5.4: Protists
- Page ID
- 82996
\( \newcommand{\vecs}[1]{\overset { \scriptstyle \rightharpoonup} {\mathbf{#1}} } \)
\( \newcommand{\vecd}[1]{\overset{-\!-\!\rightharpoonup}{\vphantom{a}\smash {#1}}} \)
\( \newcommand{\id}{\mathrm{id}}\) \( \newcommand{\Span}{\mathrm{span}}\)
( \newcommand{\kernel}{\mathrm{null}\,}\) \( \newcommand{\range}{\mathrm{range}\,}\)
\( \newcommand{\RealPart}{\mathrm{Re}}\) \( \newcommand{\ImaginaryPart}{\mathrm{Im}}\)
\( \newcommand{\Argument}{\mathrm{Arg}}\) \( \newcommand{\norm}[1]{\| #1 \|}\)
\( \newcommand{\inner}[2]{\langle #1, #2 \rangle}\)
\( \newcommand{\Span}{\mathrm{span}}\)
\( \newcommand{\id}{\mathrm{id}}\)
\( \newcommand{\Span}{\mathrm{span}}\)
\( \newcommand{\kernel}{\mathrm{null}\,}\)
\( \newcommand{\range}{\mathrm{range}\,}\)
\( \newcommand{\RealPart}{\mathrm{Re}}\)
\( \newcommand{\ImaginaryPart}{\mathrm{Im}}\)
\( \newcommand{\Argument}{\mathrm{Arg}}\)
\( \newcommand{\norm}[1]{\| #1 \|}\)
\( \newcommand{\inner}[2]{\langle #1, #2 \rangle}\)
\( \newcommand{\Span}{\mathrm{span}}\) \( \newcommand{\AA}{\unicode[.8,0]{x212B}}\)
\( \newcommand{\vectorA}[1]{\vec{#1}} % arrow\)
\( \newcommand{\vectorAt}[1]{\vec{\text{#1}}} % arrow\)
\( \newcommand{\vectorB}[1]{\overset { \scriptstyle \rightharpoonup} {\mathbf{#1}} } \)
\( \newcommand{\vectorC}[1]{\textbf{#1}} \)
\( \newcommand{\vectorD}[1]{\overrightarrow{#1}} \)
\( \newcommand{\vectorDt}[1]{\overrightarrow{\text{#1}}} \)
\( \newcommand{\vectE}[1]{\overset{-\!-\!\rightharpoonup}{\vphantom{a}\smash{\mathbf {#1}}}} \)
\( \newcommand{\vecs}[1]{\overset { \scriptstyle \rightharpoonup} {\mathbf{#1}} } \)
\( \newcommand{\vecd}[1]{\overset{-\!-\!\rightharpoonup}{\vphantom{a}\smash {#1}}} \)
\(\newcommand{\avec}{\mathbf a}\) \(\newcommand{\bvec}{\mathbf b}\) \(\newcommand{\cvec}{\mathbf c}\) \(\newcommand{\dvec}{\mathbf d}\) \(\newcommand{\dtil}{\widetilde{\mathbf d}}\) \(\newcommand{\evec}{\mathbf e}\) \(\newcommand{\fvec}{\mathbf f}\) \(\newcommand{\nvec}{\mathbf n}\) \(\newcommand{\pvec}{\mathbf p}\) \(\newcommand{\qvec}{\mathbf q}\) \(\newcommand{\svec}{\mathbf s}\) \(\newcommand{\tvec}{\mathbf t}\) \(\newcommand{\uvec}{\mathbf u}\) \(\newcommand{\vvec}{\mathbf v}\) \(\newcommand{\wvec}{\mathbf w}\) \(\newcommand{\xvec}{\mathbf x}\) \(\newcommand{\yvec}{\mathbf y}\) \(\newcommand{\zvec}{\mathbf z}\) \(\newcommand{\rvec}{\mathbf r}\) \(\newcommand{\mvec}{\mathbf m}\) \(\newcommand{\zerovec}{\mathbf 0}\) \(\newcommand{\onevec}{\mathbf 1}\) \(\newcommand{\real}{\mathbb R}\) \(\newcommand{\twovec}[2]{\left[\begin{array}{r}#1 \\ #2 \end{array}\right]}\) \(\newcommand{\ctwovec}[2]{\left[\begin{array}{c}#1 \\ #2 \end{array}\right]}\) \(\newcommand{\threevec}[3]{\left[\begin{array}{r}#1 \\ #2 \\ #3 \end{array}\right]}\) \(\newcommand{\cthreevec}[3]{\left[\begin{array}{c}#1 \\ #2 \\ #3 \end{array}\right]}\) \(\newcommand{\fourvec}[4]{\left[\begin{array}{r}#1 \\ #2 \\ #3 \\ #4 \end{array}\right]}\) \(\newcommand{\cfourvec}[4]{\left[\begin{array}{c}#1 \\ #2 \\ #3 \\ #4 \end{array}\right]}\) \(\newcommand{\fivevec}[5]{\left[\begin{array}{r}#1 \\ #2 \\ #3 \\ #4 \\ #5 \\ \end{array}\right]}\) \(\newcommand{\cfivevec}[5]{\left[\begin{array}{c}#1 \\ #2 \\ #3 \\ #4 \\ #5 \\ \end{array}\right]}\) \(\newcommand{\mattwo}[4]{\left[\begin{array}{rr}#1 \amp #2 \\ #3 \amp #4 \\ \end{array}\right]}\) \(\newcommand{\laspan}[1]{\text{Span}\{#1\}}\) \(\newcommand{\bcal}{\cal B}\) \(\newcommand{\ccal}{\cal C}\) \(\newcommand{\scal}{\cal S}\) \(\newcommand{\wcal}{\cal W}\) \(\newcommand{\ecal}{\cal E}\) \(\newcommand{\coords}[2]{\left\{#1\right\}_{#2}}\) \(\newcommand{\gray}[1]{\color{gray}{#1}}\) \(\newcommand{\lgray}[1]{\color{lightgray}{#1}}\) \(\newcommand{\rank}{\operatorname{rank}}\) \(\newcommand{\row}{\text{Row}}\) \(\newcommand{\col}{\text{Col}}\) \(\renewcommand{\row}{\text{Row}}\) \(\newcommand{\nul}{\text{Nul}}\) \(\newcommand{\var}{\text{Var}}\) \(\newcommand{\corr}{\text{corr}}\) \(\newcommand{\len}[1]{\left|#1\right|}\) \(\newcommand{\bbar}{\overline{\bvec}}\) \(\newcommand{\bhat}{\widehat{\bvec}}\) \(\newcommand{\bperp}{\bvec^\perp}\) \(\newcommand{\xhat}{\widehat{\xvec}}\) \(\newcommand{\vhat}{\widehat{\vvec}}\) \(\newcommand{\uhat}{\widehat{\uvec}}\) \(\newcommand{\what}{\widehat{\wvec}}\) \(\newcommand{\Sighat}{\widehat{\Sigma}}\) \(\newcommand{\lt}{<}\) \(\newcommand{\gt}{>}\) \(\newcommand{\amp}{&}\) \(\definecolor{fillinmathshade}{gray}{0.9}\)Protists
- Please read and watch the following Learning Resources
- Reading the material for understanding, and taking notes during videos, will take approximately 3 hours.
- Optional Activities are embedded.
- To navigate to the next section, use the Contents menu at the top of the page OR the right arrow on the side of the page.
- If on a mobile device, use the Contents menu at the top of the page OR the links at the bottom of the page.
- Describe the cell structure characteristics of protists
- Describe the metabolic diversity of protists
- Describe the life cycle diversity of protists
- Describe representative protist organisms from each of the six presently recognized supergroups of eukaryotes
- Identify the evolutionary relationships of plants, animals, and fungi within the six presently recognized supergroups of eukaryotes
Introduction
Humans have been familiar with macroscopic organisms (organisms big enough to see with the unaided eye) since before there was written history, and it is likely that most cultures distinguished between animals and land plants, and most probably included the macroscopic fungi as plants. Therefore, it became an interesting challenge to deal with the world of microorganisms once microscopes were developed a few centuries ago. Many different naming schemes were used over the last couple of centuries, but it has become the most common practice to refer to eukaryotes that are not land plants, animals, or fungi as protists.
