4.2.1: Tropisms
- Page ID
- 31996
Learning Objectives
- Distinguish among phototropism, gravitropism, hydrotropism, and thigmotropism.
- Discuss the adaptive value of tropisms.
- Describe the mechanism of phototropism in shoots.
- Describe the mechanism of gravitropism in shoots and roots.
- Distinguish among thigmotropism, thigmonastic movements, and thigmomorphogenesis.
A tropism is directional growth in response to a stimulus. A positive tropism occurs when a plant (or a part of the plant) grows towards the stimulus, and a negative tropism is growth away from the stimulus.
Phototropism is directional growth in response to light (Figure \(\PageIndex{1}\)). (More generally, photomorphogenesis is the growth and development of plants in response to light.) Stems are positively phototropic, and roots are typically negatively phototropic. Gravitropism is directional growth in response to gravity. Stems are negatively gravitropic, and roots are positively gravitropic. The adaptive value of these tropisms is clear. Stems growing upward and/or toward the light will be able to expose their leaves so that photosynthesis can occur. Roots growing downward and/or away from light are more likely to find the soil, water, and minerals they need. Plants can also grow directionally in response to water (hydrotropism) and touch (thigmotropism).
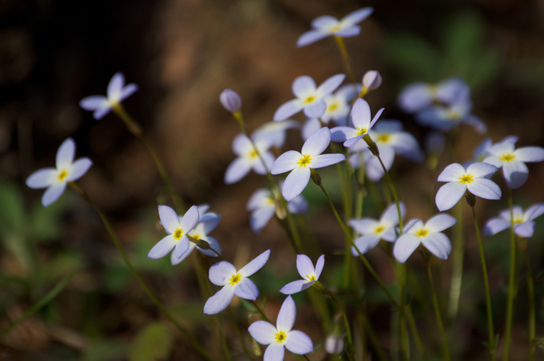
Phototropism in Shoots
Plants can detect different characteristics of light, such as quantity, quality, duration, and direction. They can tell the time of day and time of year by sensing and using various wavelengths of sunlight. Plants are generally capable of detecting and responding to at least three wavelengths of light: blue light, red light, and far-red light. The different wavelengths are detected by different photoreceptors (Figure \(\PageIndex{2}\)), an example of which are phototropins.

The Shoot Tip Detects Light and Induces Phototropism
In their 1880 treatise The Power of Movements in Plants, Charles Darwin and his son Francis first described phototropism as the bending of seedlings toward light. The Darwins used grass seedlings for some of their experiments. When grass seeds germinate, the primary leaf pierces the seed coverings and the soil while protected by the coleoptile, a hollow, cylindrical sheath that surrounds it (Figure \(\PageIndex{3}\) ).

Once the seedling has grown above the surface, the coleoptile stops growing and the primary leaf pierces it. If they placed an opaque cover over the tip, phototropism failed to occur even though the rest of the coleoptile was illuminated from one side. However, when they buried the plant in fine black sand so that only its tip was exposed, there was no interference with the tropism — the buried coleoptile bent in the direction of the light (Figure \(\PageIndex{4}\) ). In conclusion, light was perceived by the tip of the plant (the apical meristem), but that the response (bending) took place in a different part of the plant. The signal had to travel from the apical meristem to the base of the plant.
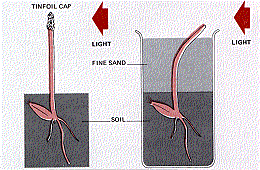
We now know that phototropism is a response to blue wavelengths of light. The detection of light in the apical meristem occurs via phototropins called phot1 and phot2, which specifically detect blue light (Figure \(\PageIndex{5}\) ). The aptly-named phototropins are protein-based receptors responsible for mediating the phototropic response. Like all plant photoreceptors, phototropins consist of a protein portion and a light-absorbing portion, called the chromophore. Together, the two are called a chromoprotein. In phototropins, the chromophore is a covalently-bound molecule of flavin; hence, phototropins belong to a class of proteins called flavoproteins.
