3.2: Cell Division and Movement: Cleavage - Frog Gastrulation and the Dorsal Lip of the Blastopore
- Page ID
- 21529
Cleavage
Cleavage stages of embryogenesis are typically very similar across animals. During cleavage a single cell embryo rapidly divides to form a ball of cells, called a blastula. This ball may be hollow inside, have a hollow region, or be fairly solid. Early cleavage stages are different from later cell divisions in that they quickly produce many very similar looking cells. If morphogens are asymmetrically distributed in the egg, then these cells can differentiate via autonomous specification even at early stages. Conditional specification typically begins to occur towards the end of cleavage stages when cell division slows down and cells begin to communicate more extensively with each other. Sometimes this is called the "mid-blastula transition" or MBT. The MBT demarcates the transition between using mostly maternal cell and fate determinants (RNAs and protein) to the embryo producing its own RNAs and proteins. One interesting experiment that shows the extent of this transition is to block transcription using the drugs alpha-amanitin or actinomycin D - two inhibitors of RNA polymerase action. If these drugs are applied to an early cleavage-stage embryo cleavages proceed normally, suggesting that developmental processes and cell division at this early stage are using maternally-derived factors already present in the embryo. If the drugs are applied to later-stage embryos development halts, suggesting that at this point zygotically derived transcripts are necessary for development and cell division. The timing of the MBT and the sorting of maternal proteins and RNAs into different cleavage-stage cells varies widely among different animals.
Read more details about Cleavage here, focus on any section you find interesting and especially figure 8.5. www.ncbi.nlm.nih.gov/books/NBK9992/#_A1678_
Gastrulation
Gastrulation refers to a time of great flux in animal embryos. During gastrulation the cells produced during cleavage begin to move relative to each other. As they move, they change their local environment and come into contact with new cells. This change in local environment means that the cells may a) be exposed to different extracellular