3.9: Cellular Respiration
- Last updated
- Save as PDF
- Page ID
- 30628
Bring on the S'mores!
This inviting campfire can be used for both heat and light. Heat and light are two forms of energy that are released when a fuel like wood is burned. The cells of living things also get energy by "burning." They "burn" glucose in the process called cellular respiration.
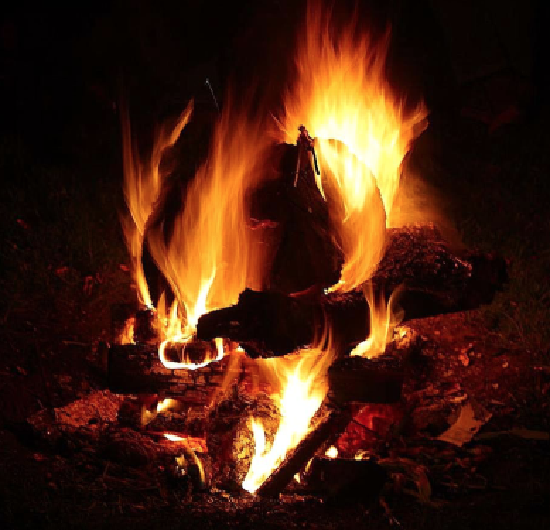
Inside every cell of all living things, energy is needed to carry out life processes. Energy is required to break down and build up molecules and to transport many molecules across plasma membranes. All of life’s work needs energy. A lot of energy is also simply lost to the environment as heat. The story of life is a story of energy flow — its capture, its change of form, its use for work, and its loss as heat. Energy, unlike matter, cannot be recycled, so organisms require a constant input of energy. Life runs on chemical energy. Where do living organisms get this chemical energy?
Where do organisms get energy from?
The chemical energy that organisms need comes from food. Food consists of organic molecules that store energy in their chemical bonds. Glucose is a simple carbohydrate with the chemical formula \(\mathrm{C_6H_{12}O_6}\). It stores chemical energy in a concentrated, stable form. In your body, glucose is the form of energy that is carried in your blood and taken up by each of your trillions of cells. Cells do cellular respiration to extract energy from the bonds of glucose and other food molecules. Cells can store the extracted energy in the form of ATP (adenosine triphosphate).
What is ATP?
Let’s take a closer look at a molecule of ATP, shown in the figure \(\PageIndex{2}\). Although it carries less energy than glucose, its structure is more complex. “A” in ATP refers to the majority of the molecule – adenosine – a combination of a nitrogenous base and a five-carbon sugar. “T” and “P” indicate the three phosphates, linked by bonds that hold the energy actually used by cells. Usually, only the outermost bond breaks to release or spend energy for cellular work.
An ATP molecule is like a rechargeable battery: its energy can be used by the cell when it breaks apart into ADP (adenosine diphosphate) and phosphate, and then the “worn-out battery” ADP can be recharged using new energy to attach a new phosphate and rebuild ATP. The materials are recyclable, but recall that energy is not! ADP can be further reduced to AMP (adenosine monophosphate and phosphate, releasing additional energy. As with ADT "recharged" to ATP, AMP can be recharged to ADP.
How much energy does it cost to do your body’s work? A single cell uses about 10 million ATP molecules per second and recycles all of its ATP molecules about every 20-30 seconds.
What Is Cellular Respiration?
Some organisms can make their own food, whereas others cannot. An autotroph is an organism that can produce its own food. The Greek roots of the word autotroph mean “self” (auto) “feeder” (troph). Plants are the best-known autotrophs, but others exist, including certain types of bacteria and algae. Oceanic algae contribute enormous quantities of food and oxygen to global food chains. Plants are also photoautotrophs, a type of autotroph that uses sunlight and carbon from carbon dioxide to synthesize chemical energy in the form of carbohydrates. Heterotrophs are organisms incapable of photosynthesis that must therefore obtain energy and carbon from food by consuming other organisms. The Greek roots of the word heterotroph mean “other” (hetero) “feeder” (troph), meaning that their food comes from other organisms. Even if the food organism is another animal, this food traces its origins back to autotrophs and the process of photosynthesis. Humans are heterotrophs, as are all animals. Heterotrophs depend on autotrophs, either directly or indirectly.
