8.3: Atmospheric and Ocean Circulation
- Last updated
- Save as PDF
- Page ID
- 94299
Atmospheric Circulation
Differential heating of the Earth’s surface results in equatorial regions receiving more heat than the poles. As air is warmed at the equator it becomes less dense and rises, while at the poles the cold air is denser and sinks. If the Earth was non-rotating, the warm air rising at the equator would reach the upper atmosphere and begin moving horizontally towards the poles. As the air reached the poles it would cool and sink, and would move over the surface of Earth back towards the equator. This would result in one large atmospheric convection cell in each hemisphere (Figure \(\PageIndex{1}\)), with air rising at the equator and sinking at the poles, and the movement of air over the Earth’s surface creating the winds. On this non-rotating Earth, the prevailing winds would thus blow from the poles towards the equator in both hemispheres (Figure \(\PageIndex{1}\)).
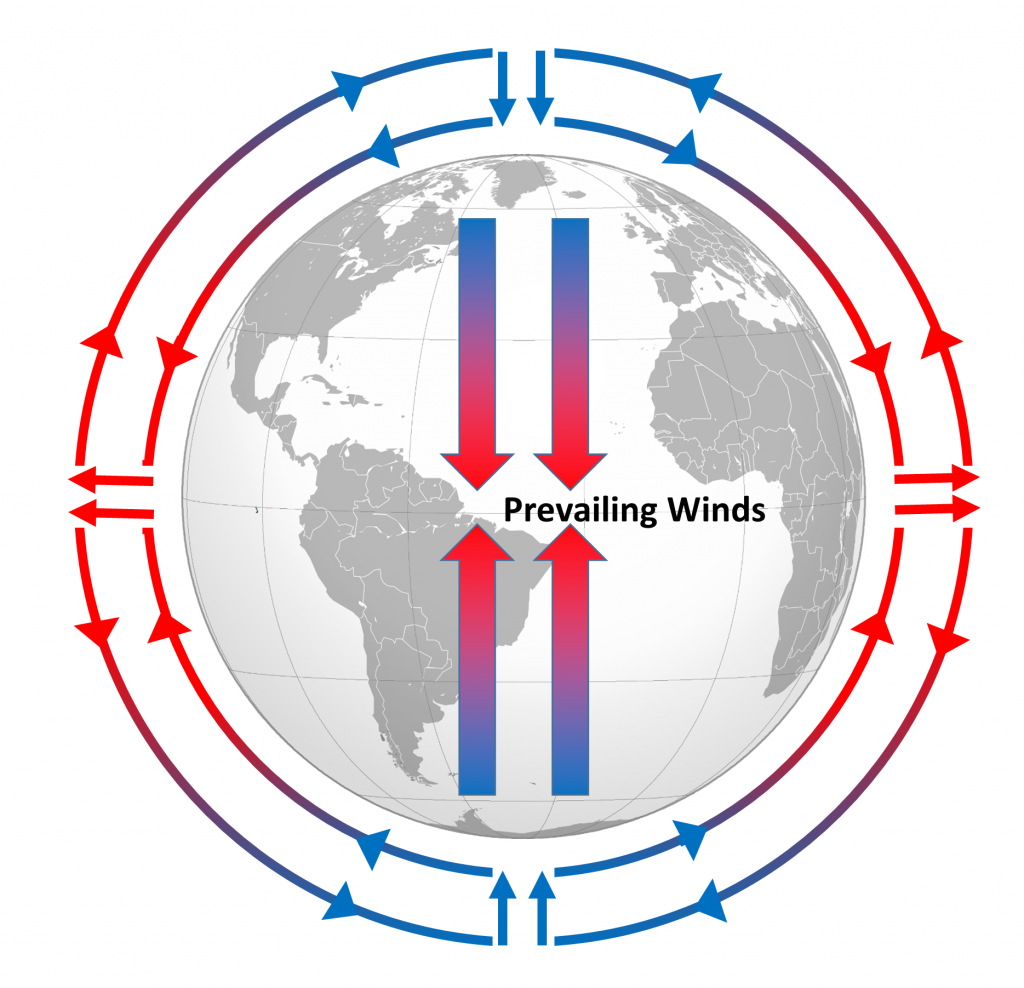
The non-rotating situation in Figure \(\PageIndex{1}\) is of course only hypothetical, and in reality the Earth’s rotation makes this atmospheric circulation a bit more complex. The paths of the winds on a rotating Earth are deflected by the Coriolis Effect. The Coriolis Effect is a result of the fact that different latitudes on Earth rotate at different speeds. This is because every point on Earth must make a complete rotation in 24 hours, but some points must travel farther, and therefore faster, to complete the rotation in the same amount of time. In 24 hours a point on the equator must complete a rotation distance equal to the circumference of the Earth, which is about 40,000 km. A point right on the poles covers no distance in that time; it just turns in a circle. So the speed of rotation at the equator is about 1600 km/hr, while at the poles the speed is 0 km/hr. Latitudes in between rotate at intermediate speeds; approximately 1400 km/hr at 30o and 800 km/hr at 60o. As objects move over the surface of the Earth they encounter regions of varying speed, which causes their path to be deflected by the Coriolis Effect.