morphogens and b) make contact with new cell-cell signaling partners. The movement of cells (Figure 3) over (epiboly), under (involution), between (intercalation), internally (invagination) or away from an epithelial sheet (ingression and delamination) creates layers of tissue. As the cells comprising these tissue layers encounter new morphogens and new signaling partners, they become specified as germ-layers.
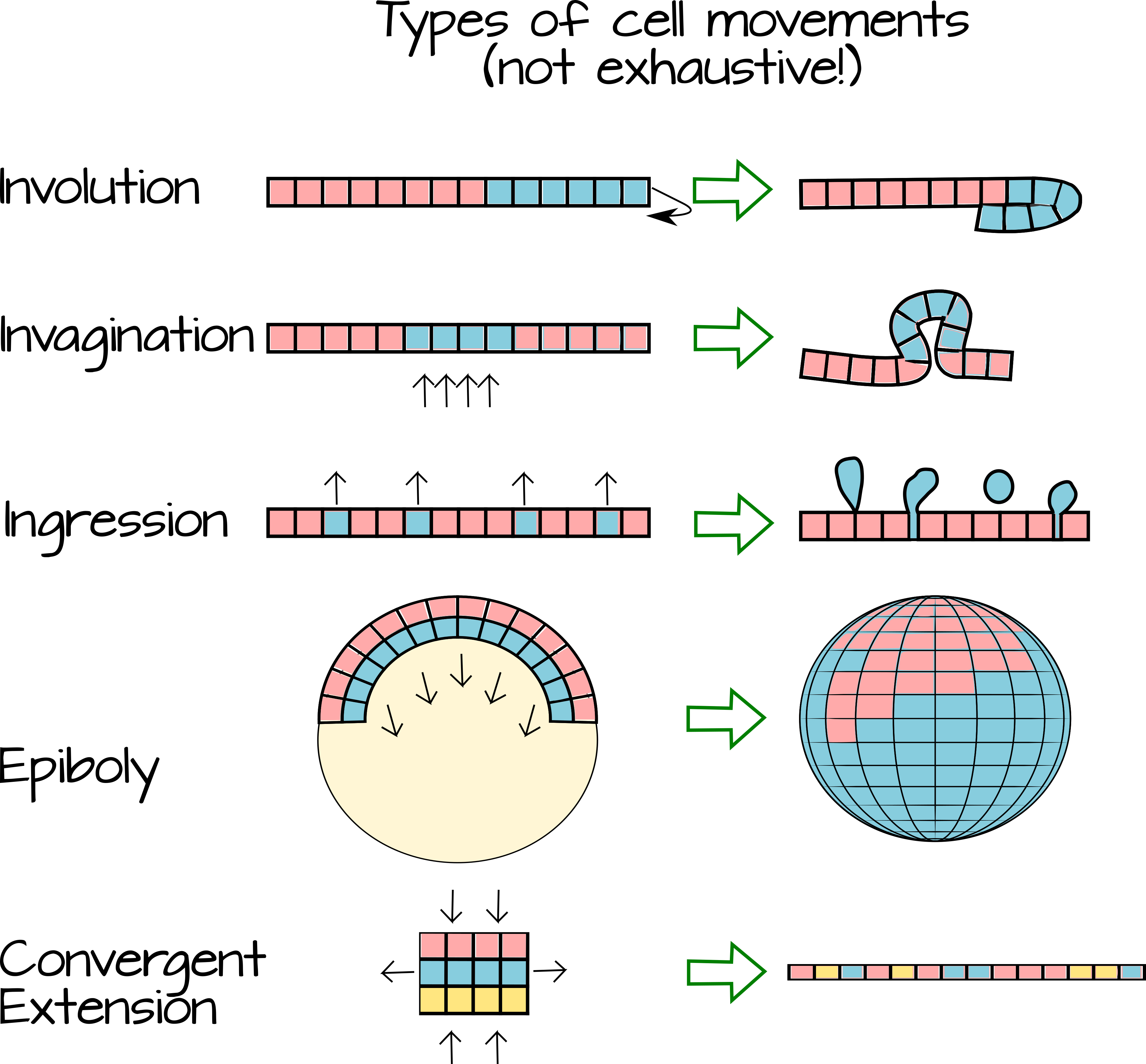
The following video shows a live-frog embryo undergoing cleavage and gastrulation and also shows an animated internal view of how the cells move inside the embryo. Note the different kinds of movements. https://www.youtube.com/watch?v=riSA1mo86Kg
Changing Partners
As mentioned earlier, gastrulation performs two key functions in a developing animal embryo. First, it creates tissue layers (germ layers) from set of fairly homogenous cells. Second, it allows for new interactions between cells and places cells in new morphogenic positions. As cells change their partners over time, they become fated to a specific path. For example, compare a picture of a fate-map of a blastula-stage frog to the fate map of a gastrulated frog (Figure 4).
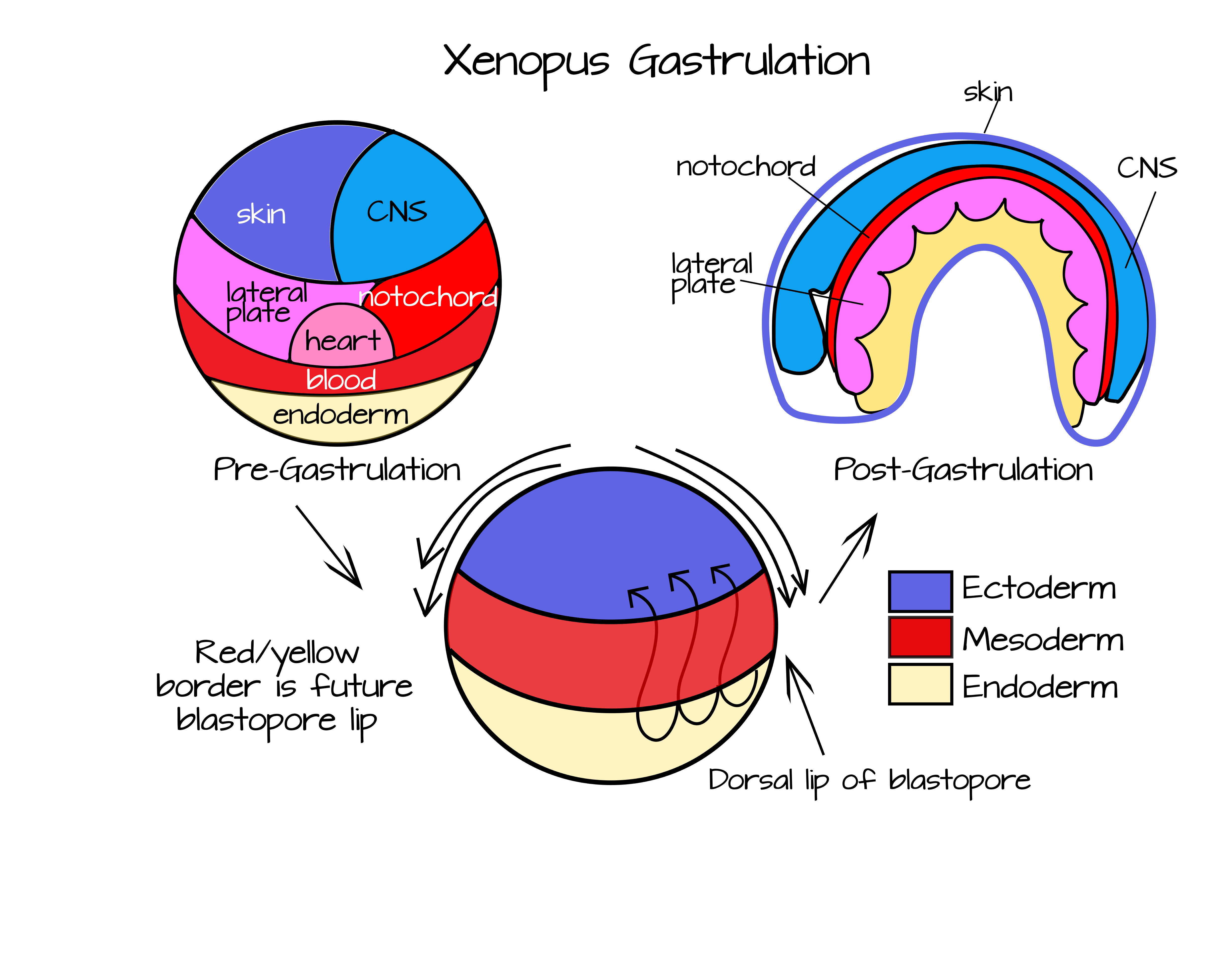
While the blastula-stage frog seems almost random in its mapping, the gastrulated frog has all of its tissues/organs in roughly adult positions. The blastula stage has not undergone extensive cell movements yet and the cells are largely unspecified. If cells are removed during the blastula stage, other cells will often be able to take on the fate of the missing cells. For example, identical twins develop from a single embryo that has "split" into two after multiple cell divisions. Rather than each half of the embryo making only half an animal when contact is broken between the two cell masses they each develop into an entire animal. One of my favorite sets of experiments of all time, the Spemann-Mangold organizer experiments, shows the extent to which cell-cell communication can rule development. Before I discuss that I would like to briefly summarize frog gastrulation (no offense to other animals, but they are my favorite gastrulators). A more in-depth look at frog gastrulation can be found here: www.ncbi.nlm.nih.gov/books/NBK10113/ and an interactive fate-map for Xenopus is available from https://www.xenbase.org/anatomy/static/xenbasefate.jsp
