32.2: Exercise
- Page ID
- 105973
\( \newcommand{\vecs}[1]{\overset { \scriptstyle \rightharpoonup} {\mathbf{#1}} } \)
\( \newcommand{\vecd}[1]{\overset{-\!-\!\rightharpoonup}{\vphantom{a}\smash {#1}}} \)
\( \newcommand{\id}{\mathrm{id}}\) \( \newcommand{\Span}{\mathrm{span}}\)
( \newcommand{\kernel}{\mathrm{null}\,}\) \( \newcommand{\range}{\mathrm{range}\,}\)
\( \newcommand{\RealPart}{\mathrm{Re}}\) \( \newcommand{\ImaginaryPart}{\mathrm{Im}}\)
\( \newcommand{\Argument}{\mathrm{Arg}}\) \( \newcommand{\norm}[1]{\| #1 \|}\)
\( \newcommand{\inner}[2]{\langle #1, #2 \rangle}\)
\( \newcommand{\Span}{\mathrm{span}}\)
\( \newcommand{\id}{\mathrm{id}}\)
\( \newcommand{\Span}{\mathrm{span}}\)
\( \newcommand{\kernel}{\mathrm{null}\,}\)
\( \newcommand{\range}{\mathrm{range}\,}\)
\( \newcommand{\RealPart}{\mathrm{Re}}\)
\( \newcommand{\ImaginaryPart}{\mathrm{Im}}\)
\( \newcommand{\Argument}{\mathrm{Arg}}\)
\( \newcommand{\norm}[1]{\| #1 \|}\)
\( \newcommand{\inner}[2]{\langle #1, #2 \rangle}\)
\( \newcommand{\Span}{\mathrm{span}}\) \( \newcommand{\AA}{\unicode[.8,0]{x212B}}\)
\( \newcommand{\vectorA}[1]{\vec{#1}} % arrow\)
\( \newcommand{\vectorAt}[1]{\vec{\text{#1}}} % arrow\)
\( \newcommand{\vectorB}[1]{\overset { \scriptstyle \rightharpoonup} {\mathbf{#1}} } \)
\( \newcommand{\vectorC}[1]{\textbf{#1}} \)
\( \newcommand{\vectorD}[1]{\overrightarrow{#1}} \)
\( \newcommand{\vectorDt}[1]{\overrightarrow{\text{#1}}} \)
\( \newcommand{\vectE}[1]{\overset{-\!-\!\rightharpoonup}{\vphantom{a}\smash{\mathbf {#1}}}} \)
\( \newcommand{\vecs}[1]{\overset { \scriptstyle \rightharpoonup} {\mathbf{#1}} } \)
\( \newcommand{\vecd}[1]{\overset{-\!-\!\rightharpoonup}{\vphantom{a}\smash {#1}}} \)
Modeling a Food Web
Draw a food web. If you are on a field trip, include organisms that you see in the same area, interacting together. If in the lab, try to include organisms that you have seen or learned about. Make sure to include a primary producer, at least two levels of consumers, and a decomposer. Label which organisms are autotrophs and which are heterotrophs. Use arrows to show how energy is transferred and lost throughout this web.
What is the ultimate source of energy for your food web?
What happens to this energy as it moves through the food web? Where does it end up?
Nutrient Cycling in Ecosystems
In drawing your food web, you depicted a combination of the flow of energy and the cycling of nutrients through an ecosystem. Where as energy has an input and an output, nutrients are continually recycled. These elements often cycle between the biotic and abiotic components in an ecosystem.
For example, water is composed of hydrogen and oxygen atoms. Water molecules precipitate from the atmosphere and fall as rain, saturating the soil. A plant absorbs those molecules through its roots, transporting them up to its leaves. Some of these water molecules will be broken apart during the process of photosynthesis, the oxygen exiting the plant as \(\ce{O2}\) gas that you will breathe in while the hydrogen might be used to form molecules of glucose that you will eat. The rest of the water exits the plant through its stomata, evaporating back into the atmosphere in a process called transpiration, the evaporation of water from plant tissues. The oxygen atoms that you breathed in will be used for cellular respiration and be joined back to hydrogen molecules to once again form water. This water might exit your body as vapor on your breath or as perspiration and return directly to the atmosphere. It might also soak into your clothes as sweat or exit as urine, be processed in a wastewater facility, then sent out to the ocean where it will evaporate back into the atmosphere.