To explain the Coriolis Effect, imagine a cannon positioned at the equator and facing north. Even though the cannon appears stationary to someone on Earth, it is in fact moving east at about 1600 km/hr due to Earth’s rotation. When the cannon fires the projectile travels north towards its target; but it also continues to move to the east at 1600 km/hr, the speed it had while it was still in the cannon. As the shell moves over higher latitudes, its momentum carries it eastward faster than the speed at which the ground beneath it is rotating. For example, by 30o latitude the shell is moving east at 1600 km/hr while the ground is moving east at only 1400 km/hr. Therefore, the shell gets “ahead” of its target, and will land to the east of its intended destination. From the point of view of the cannon, the path of the projectile appears to have been deflected to the right (red arrow, Figure \(\PageIndex{2}\)). Similarly, a cannon located at 60o and facing the equator will be moving east at 800 km/hr. When its shell is fired towards the equator, the shell will be moving east at 800 km/hr, but as it approaches the equator it will be moving over land that is traveling east faster than the projectile. So the projectile gets “behind” its target, and will land to the west of its destination. But from the point of view of the cannon facing the equator, the path of the shell still appears to have been deflected to the right (green arrow, Figure \(\PageIndex{2}\)). Therefore, in the Northern Hemisphere, the apparent Coriolis deflection will always be to the right.
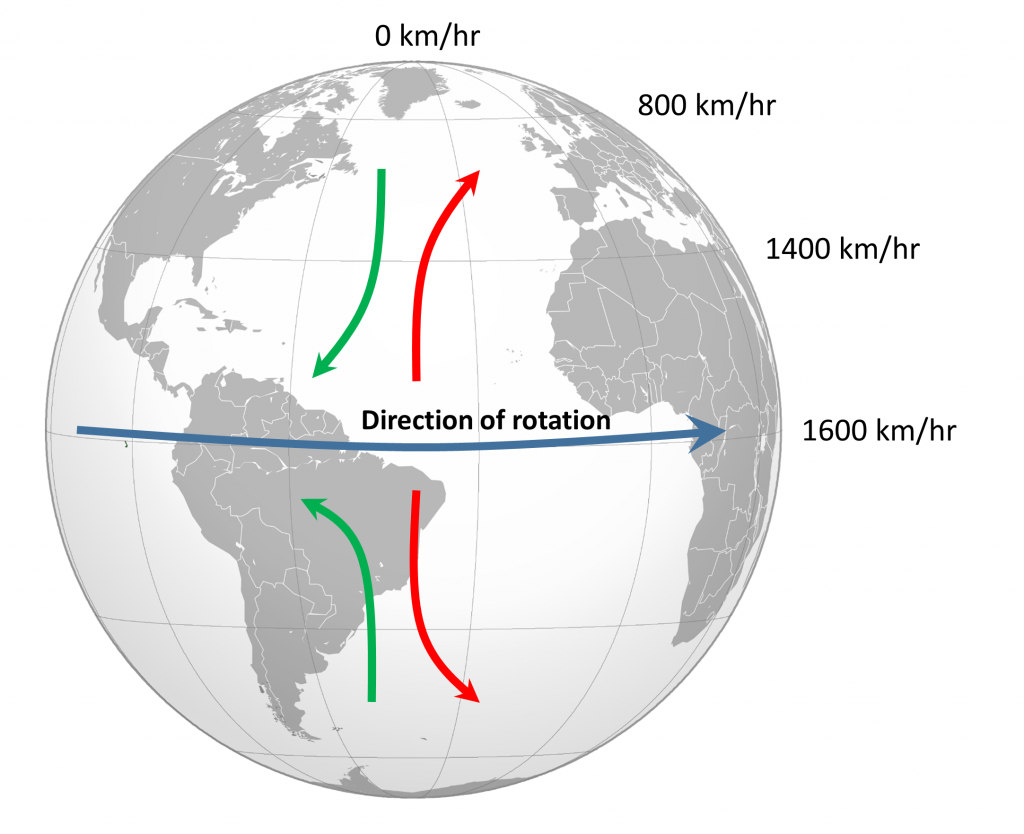
(Modified by Paul Webb from globe image by Location_of_Cape_Verde_in_the_globe.svg: Eddo derivative work: Luan fala! [CC BY-SA 3.0], via Wikimedia Commons).
In the Southern Hemisphere the situation is reversed (Figure \(\PageIndex{2}\)). Objects moving towards the equator from the south pole are moving from low speed to high speed, so are left behind and their path is deflected to the left. Movement from the equator towards the south pole also leads to deflection to the left. In the Southern Hemisphere, the Coriolis deflection is always to the left from the point of origin.