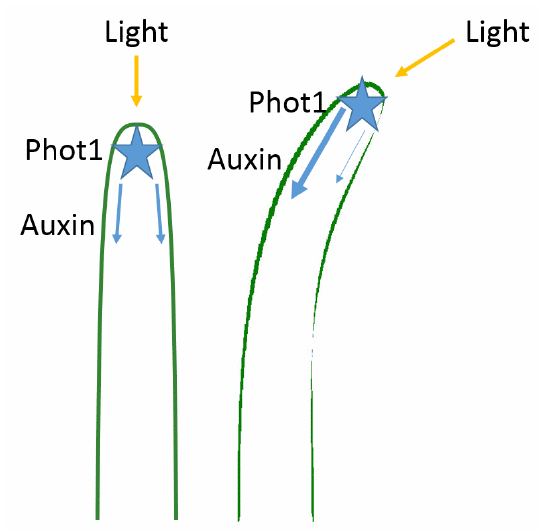
The Hormone Auxin from the Shoot Tip Stimulates Elongation on the Shaded Side of the Stem
In 1913, the Danish plant physiologist Peter Boysen-Jensen demonstrated that a chemical signal produced in the plant tip was responsible for the bending at the base. He cut off the tip of a seedling, covered the cut section with a layer of gelatin, and then replaced the tip. The seedling bent toward the light when illuminated. However, when impermeable mica flakes were inserted between the tip and the cut base, the seedling did not bend. A refinement of the experiment showed that the signal traveled on the shaded side of the seedling. When the mica plate was inserted on the illuminated side, the plant did bend towards the light. When a horizontal incision was made on the illuminated side and the mica inserted in it, phototropism was normal (Figure \(\PageIndex{6}\) ). Therefore, the chemical signal was a growth stimulant because the phototropic response involved faster cell elongation on the shaded side than on the illuminated side.
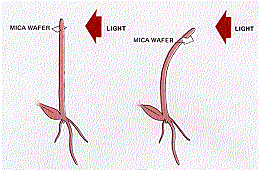
We now know that auxin is the chemical signal that accumulates and stimulates elongation on shaded side of coleoptile. H. W. Went placed a coleoptile that has previously been illuminated from one side on two separated agar blocks. The block on the side that had been shaded accumulated almost twice as much auxin as the block on the previously lighted side (Figure \(\PageIndex{7}\) ).

Auxin stimulates cell elongation on the shady side of the stem through a process called the acid growth hypothesis: Auxin causes cells to activate proton pumps, which then pump protons out of the cells and into the space between the plasma membrane and the cell wall. The movement of protons into the extracellular space does two things:
- The lower pH activates expansin, which breaks the links between the cellulose fibers in the cell walls, making them more flexible.
- The high concentration of protons causes sugars to move into the cell, which then creates an osmotic gradient where water moves into cell causing the cell to expand.
Phototropism in Roots
Although roots are underground, they can be exposed to light directly as well. Not only can light penetrate up to a few centimeters in the upper layers of some soils, the plant itself can also guide light through the stem to the roots. Additionally, roots can also be exposed to light shortly after germination in the top layer of the soil or because cracks in the soil emerge that trigger a phototropic reaction. Negative phototropism in roots may be evolutionarily advantageous because it increases root efficiency and enhances seedling survival under dry conditions. As in shoots, phototropin 1 is involved in root phototropism. Directional auxin transport and an auxin gradient are associated with root phototropsim, but it is unclear whether they mediate the process.
Gravitropism
Whether or not they germinate in the light or in total darkness, shoots usually sprout up from the ground, and roots grow downward into the ground. A plant laid on its side in the dark will send shoots upward when given enough time. Gravitropism also involves the unequal distribution of auxin.
When an oat coleoptile tip is placed on two separated agar blocks, as shown here, there is no difference in the auxin activity picked up by the two blocks. When the preparation is placed on its side, however, the lower block accumulates twice as much auxin activity as the upper block. Under natural conditions, this would cause greater cell elongation on the underside of the coleoptile and the plant would bend upward (Figure \(\PageIndex{8}\)).

Specialized amyloplasts called statoliths settle downward in response to gravity. Statoliths are found in the inner part of a stem's cortex (the starch sheath) and in the the central column of the root cap (columella). When a plant is tilted, the statoliths drop to the new bottom cell wall. A few hours later, the shoot or root will show growth in the new vertical direction (Figure \(\PageIndex{9}\)).
