3.2: Pathways and Complementation Analysis
- Page ID
- 142348
\( \newcommand{\vecs}[1]{\overset { \scriptstyle \rightharpoonup} {\mathbf{#1}} } \)
\( \newcommand{\vecd}[1]{\overset{-\!-\!\rightharpoonup}{\vphantom{a}\smash {#1}}} \)
\( \newcommand{\id}{\mathrm{id}}\) \( \newcommand{\Span}{\mathrm{span}}\)
( \newcommand{\kernel}{\mathrm{null}\,}\) \( \newcommand{\range}{\mathrm{range}\,}\)
\( \newcommand{\RealPart}{\mathrm{Re}}\) \( \newcommand{\ImaginaryPart}{\mathrm{Im}}\)
\( \newcommand{\Argument}{\mathrm{Arg}}\) \( \newcommand{\norm}[1]{\| #1 \|}\)
\( \newcommand{\inner}[2]{\langle #1, #2 \rangle}\)
\( \newcommand{\Span}{\mathrm{span}}\)
\( \newcommand{\id}{\mathrm{id}}\)
\( \newcommand{\Span}{\mathrm{span}}\)
\( \newcommand{\kernel}{\mathrm{null}\,}\)
\( \newcommand{\range}{\mathrm{range}\,}\)
\( \newcommand{\RealPart}{\mathrm{Re}}\)
\( \newcommand{\ImaginaryPart}{\mathrm{Im}}\)
\( \newcommand{\Argument}{\mathrm{Arg}}\)
\( \newcommand{\norm}[1]{\| #1 \|}\)
\( \newcommand{\inner}[2]{\langle #1, #2 \rangle}\)
\( \newcommand{\Span}{\mathrm{span}}\) \( \newcommand{\AA}{\unicode[.8,0]{x212B}}\)
\( \newcommand{\vectorA}[1]{\vec{#1}} % arrow\)
\( \newcommand{\vectorAt}[1]{\vec{\text{#1}}} % arrow\)
\( \newcommand{\vectorB}[1]{\overset { \scriptstyle \rightharpoonup} {\mathbf{#1}} } \)
\( \newcommand{\vectorC}[1]{\textbf{#1}} \)
\( \newcommand{\vectorD}[1]{\overrightarrow{#1}} \)
\( \newcommand{\vectorDt}[1]{\overrightarrow{\text{#1}}} \)
\( \newcommand{\vectE}[1]{\overset{-\!-\!\rightharpoonup}{\vphantom{a}\smash{\mathbf {#1}}}} \)
\( \newcommand{\vecs}[1]{\overset { \scriptstyle \rightharpoonup} {\mathbf{#1}} } \)
\( \newcommand{\vecd}[1]{\overset{-\!-\!\rightharpoonup}{\vphantom{a}\smash {#1}}} \)
\(\newcommand{\avec}{\mathbf a}\) \(\newcommand{\bvec}{\mathbf b}\) \(\newcommand{\cvec}{\mathbf c}\) \(\newcommand{\dvec}{\mathbf d}\) \(\newcommand{\dtil}{\widetilde{\mathbf d}}\) \(\newcommand{\evec}{\mathbf e}\) \(\newcommand{\fvec}{\mathbf f}\) \(\newcommand{\nvec}{\mathbf n}\) \(\newcommand{\pvec}{\mathbf p}\) \(\newcommand{\qvec}{\mathbf q}\) \(\newcommand{\svec}{\mathbf s}\) \(\newcommand{\tvec}{\mathbf t}\) \(\newcommand{\uvec}{\mathbf u}\) \(\newcommand{\vvec}{\mathbf v}\) \(\newcommand{\wvec}{\mathbf w}\) \(\newcommand{\xvec}{\mathbf x}\) \(\newcommand{\yvec}{\mathbf y}\) \(\newcommand{\zvec}{\mathbf z}\) \(\newcommand{\rvec}{\mathbf r}\) \(\newcommand{\mvec}{\mathbf m}\) \(\newcommand{\zerovec}{\mathbf 0}\) \(\newcommand{\onevec}{\mathbf 1}\) \(\newcommand{\real}{\mathbb R}\) \(\newcommand{\twovec}[2]{\left[\begin{array}{r}#1 \\ #2 \end{array}\right]}\) \(\newcommand{\ctwovec}[2]{\left[\begin{array}{c}#1 \\ #2 \end{array}\right]}\) \(\newcommand{\threevec}[3]{\left[\begin{array}{r}#1 \\ #2 \\ #3 \end{array}\right]}\) \(\newcommand{\cthreevec}[3]{\left[\begin{array}{c}#1 \\ #2 \\ #3 \end{array}\right]}\) \(\newcommand{\fourvec}[4]{\left[\begin{array}{r}#1 \\ #2 \\ #3 \\ #4 \end{array}\right]}\) \(\newcommand{\cfourvec}[4]{\left[\begin{array}{c}#1 \\ #2 \\ #3 \\ #4 \end{array}\right]}\) \(\newcommand{\fivevec}[5]{\left[\begin{array}{r}#1 \\ #2 \\ #3 \\ #4 \\ #5 \\ \end{array}\right]}\) \(\newcommand{\cfivevec}[5]{\left[\begin{array}{c}#1 \\ #2 \\ #3 \\ #4 \\ #5 \\ \end{array}\right]}\) \(\newcommand{\mattwo}[4]{\left[\begin{array}{rr}#1 \amp #2 \\ #3 \amp #4 \\ \end{array}\right]}\) \(\newcommand{\laspan}[1]{\text{Span}\{#1\}}\) \(\newcommand{\bcal}{\cal B}\) \(\newcommand{\ccal}{\cal C}\) \(\newcommand{\scal}{\cal S}\) \(\newcommand{\wcal}{\cal W}\) \(\newcommand{\ecal}{\cal E}\) \(\newcommand{\coords}[2]{\left\{#1\right\}_{#2}}\) \(\newcommand{\gray}[1]{\color{gray}{#1}}\) \(\newcommand{\lgray}[1]{\color{lightgray}{#1}}\) \(\newcommand{\rank}{\operatorname{rank}}\) \(\newcommand{\row}{\text{Row}}\) \(\newcommand{\col}{\text{Col}}\) \(\renewcommand{\row}{\text{Row}}\) \(\newcommand{\nul}{\text{Nul}}\) \(\newcommand{\var}{\text{Var}}\) \(\newcommand{\corr}{\text{corr}}\) \(\newcommand{\len}[1]{\left|#1\right|}\) \(\newcommand{\bbar}{\overline{\bvec}}\) \(\newcommand{\bhat}{\widehat{\bvec}}\) \(\newcommand{\bperp}{\bvec^\perp}\) \(\newcommand{\xhat}{\widehat{\xvec}}\) \(\newcommand{\vhat}{\widehat{\vvec}}\) \(\newcommand{\uhat}{\widehat{\uvec}}\) \(\newcommand{\what}{\widehat{\wvec}}\) \(\newcommand{\Sighat}{\widehat{\Sigma}}\) \(\newcommand{\lt}{<}\) \(\newcommand{\gt}{>}\) \(\newcommand{\amp}{&}\) \(\definecolor{fillinmathshade}{gray}{0.9}\)Pathway Analysis: The "One Gene, One Enzyme" Hypothesis
Once you've performed a screen and recovered mutants, you can begin to use the tools of genetics, cell biology and biochemistry to understand how the mutated genes are involved in the biological process you're interested in. How can you begin to use those mutants to understand the genetic basis of the biological process?
There are two key insights for the practical use of forward genetics. The first, as we've already seen, is epistasis -- the fact that multiple genes are likely involved in any process, and the effect of the alleles of one locus often depend on the alleles at other loci. For example, a flower may get its color from a compound produced by two enzymes in a biosynthetic pathway (see the figure below.) Mutating either of the genes will result in the pigment not being produced.