The magnitude of the Coriolis deflection is related to the difference in rotation speed between the start and end points. Between the poles and 60o latitude, the difference in rotation speed is 800 km/hr. Between the equator and 30o latitude, the difference is only 200 km/hr (Figure \(\PageIndex{2}\)). Therefore the strength of the Coriolis Effect is stronger near the poles, and weaker at the equator.
Because of the rotation of the Earth and the Coriolis Effect, rather than a single atmospheric convection cell in each hemisphere, there are three major cells per hemisphere. Warm air rising at the equator cools as it moves through the upper atmosphere, and it descends at around 30o latitude. The convection cells created by rising air at the equator and sinking air at 30o are referred to as Hadley Cells, of which there is one in each hemisphere. The cold air that descends at the poles moves over the Earth’s surface towards the equator, and by about 60o latitude it begins to rise, creating a Polar Cell between 60o and 90o. Between 30o and 60o lie the Ferrel Cells, composed of sinking air at 30o and rising air at 60o (Figure \(\PageIndex{3}\)). With three convection cells in each hemisphere that rotate in alternate directions, the surface winds no longer always blow from the poles towards the equator as in the non-rotating Earth in Figure \(\PageIndex{1}\). Instead, surface winds in both hemispheres blow towards the equator between 90o and 60o latitude, and between 0o and 30o latitude. Between 30o and 60o latitude, the surface winds blow towards the poles (Figure \(\PageIndex{3}\)).

(Modified by Paul Webb from globe image by Location_of_Cape_Verde_in_the_globe.svg: Eddo derivative work: Luan fala! [CC BY-SA 3.0], via Wikimedia Commons).
The surface winds created by the atmospheric convection cells are also influenced by the Coriolis Effect as they change latitudes. The Coriolis Effect deflects the path of the winds to the right in the Northern Hemisphere and to the left in the Southern Hemisphere. Adding this deflection leads to the pattern of prevailing winds illustrated in Figure \(\PageIndex{4}\). Between the equator and 30o latitude are the trade winds; the northeast trade winds in the Northern Hemisphere and the southeast trade winds in the Southern Hemisphere (note that winds are named based on the direction from which they originate, not where they are going). The westerlies are the dominant winds between 30o and 60o in both hemispheres, and the polar easterlies are found between 60o and the poles.