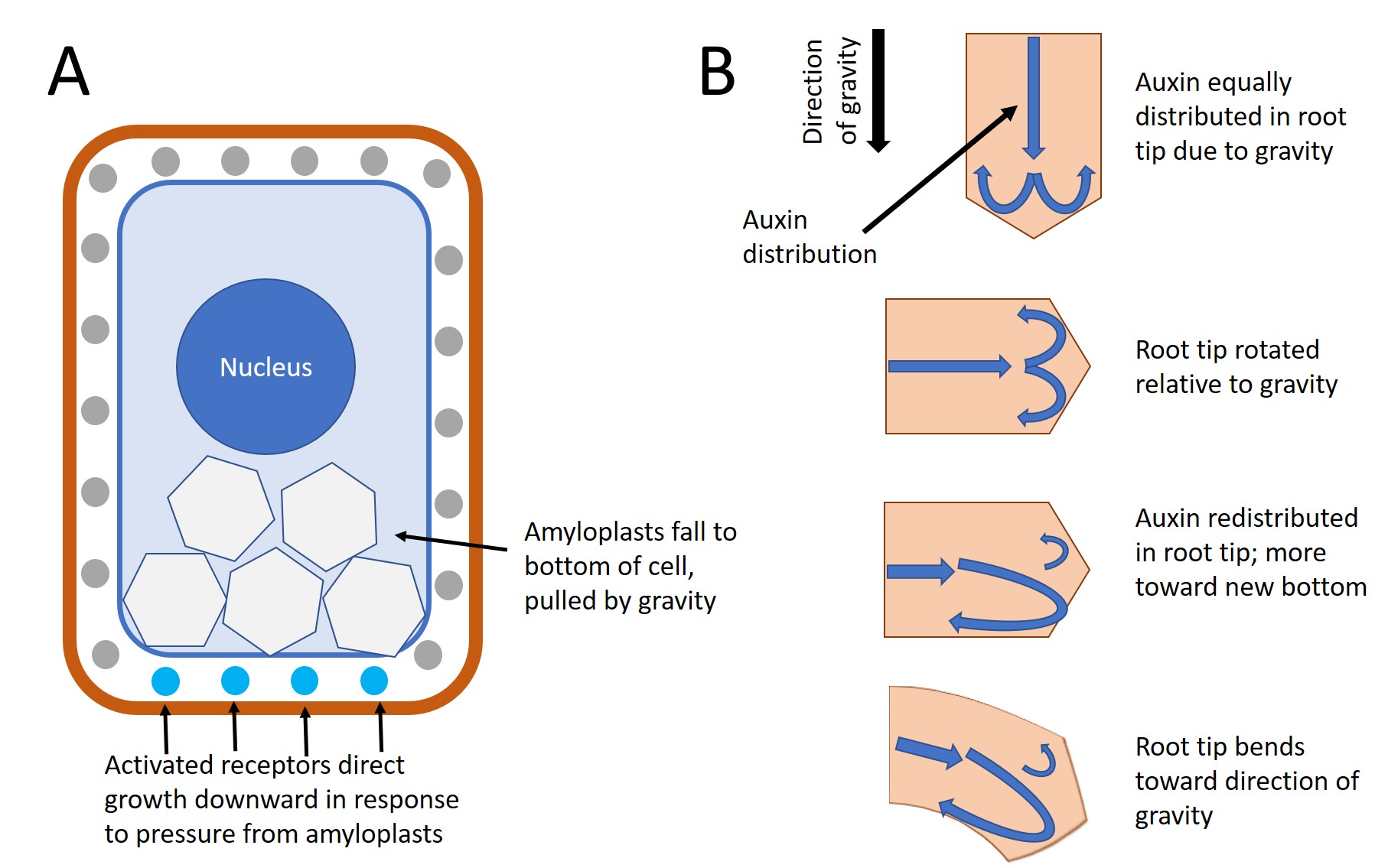
The mechanism that mediates gravitropism is reasonably well understood. When amyloplasts settle to the bottom of the gravity-sensing cells in the root or shoot, they physically contact the endoplasmic reticulum (ER), causing the release of calcium ions from inside the ER. Calcium may be released by ion channels sensitive to mechanical stimulation. This calcium signaling in the cells causes auxin transport proteins (PIN proteins) to redistribute to the underside of the cell leading to the polar transport of auxin to the bottom of the cell. In roots, a high concentration of indoleacetic acid (IAA) inhibits cell elongation. The effect slows growth on the lower side of the root, while cells develop normally on the upper side. IAA has the opposite effect in shoots, where a higher concentration at the lower side of the shoot stimulates cell expansion, causing the shoot to grow up. After the shoot or root begin to grow vertically, the amyloplasts return to their normal position. Another hypotheses—involving the entire cell in the gravitropism effect—have been proposed to explain why some mutants that lack amyloplasts may still exhibit a weak gravitropic response.
Plant Tropisms in Space
Considering the fundamental role of plants in producing fresh food and recycling of air and water, plant tropism research is critical for advancing plant-based life support systems in space. Understanding of the relative strengths of the different root tropisms would be needed to properly guide root growth by technological means, as the gravity vector is absent in space. For example, by exposing roots in microgravity to blue light, they could be induced to develop away from light toward the growth medium. Furthermore, the possibility of performing explorative experiments in the space environment, together with the development of new technologies, is crucial to pave the way toward the goal of deepening our fundamental understanding of plant tropisms and their underlying molecular networks on Earth.
Modified by Melissa Ha from Muthert, L., Izzo, L. G., van Zanten, M., & Aronne, G. (2020). Root Tropisms: Investigations on Earth and in Space to Unravel Plant Growth Direction. Frontiers in plant science, 10, 1807. https://doi.org/10.3389/fpls.2019.01807. CC BY
Hydrotropism
Water acquisition is an important function of plant roots. Because water availability in the soil is often spatially and temporally patchy, roots of many species can exert directional root growth toward water; i.e., positive hydrotropism. The underlying mechanisms of hydrotropism are still being researched. Gravitropism is often dominant over hydrotropic responses, making it difficult to study hydrotropism in isolation. In contrast to phototropism and gravitropism, hydrotropism does not result from an auxin gradient resulting from directional auxin transport. However, auxin still appears to play a role in signaling hydrotropism.