Frog Gastrulation and the Dorsal lip of the Blastopore
After fertilization and cortical rotation, the frog embryo is divided roughly into four quadrants. These are demarcated by the two major axes A/V (animal-vegetal) and D/V (dorsoventral). There is no Anteroposterior axis yet, this axis will be generated during gastrulation. https://www.youtube.com/watch?v=EPMgHMnwW28
Over the course of gastrulation, the red area (future mesoderm) in Figure 5 will involute under the blue area (future ectoderm) and extend to form a layer at the roof of the blastocoel (Figures 3 and 4). This involution occurs most extensively at the dorsal side of the embryo (the side opposite sperm entry), but also occurs along the rest of the "blastopore lip". To visualize the blastopore lip, open your mouth wide. Your lips represent mesoderm and the rest of your face represents ectoderm (sorry no endoderm in this one!). Use your lips to cover your teeth. This is physically similar to involution. Now imagine that your lips could grow thin and long and cover the inside of your mouth. This is how the mesodermal cells end up as a second layer under the ectoderm. Finally, begin to close your mouth. This is similar to epiboly - when the ectodermal cells move over the surface of the embryo to cover the mesoderm and endoderm.
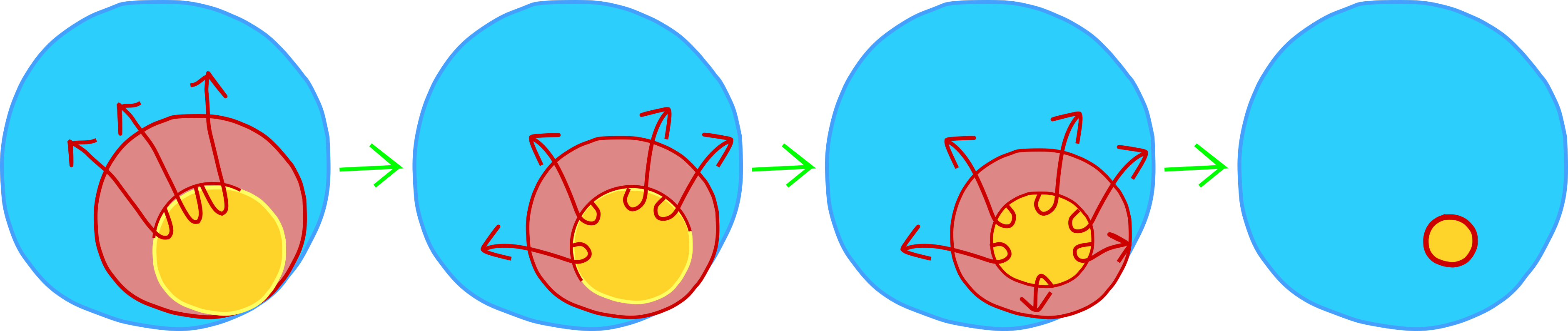
To understand the role of the Spemann-Mangold organizer, you need to keep these movements in mind. In particular, you need to think about the dorsal lip of the blastopore, the place where involution first begins and happens most extensively. If you look at the Figure 4 fate map, you will notice that the dorsal mesoderm (the mesoderm at the dorsal lip of the blastopore) will become the notochord. These cells are the first mesodermal to internalize during epiboly. This block of cells will elongate and thin out to become a rod of mesodermal tissue that spans the length of the embryo. This will run along the anteroposterior axis, just like your spinal column runs along your anterioposterior axis. Additionally, protein signals from the notochord will help to pattern the rest of the embryo, as Hans Spemann and Hilde Mangold famously discovered.