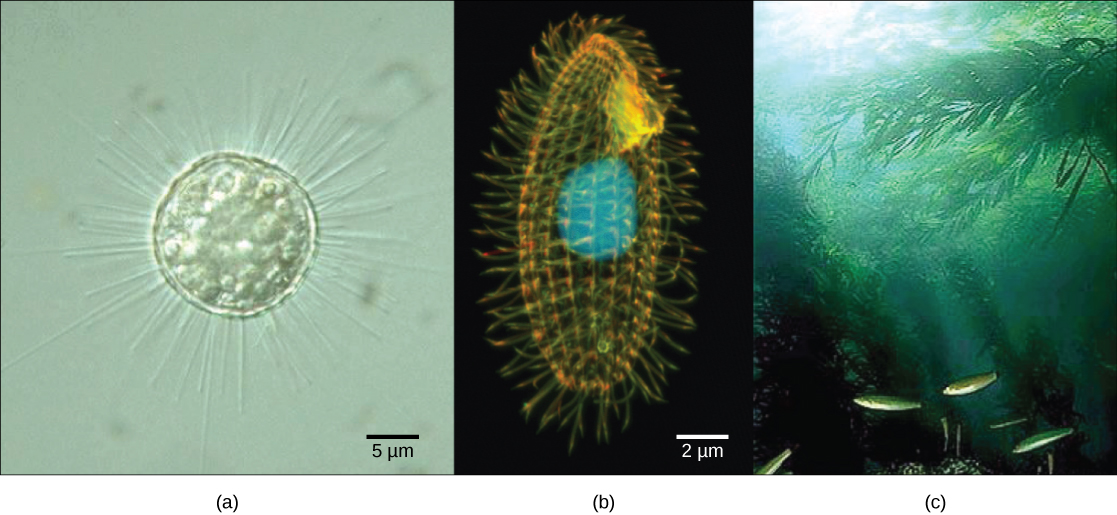
The name protist was first suggested by Ernst Haeckel in the late nineteenth century. It has been applied in many contexts and has been formally used to represent a kingdom-level taxon called Protista. Many modern systematists (biologists who study the relationships among organisms) are naming taxa as groups of organisms thought to include all the descendants of a LCA (monophyletic group). During the past two decades, the field of molecular genetics has demonstrated that some protists are more related to animals, plants, or fungi than they are to other protists. Therefore, not including animals, plants and fungi make the kingdom Protista a paraphyletic group, or one that does not include all descendants of its common ancestor Figure \(\PageIndex{1}\). For this reason, protist lineages originally classified into the kingdom Protista continue to be examined and debated. In the meantime, the term “protist” is still used informally to describe this diverse group of eukaryotes.
This 4-minute video, explore the many varied types of protists and their evolutionary history.
Question after watching: Why are all three types of algae placed in Protista rather than in Kingdom Plantae?
Cell Structure
The cells of protists are among the most elaborate of all cells. Most protists are microscopic and unicellular, but some multicellular forms exist. A few protists live as colonies that behave in some ways as a group of independent free-living cells and in other ways as a multicellular organism. Not all protists are microscopic. Some have huge, macroscopic cells, such as the plasmodia (giant amoebae) of myxomycete slime molds or the marine green alga Caulerpa. Some protists are multicellular, such as the red, green, and brown seaweeds. It is among the protists that one finds the wealth of ways that organisms can grow. In fact, many protist cells are multinucleated; in some species, the nuclei are different sizes and have distinct roles in protist cell function.
Single protist cells range in size from less than a micrometer to three meters in length, to hectares in area. Protist cells may be enveloped by animal-like cell membranes or plant-like cell walls. Others are encased in glassy silica-based shells or wound with pellicles of interlocking protein strips (Figure \(\PageIndex{4}\)). The pellicle functions like a flexible coat of armor, preventing the protist from being torn or pierced without compromising its range of motion.

Classifying Protists
In the span of several decades, the Kingdom Protista has been disassembled because sequence analyses have revealed new genetic (and therefore evolutionary) relationships among these eukaryotes. Moreover, protists that exhibit similar morphological features may have evolved analogous structures because of similar selective pressures—rather than because of recent common ancestry. This convergent evolution is one reason why protist classification is so challenging.
The emerging classification scheme groups the entire domain Eukaryota into six “supergroups” that contain all the protists as well as animals, plants ("land plants" in the figure), and fungi that evolved from a common ancestor (Figure \(\PageIndex{2}\)). The supergroups are believed to be monophyletic, meaning that all organisms within each supergroup are believed to have evolved from a single common ancestor, and thus all members are most closely related to each other than to organisms outside that group. There is still evidence lacking for the monophyly of some groups.