Thigmotropism, Thigmomorphogensis, and Thigmonastic Movements
The shoot of a pea plant winds around a trellis while a tree grows on an angle in response to strong prevailing winds. These are examples of how plants respond to touch or wind.
The movement of a plant subjected to constant directional pressure is called thigmotropism, from the Greek words thigma meaning “touch,” and tropism implying “direction.” Tendrils are one example of plants displaying positive thigmotropism (Video \(\PageIndex{1}\)). The meristematic region of tendrils is very touch sensitive; light touch will evoke a quick coiling response. Cells in contact with a support surface contract, whereas cells on the opposite side of the support expand. Application of jasmonic acid is sufficient to trigger tendril coiling without a mechanical stimulus.
Video \(\PageIndex{1}\): This video shows an example of positive thigmotropism in morning glory plants, which require a support structure of some type to grow optimally. The time lapse images were taken at 10 minute intervals. This video has no sound. Here is a description: The morning glory stems (which are modified as tendrils) grow in a counterclockwise pattern, widening their radius with each rotation. When they encounter one of the two wooden stakes on either side of the plant, grow in a tight coil around it.
Plant roots exhibit negative thigmotropism when contacting obstacles in the soil. When plant roots encounter an obstacle in their growth path, the root first continues growing in the same direction until it slips sideways. The root bends away from the stimulus and then bends again in the opposite direction, creating a step-like shape. Note that at this point the root "side stepped" the obstacle and is now continuing to grow in the original direction. If the root contacts another part of the obstacle, the same bending pattern will occur. Thigmotropism in roots may be mediated by calcium, but other signaling mechanisms have also been proposed. Following contact, the calcium concentration in the root cap is greater than that of surrounding regions. (Under regular conditions, calcium concentration in the root cap is lower.) Ultimately, the directional transport of auxin results in an auxin gradient. When touching an obstacle during downward growth, the root bends and auxin accumulates at the higher (concave) side of the root suppressing elongation. Greater elongation on the convex (lower) side of the root causes it to bend away from the obstacle.
Thigmomorphogenesis is a slow developmental change in the shape of a plant subjected to continuous mechanical stress. When trees bend in the wind, for example, growth is usually stunted and the trunk thickens. Strengthening tissue, especially xylem, is produced to add stiffness to resist the wind’s force. Researchers hypothesize that mechanical strain induces growth and differentiation to strengthen the tissues. Ethylene and jasmonate are likely involved in thigmomorphogenesis.
A thigmonastic movement is a touch response independent of the direction of stimulus. In the Venus flytrap, two modified leaves are joined at a hinge and lined with thin fork-like tines along the outer edges (Video \(\PageIndex{2}\)). Tiny hairs are located inside the trap. When an insect brushes against these trigger hairs, touching two or more of them in succession, the leaves close quickly, trapping the prey. Glands on the leaf surface secrete enzymes that slowly digest the insect. The released nutrients are absorbed by the leaves, which reopen for the next meal.
Video \(\PageIndex{2}\): This video below shows an example of thigmonastic movements in a Venus flytrap. There is no only ambient sound in this video and no speaking. Here is a description. At 0:08, a fly enters the taco-shaped trap of the Venus flytrap. It escapes before the tines (prongs) interlock, as shown in a slow-motion replay. At 0:42, another fly crawls into the trap. It crawls around a bit before the trap closes, locking it inside.
Another example of thigmonastic movement occurs in the sensitive plant (Mimosa pudica). Its leaflets and leaves retract in response to touch, and this is thought to be an adaptation that deters herbivores (Video \(\PageIndex{3}\)).
Video \(\PageIndex{3}\): The leaflets of the sensitive plant retract when touched. This video has no speaking, but there are natural sounds (such as birds) in the background and laughing when the leaflets retract.
Attributions
Curated and authored by Melissa Ha using the following sources:
- 16.2F: Tropisms from Biology by John. W. Kimball (licensed CC-BY)
- 30.6 Plant Sensory Systems and Responses from Biology 2e by OpenStax (licensed CC-BY). Access for free at openstax.org.
- Plant Hormones and Sensory Systems by Biology 1520 Introduction to Organismal Biology (licensed CC-BY-NC-SA)
- Muthert, L., Izzo, L. G., van Zanten, M., & Aronne, G. (2020). Root Tropisms: Investigations on Earth and in Space to Unravel Plant Growth Direction. Frontiers in plant science, 10, 1807. https://doi.org/10.3389/fpls.2019.01807. (licensed CC-BY)