to remove all air and seal the bag tightly.
- Gently (to prevent over-sudsing or excessive foaming), but thoroughly, continue to crush the food (strawberries) inside the bag for about one minute or until it is a slushy consistency.
- Completely line the funnel with a layer of cheesecloth. Place the funnel into the wide-mouth test tube.
- Pour the food juice/DNA extraction buffer mixture into the funnel so the juice passes through the cheesecloth and into the test tube. Use the cheesecloth to strain the mixture so that only the juice flows into the tube and the pulp is retained in the cheesecloth.
- Discard the cheesecloth and the pulp. Remove the funnel from the tube. The glass tube now contains a liquid called “filtrate”.
- Carefully and slowly pipette an equal volume of ice-cold ethanol on top of the filtrate in the test tube using the plastic transfer pipette. The alcohol is less dense than the filtrate and will float as a layer on top of the filtrate. Do not mix or stir!
- Hold the tube still at eye level and observe what happens at the interface of the alcohol and the filtrate. DNA will precipitate at the alcohol-lysis buffer interface. This means it will come out of solution into a “solid” form and appear as fluffy white cotton or cloudy material. Verify with your instructor that you have isolated DNA.
- (optional) Use your wooden stirrer or glass rod to transfer your extracted DNA into a microcentrifuge tube. Add a small amount of ethanol to the tube to prevent your DNA from drying out.
Discussion
This protocol for extraction of DNA is based largely on the principle of solubility. Solubility refers to the ability of one substance (the solute) to dissolve in another substance (the solvent). Recall that polar substances dissolve easily in polar solvents, but do not dissolve easily in nonpolar solvents, a phenomenon commonly referred to as “like dissolves like.” Water is a polar solvent, and molecules that dissolve easily in water are referred to as hydrophilic. DNA molecules are hydrophilic because the sugar-phosphate backbone of the molecules is highly polar. This means that DNA dissolves in water, so in this experiment, the DNA that is released when the cells are crushed dissolves in the juice/extraction buffer mixture.
Remember, there are two key ingredients in the DNA extraction buffer aside from the water: dish soap and salt. The dish soap acts to break up the phospholipid molecules that form the cell membrane and the nuclear membrane, which lyses the cell and releases the cellular contents, including DNA. The salt has two functions in the extraction process. It helps to neutralize the charge on the sugar-phosphate backbone, making DNA less soluble in water and allows it to more easily precipitate when the alcohol is added. The salt also helps to remove the proteins that are bound to the DNA and to keep the proteins dissolved in the lysis solution.
Although the chemical reactions described above are all happening when you add the buffer and crush the food (strawberries), they are not visible with the naked eye. However, the addition of the cold ethanol caused a much more dramatic result! Ethanol is a nonpolar solvent, and when it is added to the juice extract, the DNA precipitates out of the solution. A precipitation reaction is a chemical reaction that causes a solid substance to emerge from a liquid solution. In this experiment, the addition of ethanol to the reaction forces the DNA to precipitate out of solution, which we can then spool onto the wooden stirrer or glass rod.
Exercise 2: Modeling of DNA Structure
The DNA molecule is a complex, yet simple molecule. Only four different nucleotide building blocks (adenine, thymine, guanine and cytosine) are used to form the DNA sequences that encode for all of the proteins in our bodies. Each nucleotide has three components: a negatively charged phosphate molecule, a deoxyribose sugar and a nitrogenous base (Fig. 3). On each strand of DNA the phosphate and deoxyribose sugar are arranged on the outside of the DNA molecule, forming a “backbone.” The nitrogenous bases are positioned in the interior of the double helix. Each strand of DNA is complementary to the other, meaning that the nitrogenous bases of the two strands are always paired in specific combinations, called base pairing. If there is an adenine in one strand, then it is paired and directly across from a thymine in the other strand. If there is a cytosine in one strand then it is paired with a guanine in the other strand. Therefore, if you know the sequence of one of the strands of DNA, then you can deduce the sequence of the opposite (complementary) strand. Hydrogen bonds between the nitrogenous bases hold the two strands of DNA together.
The DNA strands also have directionality, referred to as the 5’ (5 prime) and 3’ (3 prime) ends. When looking at a strand of DNA, the end that has a free phosphate group is the 5’ end. The end of the DNA strand that has a free -OH group on the 3’ carbon of the deoxyribose sugar is referred to as the 3’ end (see Fig. 1). The two DNA strands are antiparallel to one another, running in opposite directions like opposite lanes of a street.