Cellular respiration is the process by which individual cells break down food molecules, such as glucose and release energy. The process is similar to burning, although it doesn’t produce light or intense heat as a campfire does. This is because cellular respiration releases the energy in glucose slowly, in many small steps. It uses the energy that is released to form molecules of ATP, the energy-carrying molecules that cells use to power biochemical processes. Cellular respiration involves many chemical reactions, but they can all be summed up with this chemical equation:
\[\ce{C6H12O6 + 6O2 -> 6CO2 + 6H2O + Energy} \nonumber\]
where the energy that is released is in chemical energy in ATP (vs. thermal energy as heat). The equation above shows that glucose (\(\ce{C6H12O6}\)) and oxygen (\(\ce{O_2}\)) react to form carbon dioxide (\(\ce{CO_2}\)) and water \(\ce{H_2O}\), releasing energy in the process. Because oxygen is required for cellular respiration, it is an aerobic process.
Cellular respiration occurs in the cells of all living things, both autotrophs and heterotrophs. All of them catabolize glucose to form ATP. The reactions of cellular respiration can be grouped into three main stages and an intermediate stage: glycolysis, Transformation of pyruvate, the Krebs cycle (also called the citric acid cycle), and Oxidative Phosphorylation. Figure \(\PageIndex{3}\) gives an overview of these three stages, which are also described in detail below.
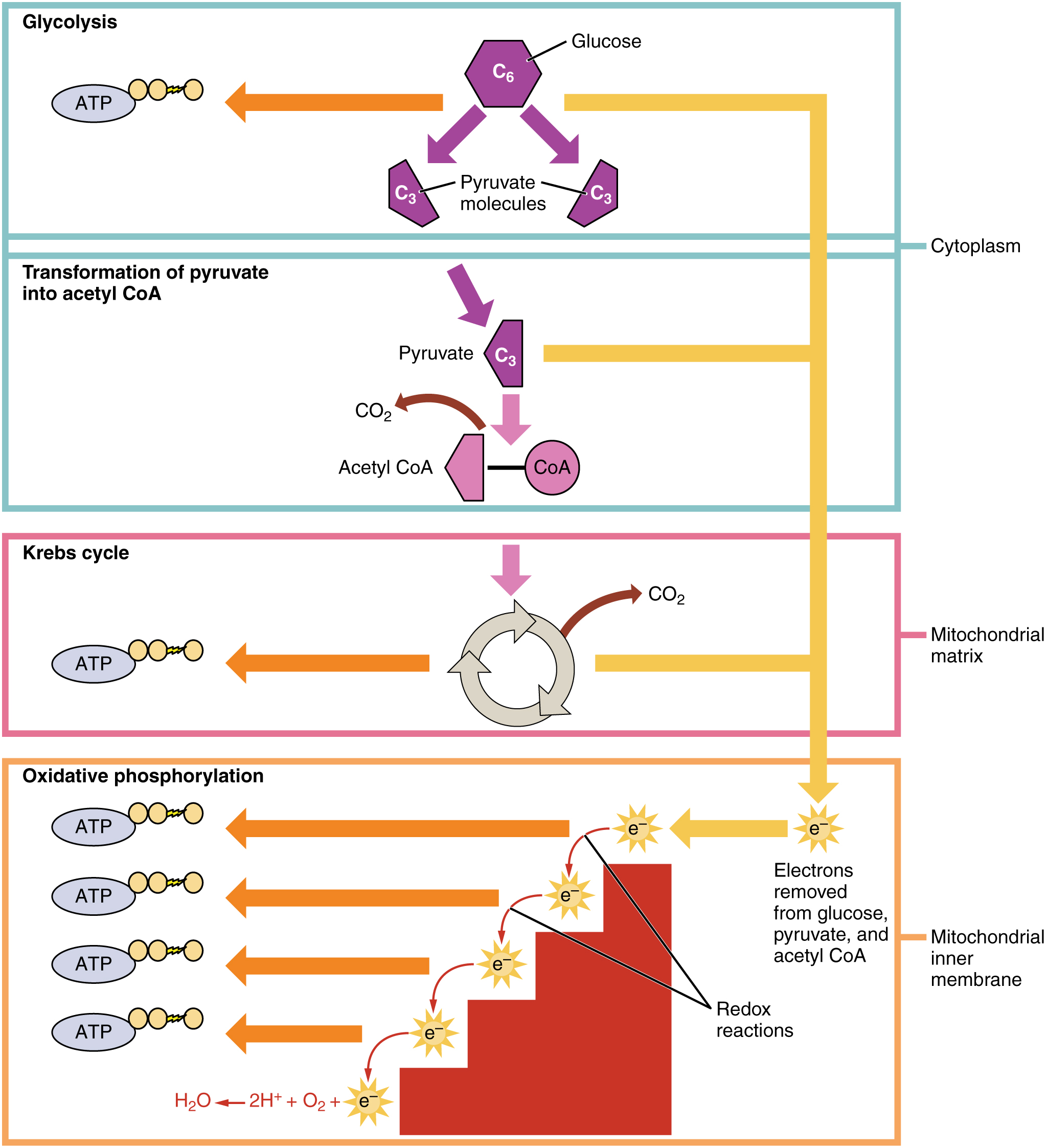
Glycolysis
The first stage of cellular respiration is glycolysis. This process is shown in the top box in Figure \(\PageIndex{3}\) showing a 6-carbon molecule being broken down into two 3-carbon pyruvate molecules. ATP is produced in this process which takes place in the cytosol of the cytoplasm.
Splitting Glucose