The atoms of hydrogen and oxygen are broken apart and reassembled into other molecules multiple times in this process, but the overall outcome is a cycle, with atoms traveling between the atmosphere, hydrosphere, lithosphere, and biosphere, but rarely ever exiting our Earth system.
In the space below, diagram the flow of water through the ecosystem around you.
What is the role of plants in the global cycling of water?
Mycorrhizal Networks
A symbiosis (sym- meaning shared, bio- meaning life) is when two or more organisms of different species live in close proximity to one another, sharing some aspect of their life cycle. A mutualism is a type of symbiosis in which both partners get a net benefit from the interaction. In other types of symbiosis, only one partner benefits and in a parasitic symbiosis, it is to the detriment of the other partner.
Most plants form a mutualistic symbiosis with fungi called a mycorrhizal relationship (myco- meaning fungus, rhiz- meaning root). In this particular partnership, the fungus enters the plant through the roots and takes sugars from the plant. In exchange, the plant takes water and dissolved nutrients (particularly nitrogen and phosphorus) from the fungus. Though both of these organisms lose something in the exchange, it is something that they tend to have a surplus of, while the thing they get in return is one that they would not have access to alone.
The fungus obtains its sugars from the living plant tissue. What terms could you apply to this organism?
The plant synthesizes its own sugars during photosynthesis. What terms could you apply to this organism?
Somewhat recently, a researcher named Suzanne Simard began tracking the exchange of nutrients through mycorrhizae. Not just from tree to fungus, but from tree to tree via connections to the same mycorrhizal partner. By providing certain trees with carbon dioxide containing a heavier isotope of carbon (C14), Dr. Simard could track the sugars formed from those heavy carbon atoms as they moved from tree to tree. What she found was that the connections extended beyond just one tree to another, but that almost all of the trees within her plot were connected through a network of different mycorrhizal fungi. Trees of different species and fungi of different species could exchange nutrients with each other. Further study revealed that the exchange was not limited to nutrients and water, but also included transfer of plant defense compounds that worked like raising an alarm, cuing the other plants to start producing defensive chemicals.
How might this information change the way we study ecosystems?
Sketch a few of the above ground plants that you can see (or imagine) and connect them below ground via a mycorrhizal network. Use arrows to indicate the flow of sugar through this network. Add in some disturbance that would set off a plant’s immune system (attack of the caterpillars, fungal infection, etc…) and depict the movement of the signal throughout the plant community.
Some plants have evolved to be parasitic by taking advantage of the mycorrhizal network. These plants no longer photosynthesize. Instead, they form relationships with mycorrhizal fungi that are attached to other plants, siphoning sugars from those plants through the fungal connection. For example, the parasitic plant Allotropa parasitizes the Matsutake mushroom, which is mycorrhizal with tanoaks and a few other trees.
Add a parasitic plant into your ecosystem above and use arrows to depict the movement of sugars.
Are these parasitic plants autotrophic or heterotrophic? How would you classify them, using ecology terminology? Explain your reasoning.
Each species in an ecosystem is considered a population. This represents a group of actually or potentially interbreeding organisms and is an important area of study for both ecologists and geneticists. When multiple species in an ecosystem are considered together, this is called a community. For example, a forest could be composed of a single population of redwood trees, but it is more likely to be a community, including other tree species like Sitka spruce and Douglas-fir, and animals like the Northern spotted owl or Coho salmon. All living organisms are considered to be a part of the community in a given ecosystem.
What is the difference between a community and an ecosystem?
Community Interactions
Pollination
One important community interaction we study in botany (in addition to mycorrhizae) is pollination. This is the transfer of pollen from one plant to another and can be mediated by animals, water, wind, or another mechanism. Since we are talking about community interactions, we will focus on animal pollination. Many flowering plants have evolved over time to attract a specific animal pollinator. Because the plant often provides food in the form of nectar or pollen, the pollinator often relies on this relationship as well and coevolves with the plant. One interesting example of this coevolution is the pollination of figs by parasitic wasps.