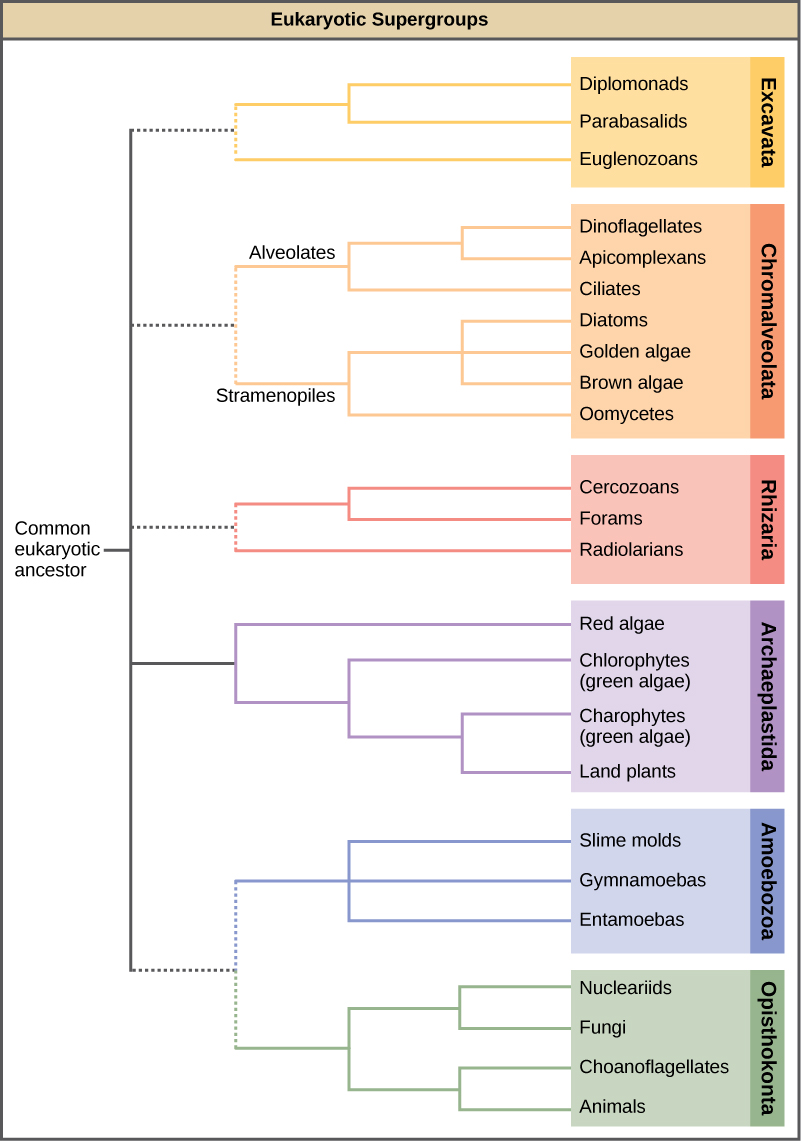
The classification of eukaryotes is still in flux, and the six supergroups may be modified or replaced by a more appropriate hierarchy as genetic, morphological, and ecological data accumulate. Keep in mind that the classification scheme presented here is just one of several hypotheses, and the true evolutionary relationships are still to be determined. Above all, eukaryotes are organisms whose cells have membrane-bound organelles, including nuclei.
Excavata
Many of the protist species classified into the supergroup Excavata are asymmetrical, single-celled organisms with a feeding groove “excavated” from one side. This supergroup includes heterotrophic predators, photosynthetic species, and parasites. Its subgroups are the diplomonads, parabasalids, and euglenozoans.
Diplomonads
Diplomonads exist in anaerobic environments and use alternative pathways, such as glycolysis, to generate energy. Each diplomonad cell has two identical nuclei and uses several flagella for locomotion.
Parabasalids
Parabasalids move with flagella and membrane rippling. Trichomonas vaginalis, a parabasalid that causes a sexually transmitted disease in humans, employs these mechanisms to transit through the male and female urogenital tracts. T. vaginalis causes trichamoniasis, which appears in an estimated 180 million cases worldwide each year.
Euglenozoans
Euglenozoans includes parasites, heterotrophs, autotrophs, and mixotrophs, ranging in size from 10 to 500 µm. Euglenoids move through their aquatic habitats using two long flagella that guide them toward light sources sensed by a primitive ocular organ called an eyespot. The familiar genus, Euglena, encompasses some mixotrophic species that display a photosynthetic capability only when light is present. In the dark, the chloroplasts of Euglena shrink up and temporarily cease functioning, and the cells instead take up organic nutrients from their environment.
Euglenoids have had a very, very long time to evolve, and that has led to the things they have evolved into being extremely diverse—so diverse that, combined with the varied shape-shifting abilities of its member species, euglenoids have proven challenging to both identify and classify.