The word glycolysis means “glucose splitting,” which is exactly what happens in this stage. Enzymes split a molecule of glucose into two molecules of pyruvate (also known as pyruvic acid). This occurs in several steps, as shown in figure \(\PageIndex{4}\). Glucose is first split into glyceraldehyde 3-phosphate (a molecule containing 3 carbons and a phosphate group). This process uses 2 ATP. Next, each glyceraldehyde 3-phosphate is converted into pyruvate (a 3-carbon molecule). this produces two 4 ATP and 2 NADH.
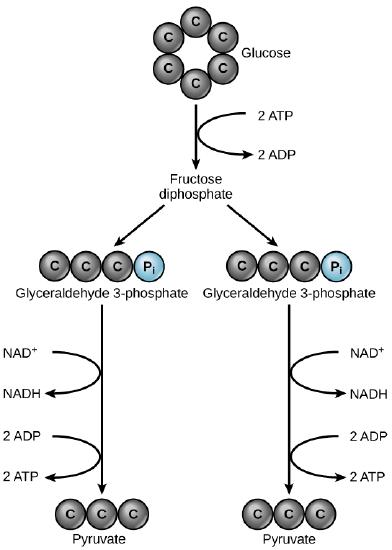
Results of Glycolysis
Energy is needed at the start of glycolysis to split the glucose molecule into two pyruvate molecules. These two molecules go on to stage II of cellular respiration. The energy to split glucose is provided by two molecules of ATP. As glycolysis proceeds, energy is released, and the energy is used to make four molecules of ATP. As a result, there is a net gain of two ATP molecules during glycolysis. high-energy electrons are also transferred to energy-carrying molecules called electron carriers through the process
known as reduction. The electron carrier of glycolysis is NAD+(nicotinamide adenine diphosphate). Electrons are transferred to 2 NAD+ to produce two molecules of NADH. The energy stored in NADH is used in stage III of cellular respiration to make more ATP. At the end of glycolysis, the following has been produced:
• 2 molecules of NADH
• 2 net molecules of ATP
Transformation of Pyruvate into Acetyl-CoA
In eukaryotic cells, the pyruvate molecules produced at the end of glycolysis are transported into mitochondria, which are sites of cellular respiration. If oxygen is available, aerobic respiration will go forward. In mitochondria, pyruvate will be transformed into a two-carbon acetyl group (by removing a molecule of carbon dioxide) that will be picked up by a carrier compound called coenzyme A (CoA), which is made from vitamin B5. The resulting compound is called acetyl CoA and its production is frequently called the oxidation or the Transformation of Pyruvate (see Figure \(\PageIndex{5}\). Acetyl CoA can be used in a variety of ways by the cell, but its major function is to deliver the acetyl group derived from pyruvate to the next pathway step, the Citric Acid Cycle.