The Story of the Fig and the Wasp
Figs are made of many small flowers in an inside-out inflorescence called a syconium. How, then, are these flowers pollinated to develop into fruits? Figs are pollinated by tiny chalcid wasps known as fig wasps. In the spring, female fig wasps laden with pollen and fertilized eggs enter into the fig syconium through a small opening at the top. As she enters, her wings are ripped off, making her unable to leave again. She has reached her destination.
As she walks along the tops of the flowers, which are in their female stage, she lays her eggs inside the ovaries by inserting a long tube called an ovipositor down through the style.
Pollen falls off of her body onto the flowers and travels down the styles as well to fertilize the ovaries. Each flower she deposits an egg into will develop into a wasp instead of a fruit. However, some of the styles are longer than others, and in these she will not be able to lay her eggs. These flowers will develop into the fig fruit, while the others will serve as nurseries for the fig's strange pollinators.
The male wasps hatch first. Blind and wingless, they roam around the enclosed inflorescence, impregnating their still-sleeping sisters. As carbon dioxide builds up within the chamber, they begin to suffocate and burrow out through the sides of the figs, allowing oxygen to flow back in.
After their brothers have died, the females hatch, climbing over the flowers that are now in their male stage, collecting pollen and stuffing it into pouches on their bodies. The young female wasps, laden with pollen and fertilized eggs, leave the fig through the tunnels their brothers made and fly off to find a developing fig to lay their eggs and pollinate the enclosed florets. The timeline of a fig wasp's life is intricately interwoven into the phenology of the fig tree.
View the diagram on the following page and identify the stages in the connected life cycles of these organisms. If available in lab or on your field trip, cut open a fig to view the florets developing inside the syconium.
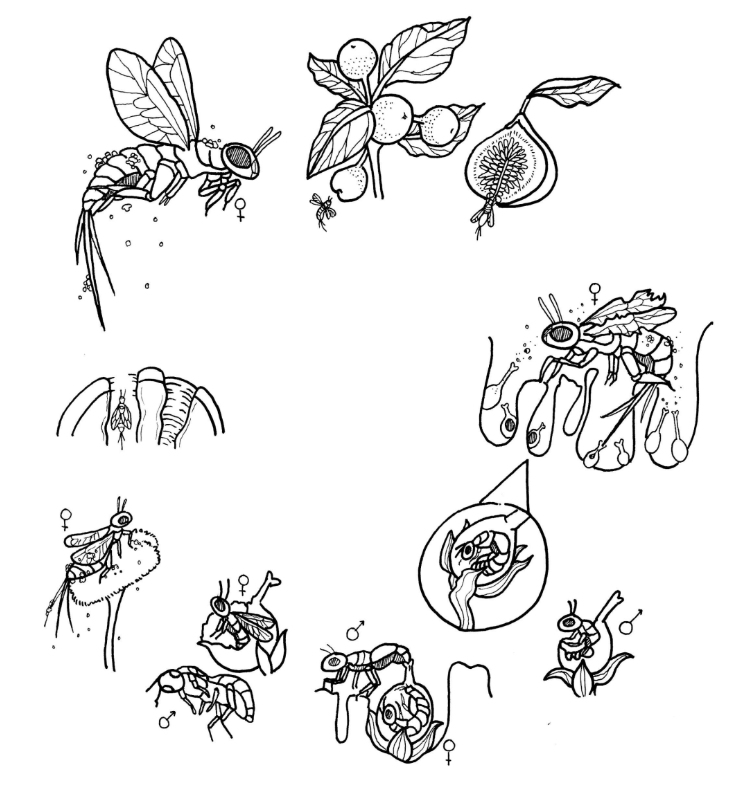
Is this relationship a parasitism or mutualism? Explain your reasoning.