In between these wind bands lie regions of high and low pressure. High pressure zones occur where air is descending, while low pressure zones indicate rising air. Along the equator the rising air creates a low pressure region called the doldrums, or the Intertropical Convergence Zone (ITCZ) (convergence zone because this is where the trade winds converge). At 30o latitude there are high pressure zones of descending air known as the horse latitudes, or the subtropical highs. Finally, at 60o lies another low pressure region called the polar front. It should be noted that these high and low pressure zones are not fixed in place; their latitude fluctuates depending on the season, and these fluctuations have important implications for regional climates.
Doldrums, horse latitudes, and trade winds may seem like some odd names for these atmospheric phenomena, but many of them can be traced back to maritime traditions and lore. The doldrums refer to regions of low pressure around the equator. In these areas, air is rising rather than moving horizontally, so these regions commonly encounter very light winds. The lack of wind could leave sailing ships becalmed for days or weeks at a time, which was not good for the morale of the ship’s crew. Like the doldrums the horse latitudes are also areas with light winds, this time due to descending air, which could leave ships becalmed. The term trade winds may have originally derived from the terms for “track” or “path”, but the term may have become more common during European exploration and commercialization of the New World. Mariners sailing from Europe to the New World could sail south until they reached the trade winds, which would then propel their ships across the Atlantic to the Caribbean. To return to Europe, ships could sail to the northeast until they entered the westerlies, which would then steer them back to Europe.
In addition to their role in creating the surface winds, these high and low pressure systems also influence other climatic phenomena (Figure \(\PageIndex{5}\). Along the equator air is rising as it is warmed by solar radiation. Warm air contains more water vapor than cold air, which is why we experience humidity during the summer and not during the winter. The water content of air roughly doubles with every 10o C increase in temperature. So the air rising at the equator is warm and full of water vapor; as it rises into the upper atmosphere it cools, and the cool air can no longer hold as much water vapor, so the water condenses and forms rain. Therefore, low pressure systems are associated with precipitation, and we see wet habitats like tropical rainforests near the equator (Figure \(\PageIndex{5}\).
Figure \(\PageIndex{5}\): Major global climatic regions in relation to atmospheric convection cells. Rising air and low pressure creates rain and wet environments at 0o and 60o latitudes, while high pressure, sinking air creates drier conditions at 30o and 90o latitudes.
(Modified by Paul Webb; Map by Waitak at en.Wikipedia Later version(s) were uploaded by Splette at en.Wikipedia; Sun by Inductiveload (Own work Based on File:Nuvola_apps_kweather.svg); Raincloud by Calusarul (Own work); all [CC BY-SA 3.0], via Wikimedia Commons).
After rising and producing rain near the equator, the air masses move towards 30o latitude and sink back towards Earth as part of the Hadley convection cells. This air has lost most of its moisture after producing the equatorial rains, so the sinking air is dry, resulting in arid climates near 30o latitude in both hemispheres. Many of the major desert regions on Earth are located near 30o latitude, including much of Australia, the Middle East, and the Sahara Desert of Africa (Figure \(\PageIndex{5}\). The air also becomes compressed and heats up as it sinks, absorbing any moisture from the clouds and creating clear skies. Thus high pressure systems are associated with dry weather and clear skies. This cycle of high and low pressure regions continues with the Ferrel and Polar convection cells, leading to rain and the boreal forests at 60o latitude in the Northern Hemisphere (there are no corresponding large land masses at these latitudes in the Southern Hemisphere). At the poles, descending, dry air produces little precipitation, leading to the polar desert climate.