Materials:
This lab manual will utilize the Carolina BioKits: DNA Simulation Kit. Your instructor may provide you with an alternative DNA Simulation Kit and instructions. A simple modeling activity using candy is discussed here.
The Carolina DNA modeling kit uses different colored beads to represent the phosphate group, deoxyribose sugar and the four different nitrogenous bases. You will use the beads to build thymine, adenine, guanine and cytosine nucleotides. Then, you will link the individual nucleotides together to “build” a strand of DNA.
Procedure: (If using an alternative DNA simulation kit, follow the directions given by your instructor.)
- Obtain from your instructor the DNA sequence that you are instructed to model. If you are provided with only the sequence of one strand, then you will need to determine the sequence of the complementary strand.
- Begin assembling individual nucleotide bases, each consisting of one phosphate, one sugar and one nitrogenous base. Note which end of the nucleotide is the 5’ end and which is the 3’ end.
- Link the nucleotide bases together in the correct sequence to build one of the strands of DNA. Pay close attention to orient the 5’ end (with the free phosphate) in the correct direction and the 3’ end in the correct direction.
- Now, you will build the complementary strand of DNA. Link the nucleotides together in the correct sequence so that they are complementary to the other strand. Be sure that the nucleotides are oriented in the correct direction, with the 5’ end and 3’ end on the appropriate ends of the strand. Your two DNA strands should be antiparallel to one another.
- Position the two strands together to check that base pairing is correct and that the strands are antiparallel.
- Use the plastic connectors to attach the paired nitrogenous bases of the two strands together. The plastic connectors represent the hydrogen bonds that hold the two DNA strands together.
- Twist the double stranded DNA model into a double helix. Check your model with your instructor.
Exercise 3: DNA Fingerprinting
DNA fingerprinting is routinely used today to establish paternity, to diagnose inherited disorders, and for use in criminal cases. DNA fingerprinting enables forensic investigators to determine whether two DNA samples originate from the same individual. Not all of the DNA present in a sample is used in an analysis. Restriction enzymes act as molecular scissors and are used to cleave DNA molecules at specific sequences of DNA. Over 2,500 different restriction enzymes have been identified. These enzymes are produced by bacteria and are used by the bacteria to destroy foreign DNA such as bacteriophages - viruses that infect and replicate within a bacterium. Each restriction enzyme cleaves DNA at a specific nucleotide sequence. For example, the restriction enzyme EcoR1, isolated from E. coli, cuts DNA at the sequence GAATTC (Fig. 4).
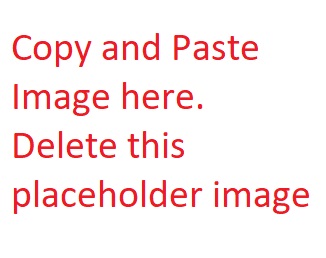
The length and the number of the fragments produced depend upon the frequency and the distance between the recognition sites. This distinct pattern is known as restriction fragment length polymorphisms (RFLP’s) which are unique to each individual, therefore forming a DNA fingerprint. After DNA samples are cut by restriction enzymes, the fragments are separated using gel electrophoresis. PCR, polymerase chain reaction, can be used to amplify trace amounts of DNA in a sample to levels that can be analyzed using restriction enzymes. The length of the segments analyzed is much smaller and the repeat sites are called microsatellites.
Materials:
- DNA sequences in Figure 6
- Highlighter
- Scissors
Procedure:
You will use DNA fingerprinting technology to determine which male dog is the real father of a puppy, Willy. DNA sequences from each dog are on the next page. In this DNA fingerprinting scenario, the restriction enzyme, Haelll, will be used to digest each DNA sequence. The recognition sequence and cut site of Haelll is shown below (Fig. 5).
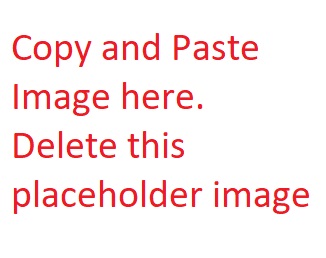
- Cut the DNA sequence (Figure 6) paper into strips to separate the DNA sequences of each dog into one long strip. You will have 5 strips of DNA sequences (one for each dog).
- Scan each sequence of DNA for the Haelll sequence. There may be more than one Haelll sequence in the DNA sequences. Be sure to scan the entirety of each DNA sequence. When you find the recognition sequence, use a highlighter to highlight the recognition sequence.