Contributors and Attributions
Ecosystem & Eutrophication - Silver Springs
This lab will utilize the Silver Spring model, an analytical model, developed by H. T. Odum in 1957. Silver Springs is a real aquatic ecosystem located in central Florida. Odum developed his model to illustrate energy flow through the different trophic levels. The main organisms in Sliver Sping organized by trophic level are:
- First trophic level: Eelgrass and algae are the main photosynthetic organisms.
- Second trophic level: Invertebrates, turtles, and fish are the herbivores.
- Third tropic level: Both fish and invertebrates are carnivores and prey upon the herbivores.
- Fourth tropic level: The top carnivores consist of gar and bass, which eat the other fish species at lower trophic levels.
- Decomposers: Bacteria and crayfish are the main decomposers.
The Silver Springs model is on Blackboard as an Excel file. The general model structure is pictured on the next page. Odum’s model contains five state variables representing energy inputs into the four tropic levels and the decomposers. Flux rates describe the addition/removal of energy to/from the various parts of the system and the rate of transfer of energy between the components of the system. In the Excel file you can change the initial values (X) of any or all of the state variables and coefficients (P), and control the length of time simulated. Following a simulation “run” you will be easily able to view graphs of the state variables or community respiration versus time. To change the initial values, simply type over the current value and press return. Use the model to investigate the scenarios below:
We will use the model in two different scenarios in today’s lab, to understand eutrophication and to determine the impact of bread on the ecosystem.

Activity I
To understand more about eutrophication, you will conduct a laboratory experiment (Part 1) and use the Silver Springs computer model (Part 2)
Part 1
One potential way to decrease cultural eutrophication is by having higher trophic level organisms consume the algae. We will investigate this possibility using Daphnia, a zooplankton that feeds on green algae, and an alga species called Chorella. Although the information we gain from this activity is useful, it is an over simplification as to what might really occur in the aquatic environment.
We will use a colorimeter to measure the algae population. A colorimeter measures absorbance, the amount of light that is absorbed by a solution rather than the amount of light that can pass through. As the algae population increases (number of algae cells), the absorbance will also increase. It is a direct relationship.
Procedure
- Obtain two cuvettes and a transfer pipette.
- Fill one cuvette with distilled water. This cuvette is the blank
- Place the blank into the colorimeter and zero it. This provides a baseline measure for the experiment. Save the blank cuvette for later use.
- Using the disposable pipette, fill the second cuvette with the Chorella algae. Use the pipette to try and disperse the algae evenly throughout the cuvette.
- Add 10 daphnia to the cuvette and immediately measure the absorbance using the colorimeter. Record your reading in the table below.
- Remove the cuvette from the colorimeter and allow it to sit, undisturbed for 30 minutes.
- After 30 minutes, place the blank cuvette (with distilled water) back into the colorimeter. Use the blank to re-zero the machine.
- Remove the blank cuvette and put the algae/Daphnia cuvette into the colorimeter. Measure the absorbance and record your reading in the table below.
Table 1: Colorimeter data of Daphnia feeding on Chorella | |
---|---|
Absorbance value | |
Before feeding (time zero) | |
After feeding (time 30 minutes) |
Questions
- How did the absorbance change from time 0 to time 30 minutes? Did the absorbance increase, decrease, or stay the same?
- What does the absorbance value change tell you about the concentration of the Chorella? Did it increase, decrease, or stay the same?
- What does the absorbance value chance tell you about the behavior of the Daphnia? Did they consume Chorella? How do you know?
- In a real aquatic ecosystem, do you think zooplankton could decrease the impact of cultural eutrophication and algae blooms? Explain why or why not.
Part 2
Now that you have observed trophic interactions and cultural eutrophication in a lab experiment, apply that knowledge to the computer model(opens in new window). Use the model to analyze the sensitivity of the Silver Springs ecosystem to cultural eutrophication. Remember that alga is a photosynthetic producer on the first trophic level. The Daphnia would be one of the herbivores in the environment on the second trophic level. Change the levels in the model to simulate a eutrophication situation. View the graphs to see how the different trophic level populations change through the simulation.
Questions
Based on your findings with the model, answer the following questions:
- What is the maximum number of producers the ecosystem can support before higher trophic levels begin to decline?