The elevation of the land also plays a role in precipitation and climactic characteristics. As moist air moves over land and encounters mountains it rises, expands, and cools because of the declining pressure and temperature. The cool air holds less water vapor, so condensation occurs and rain falls on the windward side of the mountains (Figure \(\PageIndex{6}\). As the air passes over the mountains to the leeward side, it is now dry air, and as it sinks the pressure increases, it heats back up, any moisture revaporizes, and it creates dry, deserts regions behind the mountains (Figure \(\PageIndex{6}\). This phenomenon is referred to as a rain shadow, and can be found in areas such as the Tibetan Plateau and Gobi Desert behind the Himalayas, Death Valley behind the Sierra Nevada mountains, and the dry San Joaquin Valley in California.
Figure \(\PageIndex{6}\): A rain shadow. Air rising over mountains cools and condenses and forms rain, leaving dry descending air and arid conditions on the other side of the mountain (Modified by Paul Webb from Thebiologyprimer, Public domain via Wikimedia Commons).
Ocean Circulation
Not unexpectedly, the oceans are warmest near the equator—typically 25° to 30°C—and coldest near the poles—around 0°C (Figure \(\PageIndex{7}\)). (Sea water will remain unfrozen down to about -2°C.) Variations in sea-surface temperatures (SST) are related to redistribution of water by ocean currents, as we’ll see below. A good example of that is the plume of warm Gulf Stream water that extends across the northern Atlantic. St. John’s, Newfoundland, and Brittany in France are at about the same latitude (47.5° N), but the average SST in St. John’s is a frigid 3°C, while that in Brittany is a reasonably comfortable 15°C.
.png?revision=1)
Figure \(\PageIndex{7}\): The global distribution of average annual sea-surface temperatures.
[© Plumbago. CC BY-SA. https://upload.wikimedia.org/wikiped..._TMP_AYool.png]
Currents in the open ocean are created by wind moving across the water and by density differences related to temperature and salinity. An overview of the main ocean currents is shown in Figure \(\PageIndex{8}\). As you can see, the northern hemisphere currents form circular patterns (gyres) that rotate clockwise, while the southern hemisphere gyres are counter-clockwise. This happens for the same reason that the water in your northern hemisphere sink rotates in a clockwise direction as it flows down the drain; this is caused by the Coriolis effect.

Figure \(\PageIndex{8}\): Overview of the main open-ocean currents. Red arrows represent warm water moving toward colder regions. Blue arrows represent cold water moving toward warmer regions. Black arrows represent currents that don’t involve significant temperature changes.
[“Corrientes Oceanicas” by Dr. Michael Pidwirny. Public domain. From: https://upload.wikimedia.org/wikiped...-oceanicas.png]
Because the ocean basins aren’t like bathroom basins, not all ocean currents behave the way we would expect. In the North Pacific, for example, the main current flows clockwise, but there is a secondary current in the area adjacent to our coast—the Alaska Current—that flows counter-clockwise, bringing relatively warm water from California, past Oregon, Washington, and B.C. to Alaska. On Canada’s eastern coast, the cold Labrador Current flows south past Newfoundland, bringing a stream of icebergs past the harbour at St. John’s (Figure \(\PageIndex{9}\)). This current helps to deflect the Gulf Stream toward the northeast, ensuring that Newfoundland stays cool, and western Europe stays warm.