- At each recognition site, use a pencil to draw a vertical line between both strands of DNA to indicate exactly where the restriction enzyme will cut the DNA, separating it into 2 pieces (refer to Fig. 5).
- Use scissors to cut the DNA sequences at the indicated restriction sites you marked.
- Count the number of nucleotides on one strand of each resulting fragment of DNA. Write the nucleotide length on each fragment.
- For each dog, arrange the DNA fragments from largest to smallest to simulate how they would separate when analyzed via gel electrophoresis. Record those DNA fragment sizes in the table below.
Size of DNA fragments |
Mom |
Willy |
Sire X |
Sire Y |
Sire Z |
---|---|---|---|---|---|
Largest (60-51 bp) |
|||||
(50-41 bp) |
|||||
(40-31 bp) |
|||||
(30-21 bp) |
|||||
(20-11 bp) |
|||||
<10 bp |
|||||
smallest |
- Compare the DNA fragments from Willy to the DNA fragments of the Mom and possible fathers. Every fragment in Willy’s digest will be found in either the Mom or Sire’s digest. This comparison will identify the real sire of Willy.
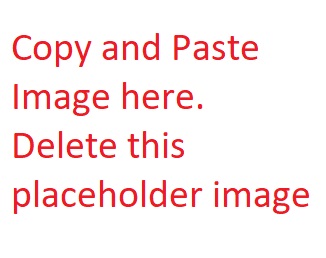
Questions for Review
- How well do you understand the necessity of each step in the DNA extraction procedure? Match each of the procedures below with its function, by placing the appropriate letter on the line provided.
Procedure
- Filter strawberry slurry through cheesecloth
- Mix strawberry with salty/soapy solution
- Initial smashing and grinding of strawberry
- Addition of cold ethanol to filtered extract
Function
_____ To precipitate the DNA from solution
_____ Separate components of the cell
_____ Physically disrupt the cells
_____ Break up proteins and dissolve cell membranes
- Briefly describe what precipitation means. Why was this step necessary?
- Relate what you know about the chemical structure of DNA to what you observed during the precipitation step.
- Briefly explain why strawberry fruit tissue is a great source for extracting DNA.
- Why is it important for scientists to be able to extract DNA from living organisms? State at least 2 reasons.
- What is the function of genomic DNA and where is it found in a eukaryotic cell?
- You are given the DNA sequence below. Using the base-pairing rules, draw the complementary DNA strand in the space below. Make sure to designate the 5’ and 3’ ends appropriately.
5’ - ATTCGCTCG - 3’
- Why might DNA fingerprinting be more useful in identifying individuals than blood typing analysis?
- What are the building blocks that compose DNA? What are the three specific components that make up these building blocks?
- DNA sequences contain palindromes. Briefly explain what a palindrome is in DNA and give an example.
- What biological molecules, which are isolated from different types of bacteria, act as molecular scissors to cut DNA at specific locations? What advantage do you think bacteria gain by having these particular molecules?
Practical Challenge Questions
- For the DNA sequence shown below, use the base-pairing rules to draw the complementary DNA sequence. Be sure to designate the polarity of the resulting strand.
5’ ATATCATGGAATTCGATCCTAG 3’
- Now, “digest” your DNA molecule using EcoRl enzyme. The EcoRl recognition sequence is shown below. Draw a vertical line in your DNA sequence above to indicate specifically where the restriction enzyme would cleave the DNA.
References
Belwood, Jacqueline; Rogers, Brandy; and Christian, Jason. Foundations of Biology Lab Manual (Georgia Highlands College). “Lab Activity: DNA Extraction from Strawberries,” (2019). Biological Sciences Open Textbooks. 18. CC-BY https://oer.galileo.usg.edu/biology-textbooks/18
Natale, E. G., Laura Blinderman, &. Patrick. (2021, March 19). Book: Unfolding the Mystery of Life - Biology Lab Manual for Non-Science Majors (Genovesi, Blinderman & Natale). “Lab Exercise 11: Isolation of DNA from Plants.” CC-BY Retrieved April 5, 2021, from https://bio.libretexts.org/@go/page/24114
Sanver-Wang, Dilek. College of the Canyons, General Biology BioSci100 Laboratory Manual, Building Knowledge through Experiments 4th Edition. “Lab 9: Analysis of DNA.” CC-BY.