- What happens to community respiration in the simulated algae bloom? Does it increase, decrease, or stay the same?
- What happens to the decomposer population in the simulated algae bloom? Does it increase, decrease, or stay the same? Why?
- Which group of carnivores (which trophic level) is more greatly impacted by the algae bloom? Why do you think this is the case?
Activity II
Eutrophication is not the only way that human activities affect aquatic ecosystems. Tourism in the Silver Spring area has grown lately as a result of more of vacationers visiting Central Florida. When visiting Silver Spring, tourists enjoy feeding the ducks. But, the ducks are gaining weight and becoming dependent on the tourists as a food source disrupting the normal food chain in the environment. Some environmental organizations have voiced displeasure regarding the dependence of ducks on bread handouts from visitors to the lake. Is it feasible to stop feeding the ducks or have they become too reliant on the tourists and the bread handouts?
Task
Use the model to analyze the sensitivity of long-term duck populations to an increase or decrease in bread input. There is a separate variable for bread and the ducks would be on the second trophic level as herbivores. View the graphs to see how the different trophic level populations change through the simulation.
QUESTIONS
Based on your findings with the model, answer the following questions:
- Is an elimination of bread feasible? Why or why not?
- How would the system respond to increasing levels of tourism, assuming bread levels also increased? What trophic levels are most impacted by increased levels of bread?
- What is the role of bread in the system, and how does its presence or absence impact the ecology of Silver Springs?
- What would be your suggestion to the managing board of Silver Springs? Should they completely eliminate the tourists feeding the ducks? Why or why not?
LICENSES AND ATTRIBUTIONS
CC LICENSED CONTENT, ORIGINAL
- Biology 102 Labs. Authored by: Lynette Hauser. Provided by: Tidewater Community College. Located at: [www.tcc.edu]. License: CC BY: Attribution
CC LICENSED CONTENT, SHARED PREVIOUSLY
- Trophic Levels. Authored by: Thompsma. Located at: [www.tcc.edu] [commons.wikimedia.org]. License: CC BY-SA: Attribution-ShareAlike
PUBLIC DOMAIN CONTENT
- Silver Spring Model. Authored by: Sholto Maud. Provided by: Wikipedia. Located at: [commons.wikimedia.org]. License: Public Domain: No Known Copyright
Ecology Game:
Islands have always provided scientists unique locations to learn about the natural world. Many of Charles Darwin’s key observations that led to his understanding of natural selection came from species living on the many islands that make up the Galapagos archipelago. For example, Darwin observed different species of finches were found on different islands and that the shape of the finches’ beaks corresponded to the type of food they consumed. Further research on the finches of the Galapagos by Dr. Peter Grant and Dr. Rosemary Grant found that even within an island, changes in environmental conditions (i.e. drought, disease) could influence the adaptations observed in the finches.
Because islands are isolated from the mainland and each other by water and often long distances, they can be colonized by new species that have intentionally or unintentionally dispersed to an island. In biology, we call this the founder effect, which is when a new population is established. A founding population is often small and has a different allele frequency than the original population. It is important to remember that every species that finds itself in a new habitat may not be ideally suited for the environmental conditions. Is the habitat, food, climate appropriate for the new species needs? Is there competition for any of these resources with species already living on the island? Large islands that are close to the mainland tend to support more species than small islands that are far from the mainland. As we discuss in class, we know that no two species can occupy the same ecological niche—basically, two species cannot co-exist if they are competing for the same resource. While niches can overlap significantly between two species, they cannot be exactly alike. Any habitat has a limited number of species that can be supported by the available resources. We know that the most successful species are those with adaptations best suited to the environment in which they find themselves and those that can best utilize the available resources. These individuals are able to survive and reproduce on the island and a new population is established there. Occasionally, if there is a mismatch in the individual’s traits and the environment in which they are located or the environmental conditions change unfavorably the individual may no longer succeed in that environment. This can lead to local extinction which is also known as extirpation. An extirpated species can recolonize if environmental conditions become favorable.