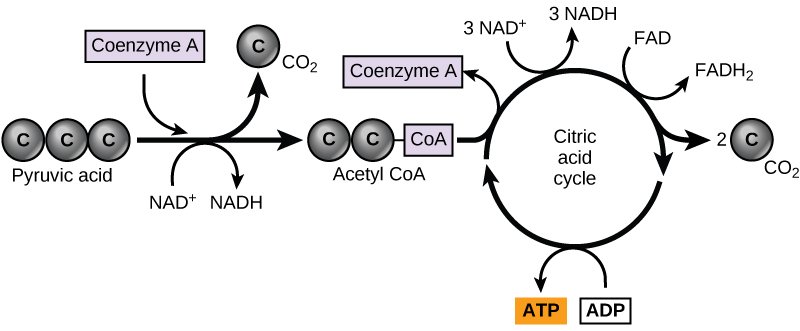
Citric Acid Cycle
Before you read about the last two stages of cellular respiration, you need to review the structure of the mitochondrion, where these two stages take place. As you can see from Figure \(\PageIndex{6}\), a mitochondrion has an inner and outer membrane. The space between the inner and outer membrane is called the intermembrane space. The space enclosed by the inner membrane is called the matrix. The second stage of cellular respiration, the Krebs cycle, takes place in the matrix. The third stage, electron transport, takes place on the inner membrane.
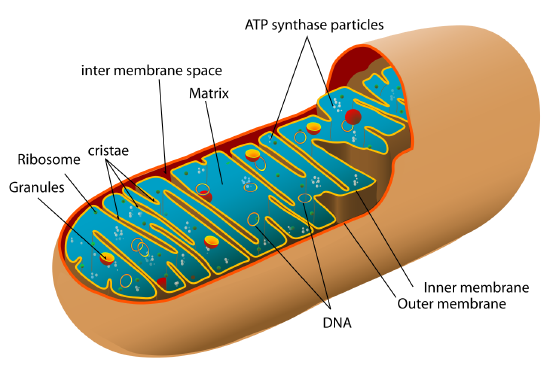
Recall that glycolysis produces two molecules of pyruvate (pyruvic acid). Pyruvate, which has three carbon atoms, is split apart and combined with CoA, which stands for coenzyme A. The product of this reaction is acetyl-CoA. These molecules enter the matrix of a mitochondrion, where they start the Citric Acid Cycle. The third carbon from pyruvate combines with oxygen to form carbon dioxide, which is released as a waste product. High-energy electrons are also released and captured in NADH. The reactions that occur next are shown in Figure \(\PageIndex{7}\).
Steps of the Citric Acid (Krebs) Cycle
The Citric Acid Cycle begins when acetyl-CoA combines with a four-carbon molecule called OAA (oxaloacetate; see the lower panel of Figure \(\PageIndex{7}\)). This produces citric acid, which has six carbon atoms. This is why the Krebs cycle is also called the citric acid cycle. After citric acid forms, it goes through a series of reactions that release energy. This energy is captured in molecules of ATP and electron carriers. The Krebs cycle has two types of energy-carrying electron carriers: NAD+ and FAD. The transfer of electrons to FAD during the Kreb’s Cycle produces a molecule of FADH2. Carbon dioxide is also released as a waste product of these reactions. The final step of the Krebs cycle regenerates OAA, the molecule that began the Krebs cycle. This molecule is needed for the next turn through the cycle. Two turns are needed because glycolysis produces two pyruvate molecules when it splits glucose.