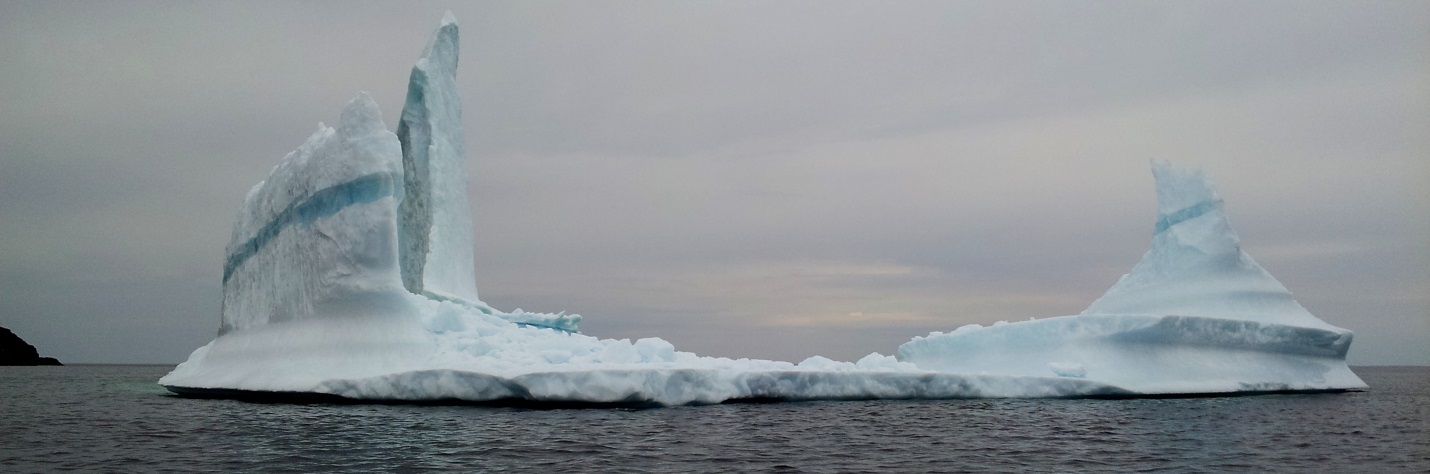
Figure \(\PageIndex{9}\): An iceberg floating past Exploits Island on the Labrador Current.
“Newfoundland Iceberg just off Exploits Island” © Shawn. CC BY-SA.

Figure \(\PageIndex{10}\): A depiction of the vertical movement of water along a north-south cross-section through the Atlantic basin.
© Steven Earle. CC BY.
The currents shown in Figure \(\PageIndex{8}\) are all surface currents, and they only involve the upper few hundred meters of the oceans. But there is much more going on underneath. The Gulf Stream, for example, which is warm and saline, flows past Britain and Iceland into the Norwegian Sea (where it becomes the Norwegian Current). As it cools down, it becomes denser, and because of its high salinity, which also contributes to its density, it starts to sink beneath the surrounding water (Figure \(\PageIndex{10}\)). At this point, it is known as North Atlantic Deep Water (NADW), and it flows to significant depth in the Atlantic as it heads back south. Meanwhile, at the southern extreme of the Atlantic, very cold water adjacent to Antarctica also sinks to the bottom to become Antarctic Bottom Water (AABW) which flows to the north, underneath the NADW.
The descent of the dense NADW is just one part of a global system of seawater circulation, both at surface and at depth, as illustrated in Figure \(\PageIndex{11}\). The water that sinks in the areas of deep water formation in the Norwegian Sea and adjacent to Antarctica moves very slowly at depth. It eventually resurfaces in the Indian Ocean between Africa and India, and in the Pacific Ocean, north of the equator.

Figure \(\PageIndex{11}\): The thermohaline circulation system, also known as the Global Ocean Conveyor.
[“Thermohaline Circulation” by NASA. Public domain from: https://en.Wikipedia.org/wiki/Thermo...culation_2.png]
The thermohaline circulation is critically important to the transfer of heat on Earth. It brings warm water from the tropics to the poles, and cold water from the poles to the tropics, thus keeping polar regions from getting too cold and tropical regions from getting too hot. A reduction in the rate of thermohaline circulation would lead to colder conditions and enhanced formation of sea ice at the poles. This would start a positive feedback process that could result in significant global cooling. There is compelling evidence to indicate that there were major changes in thermohaline circulation, corresponding with climate changes, during the Pleistocene Glaciation.
Contributors and Attributions
- Kyle Whittinghill (University of Pittsburgh)
- Contributed by Paul Webb Professor (Biology) at Rodger Williams University
- Contributed by Steven Earle Faculty (Geology) at Vancover Island University
- 18.4 Ocean Water from Physical Geology by Steven Earle (licensed under CC-BY)
- 8.2 Winds and the Coriolis Effect from Introduction to Oceanography by Paul Webb (licensed under CC-BY)
- 8.3 Winds and Climate from Introduction to Oceanography by Paul Webb (licensed under CC-BY)