Today, you will be playing the role of an individual of one of the six different species on an ancient continent to the East in a game we call the Ecology Game. The objective of the game is to cross the ocean, colonizing islands as you go. However, every species in the game has its own ecological needs and each island along the way has specific environmental conditions and the ability to support different numbers of species. If you are a successful colonizer, a population of your species will exist on the island even after you have moved on. Please note that in order to colonize and establish a new population in real life, you would need a sexually mature male and female or a pregnant female. However, in this game, we are simplifying this concept by using a model of a single individual. Every species will not be able to colonize every island successfully and you have to compete with the other species who are also trying to make it to the new continent. The islands are of varying sizes and successful colonization may cover a small area or a large area depending on the size of the island. The winner is determined by a combination of the order that you reach the new continent and the island area (not how many islands, but how much island space) you have successfully colonized. Remember to think strategically! The Ecology Game is not always about speed, but thinking about your species, its ecological niche, and what we know about colonization and adaptation. Just because you are the first species to reach the new continent that does not mean you win the game. Think of today like an ecological game of chess!
Set up (per group—groups should have no more than 6 students):
- 1 board map
- 2 six-sided dice
- 1 cup of 6 species sticks
- 4 bags of colored game pieces (if groups are larger than 4 and additional game pieces are required use coins or see Manal for additional colored pieces)
- 6 species cards
- 24 island cards
- Environmental factor cards
To Begin:
- Each person in the group should draw a species stick out of the cup. You will play the role of an individual of this species for the entire game.
- Read the associated species card. This will tell you about your ecological niche and any movement limitations you will have in the game.
- Each player can place their colored game piece at any location on the mainland to start the game.
- Roll the dice. The player with the highest roll will go first and play will move counterclockwise around the group.
To Play:
1. Player 1 will roll both die. The sum of the numbers shown on the roll indicates how many squares the player can move.
Note
You cannot move diagonally but you can move vertically and horizontally and you can move forwards or backwards. Some species have limitations on the maximum number of moves they can make. If your roll exceeds the maximum number of moves for your species, you must stop at your maximum number. Every species can stop in the ocean.
2. With each roll, player 1 will also draw an environmental factor card which may influence your movement along the way. This card may affect all players, a subset of players, or just the player drawing the card.
3. If you land on any part of an island, choose the island card and determine if your species can successfully colonize the island. If so, fill that player’s name/species name in the appropriate slot on the card (remember, some islands can only hold a few species) and place a colored game piece on the island to indicate that it has been colonized by you.
Note
On the next turn, a species on an island can leave from any part of the island.
4. Play continues in a counterclockwise fashion.
5. The game is complete for an individual species when they have reached the new continent. At this point, they no longer roll the dice and are no longer affected by environmental factor cards.
6. Play continues until all species have reached the new continent.
7. Calculate the winners using the formulas below.
Who Won?
- Each player’s score is determined by adding up the total area your species covered (including bonuses) and dividing by your finish place modifier.
- 1st place = 1
- 2nd place = 1.5
- 3rd place = 2
- 4th place = 2.5
- For example: If my species covers 144 units of land and I had a bonus of 25 units of land for a total of 169 and I came in second place. My score = 122.7
\[\frac{(144\;units\;covered + 25\; bonus\;units)}{1.5\;(finish\; place\; modifier)} = 122.7\;(final \;score)\]
Questions:
1. What did the game clear up for you that you might not have understood fully about ecology and evolution?
2. Movement from the mainland to an island or from one island to another island represents what force that can cause a population to evolve?
3. If once your species has colonized an island and remains there and also becomes isolated from other populations over time, what possible outcome might occur given the passage of enough time?
4. When an island has multiple habitat types what does this say about the island’s ability to support populations of different species?
5. How suitable are small islands to support multiple species? Explain your answer.
6. Once colonizing an island, a species may thrive or fail there. Describe how a species can either thrive or fail using terms and ideas from lecture. To help you answer the question, draw how a population’s size will change over time if the species is K-selected.
Figure 1 is adapted from Wikimedia Commons material and this lab is licensed under a Creative Commons Attribution License License (3.0).