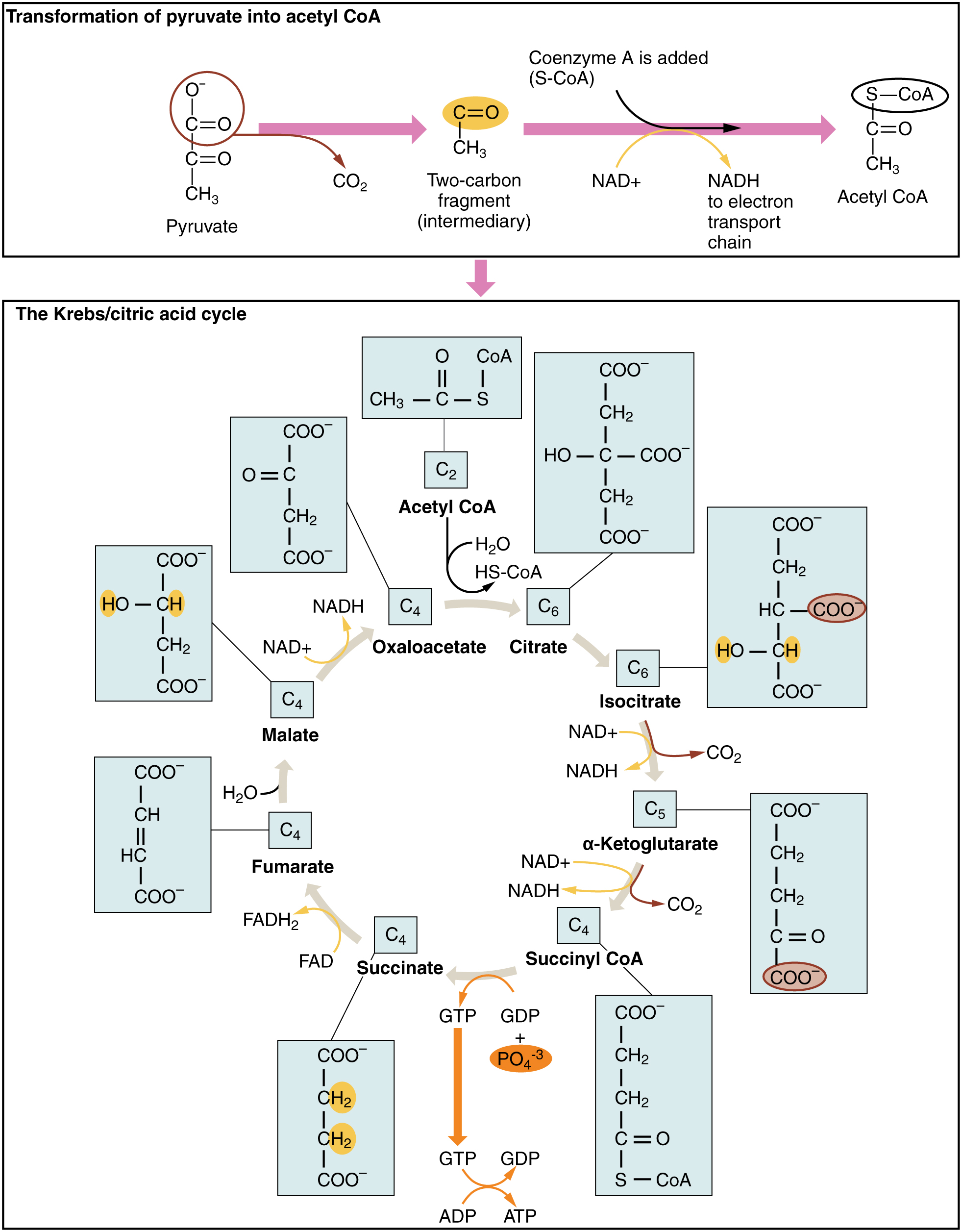
Results of the Citric Acid Cycle
After the second turn through the Citric Acid Cycle, the original glucose molecule has been broken down completely. All six of its carbon atoms have combined with oxygen to form carbon dioxide. The energy from its chemical bonds has been stored in a total of 16 energy-carrier molecules. These molecules are:
- 2 ATP
- 8 NADH
- 2 FADH\(_2\)
- 6 CO\(_2\): 2 CO\(_2\) from Transformation of Acetyl CoA and 4 CO\(_2\) from Citric Acid Cycle.