Synthesis of even a relatively simple molecule requires many steps, each with a different enzyme. Each enzyme works sequentially on a different intermediate in the pathway. For example, the genes involved in synthesizing the amino acid arginine were identified in Neurospora crassa using a screen where the progeny of a mutagenized spore could grow on minimal medium only when it was supplemented with arginine (Arg). (Such strains that can only grow with supplements like this are called auxotrophs.)Thus, the mutant strain must bear a mutation in the Arg biosynthetic pathway and was called an “arginineless” strain (arg-).

Our screen has told us that genes A, B and C are necessary to synthesize arginine -- but what order are they in in the biosynthetic pathway? To answer this question, we can use a method called pathway analysis (or sometimes genetic analysis). For arginine (Arg), two biochemical intermediates are ornithine (Orn) and citrulline (Cit). Thus, mutation of any one of the enzymes in this pathway could turn Neurospora into an Arg auxotroph (arg-). Srb and Horowitz extended their analysis of Arg auxotrophs by testing the intermediates of amino acid biosynthesis for the ability to restore growth of the mutants (Figure 5.4.3).

They found that only Arg could rescue all the Arg auxotrophs, while either Arg or Cit could rescue some (Table 5.4.1). Based on these results, they deduced the location of each mutation in the Arg biochemical pathway, (i.e., which gene was responsible for the metabolism of which intermediate).
Mutants in: | MM + Orn | MM + Cit | MM + Arg |
---|---|---|---|
Gene A | Yes | Yes | Yes |
Gene B | No | Yes | Yes |
Gene C | No | No | Yes |
Complementation Analysis
If epistasis is the first key to using forward genetics, the second is this: you may have isolated more mutants than there are genes in the pathway. Geneticists often observe two independently derived mutants with similar phenotypes, through a mutant screen or in natural populations. Is mutant phenotype is due to a loss of function in the same gene, or are they mutant in different genes that both cause the same phenotype (e.g., in the same pathway)? In other words, are they allelic mutations or non-allelic mutations, respectively? This question can be resolved using complementation tests, which bring together the two mutations into the same organism to assess the combined phenotype.
The easiest way to understand a complementation test is by example in the figure below. Let's assume, as above, that the purple flower pigment is due to a ciochemical pathway involving two genes. A diploid plant that lacks the function of gene A (genotype aa) would produce mutant white flowers that phenotypically looked just like the white flowers of a plant that lacked the function of gene B (genotype bb). Both A and B are enzymes in the same pathway that leads from a colourless compound #1, through colourless compound #2, to the purple pigment. Blocks at either step will result in a mutant white flower instead of the wild type purple flower.
If these two strains are crossed together the resulting progeny will all be AaBb. They will have both a wild type, functional A gene and B gene and will thus have a pigmented, purple flower, a wild type phenotype. This is an example of complementation. Together, each strain provides what the other is lacking (AaBb). The mutations are in different genes and are thus called non-allelic mutations.
Now, if we are presented with a third pure-breeding, independently derived, white-flower, mutant strain, we won’t initially know if it is mutant in gene A, gene B, or some other gene altogether. We can use complementation testing to determine which gene is mutated. To perform a complementation test, two homozygous individuals with similar mutant phenotypes are crossed.
If the F1 progeny all have the same mutant phenotype (Case 1), then we infer that the same gene is mutated in each parent. These mutations would then be called allelic mutations — mutant in the same gene locus. These two mutations FAIL to COMPLEMENT one another (still mutant). These could either be exactly the same mutant alleles (same base pair changes), or different mutations (different base pair changes, but in the same gene — allelic).
Conversely, if the F1 progeny all appear to be wild type (Case 2), then each of the parents most likely carries a mutation in a different gene. These mutations would then be called non-allelic mutations — mutant in a different gene locus. These mutations DO COMPLEMENT one another.


Note: For mutations to be used in complementation tests they are (1) usually true-breeding (homozygous at the mutant locus), and (2) must be recessive mutations. Dominant and semi-dominant mutations CANNOT be used in complementation tests, since these mutations won’t show complementation effects of two non-allelic genes.
Extending the Complementation Test
So, with the third mutant strain above, we could assign it to be allelic with either gene A or gene B, or some other locus, should it complement both gene A and gene B mutations. If they came from different natural populations or from independently mutagenized individuals, we could have a fourth, fifth, sixth, etc. white flower strain, then we could begin to organize the allelic mutations into groups, which are called complementation groups. These are groups of mutations that FAIL TO COMPLEMENT one another (a group of NON-complementing mutations) and are assumed to have mutations in the SAME gene; hence they are grouped as complementation group. A group can consist of as few as one mutation and as many as all the mutants under study. Each group represents a set of mutations in the same gene (allelic). The number of complementation groups represents the number of genes that are represented in the total collection of mutations.
It all depends on how many mutations you have in a gene. For example, the white gene in Drosophila has >300 different mutations within the white gene described in the literature. If you were to obtain and cross all these mutations to themselves, you would find they all belonged to the same complementation group or same white gene. Each complementation group represents a gene.
If, however, you obtained a different mutation, vestigial for example, which affects wing growth, and crossed it to a white eye-colour mutation, the double heterozygote would result in red eyes and normal wings (wild type for both characters) so the two would complement and represent two different complementation groups: (1) white, (2) vestigial. The same would be true for the other eye-colour mutations mentioned elsewhere in this text. For example, if you crossed a scarlet eye-colour mutant to a white eye-colour mutant, the double heterozygote would have wild type red eyes. Each mutant has the wild type allele of the other. Again, remember that all the other genes in the diploid genome are assumed to be wild type.
To drive home the concept of complementation groups, we will look at two hypothetical examples.
Example 1: Multiple Mutant Complementation Test
The first example shows the results of a series of crosses as a complementation test table with six mutants labelled a to f. The mutants fall into three complementation groups in total: (1) a (2) b, c, f, and (3) d, e. Notice that a complementation group can consist of only one mutant, or more than one.

Example 2: Double Hit Strain
The second example is similar, but has a twist. It has five mutants labelled 1-5, with 1-4 being mutations in only a single gene each, while mutant #5 has mutations in two different genes, and thus is unable to complement the mutations in two, different genes. A double-hit strain like strain #5 is normally a very rare event, but is included here to make a point. A double-hit strain may appear to belong in two different groups. In this case, mutants #3 and #4 complement (different genes) but #5 fails to complement both #3 and #4, indicating it has mutations in both the mutant genes in #3 (gene B) and #4 (gene C).

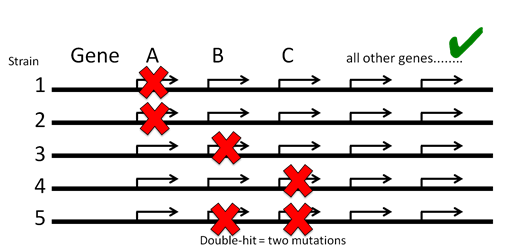