Oxidative phosphorylation
Oxidative phosphorylation is the final stage of aerobic cellular respiration. There are two substages of oxidative phosphorylation, Electron transport chain and Chemiosmosis. In these stages, energy from NADH and FADH2, which result from the previous stages of cellular respiration, is used to create ATP.

Electron Transport Chain (ETC)
During this stage, high-energy electrons are released from NADH and FADH2, and they move along electron-transport chains found in the inner membrane of the mitochondrion. An electron-transport chain is a series of molecules that transfer electrons from molecule to molecule by chemical reactions. These molecules are found making up the three complexes of the electron transport chain (red structures in the inner membrane in Figure \(\PageIndex{8}\)). As electrons flow through these molecules, some of the energy from the electrons is used to pump hydrogen ions (H+) across the inner membrane, from the matrix into the intermembrane space. This ion transfer creates an electrochemical gradient that drives the synthesis of ATP. The electrons from the final protein of the ETC are gained by the oxygen molecule, and it is reduced to water in the matrix of the mitochondrion.
Chemiosmosis
The pumping of hydrogen ions across the inner membrane creates a greater concentration of these ions in the intermembrane space than in the matrix – producing an electrochemical gradient. This gradient causes the ions to flow back across the membrane into the matrix, where their concentration is lower. The flow of these ions occurs through a protein complex, known as the ATP synthase complex (see blue structure in the inner membrane in Figure \(\PageIndex{8}\). The ATP synthase acts as a channel protein, helping the hydrogen ions across the membrane. The flow of protons through ATP synthase is considered chemiosmosis. ATP synthase also acts as an enzyme, forming ATP from ADP and inorganic phosphate. It is the flow of hydrogen ions through ATP synthase that gives the energy for ATP synthesis. After passing through the electron-transport chain, the low-energy electrons combine with oxygen to form water.
How Much ATP?
You have seen how the three stages of aerobic respiration use the energy in glucose to make ATP. How much ATP is produced in all three stages combined? Glycolysis produces 2 ATP molecules, and the Krebs cycle produces 2 more. Electron transport from the molecules of NADH and FADH2 made from glycolysis, the transformation of pyruvate, and the Krebs cycle creates as many as 32 more ATP molecules. Therefore, a total of up to 36 molecules of ATP can be made from just one molecule of glucose in the process of cellular respiration.
Review
- What is the purpose of cellular respiration? Provide a concise summary of the process.
- Draw and explain the structure of ATP (Adenosine Tri-Phosphate).
- State what happens during glycolysis.
- Describe the structure of a mitochondrion.
- Outline the steps of the Krebs cycle.
- What happens during the electron transport stage of cellular respiration?
- How many molecules of ATP can be produced from one molecule of glucose during all three stages of cellular respiration combined?
- Do plants undergo cellular respiration? Why or why not?
- Explain why the process of cellular respiration described in this section is considered aerobic.
- Name three energy-carrying molecules involved in cellular respiration.
- Energy is stored within chemical _________ within a glucose molecule.
- True or False. During cellular respiration, NADH and ATP are used to make glucose.
- True or False. ATP synthase acts as both an enzyme and a channel protein.
- True or False. The carbons from glucose end up in ATP molecules at the end of cellular respiration.
- Which stage of aerobic cellular respiration produces the most ATP?
Explore More
Watch the video below for a detailed overview of cellular respiration.
Attributions
- Campfire by Jon Sullivan, public domain via Wikimedia Commons
- ATP structure by Mysid, public domain via Wikimedia Commons
- Cellular Respiration by OpenStax College, licensed CC BY 4.0 via Wikimedia Commons
- Glycolysis by Lumen Learning, CC BY 4.0
- Citric Acid Cycle by Lumen Learning, CC BY 4.0
- Mitochondria by Mariana Ruiz Villarreal LadyofHats, released into the public domain via Wikimedia Commons
- Krebs Cycle by OpenStax College, licensed CC BY 4.0 via Wikimedia Commons
- Electron Transport Chain by OpenStax College, licensed CC BY 4.0 via Wikimedia Commons
- Text adapted from Human Biology by CK-12 licensed CC BY-NC 3.0
- Some text is adapted from Concepts of Biology by OpenStax licensed CC BY 4.0