Chromalveolata
Current evidence suggests that species classified as chromalveolates are derived from a common ancestor that engulfed a photosynthetic red algal cell, which itself had already evolved chloroplasts from an endosymbiotic relationship with a photosynthetic prokaryote. Therefore, the ancestor of chromalveolates is believed to have resulted from a secondary endosymbiotic event. However, some chromalveolates appear to have lost red alga-derived plastid organelles or lack plastid genes altogether. Therefore, this supergroup should be considered a hypothesis-based working group that is subject to change. Chromalveolates include very important photosynthetic organisms, such as diatoms, brown algae, and significant disease agents in animals and plants. The chromalveolates can be subdivided into alveolates and stramenopiles.
Alveolates: Dinoflagellates, Apicomplexians, and Ciliates
A large body of data supports that the alveolates are derived from a shared common ancestor. The alveolates are named for the presence of an alveolus, or membrane-enclosed sac, beneath the cell membrane. The exact function of the alveolus is unknown, but it may be involved in osmoregulation. The alveolates are further categorized into some of the better-known protists: the dinoflagellates, the apicomplexans, and the ciliates.
Dinoflagellates exhibit extensive morphological diversity and can be photosynthetic, heterotrophic, or mixotrophic. Many dinoflagellates are encased in interlocking plates of cellulose. Two perpendicular flagella fit into the grooves between the cellulose plates, with one flagellum extending longitudinally and a second encircling the dinoflagellate. Together, the flagella contribute to the characteristic spinning motion of dinoflagellates. These protists exist in freshwater and marine habitats, and are a component of plankton, the typically microscopic organisms that drift through the water and serve as a crucial food source for larger aquatic organisms.
The apicomplexan protists are so named because their microtubules, fibrin, and vacuoles are asymmetrically distributed at one end of the cell in a structure called an apical complex (Figure \(\PageIndex{3a}\)). The apical complex is specialized for entry and infection of host cells. Indeed, all apicomplexans are parasitic. This group includes the genus Plasmodium, which causes malaria in humans (Figure \(\PageIndex{3b}\)). Apicomplexan life cycles are complex, involving multiple hosts and stages of sexual and asexual reproduction.
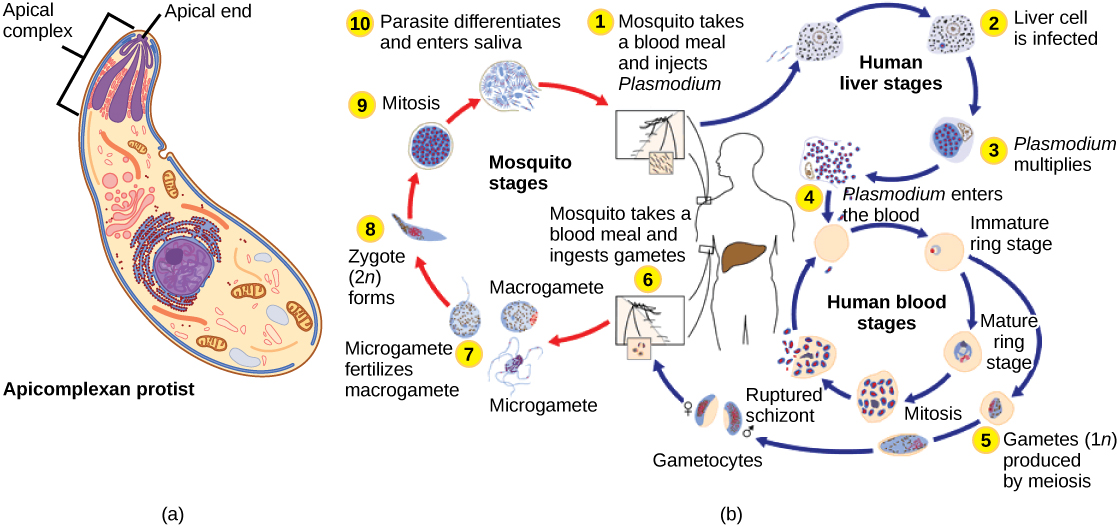
The ciliates, which include Paramecium and Tetrahymena, are a group of protists 10 to 3,000 micrometers in length that are covered in rows, tufts, or spirals of tiny cilia. By beating their cilia synchronously or in waves, ciliates can coordinate directed movements and ingest food particles. Certain ciliates have fused cilia-based structures that function like paddles, funnels, or fins. Ciliates also are surrounded by a pellicle, providing protection without compromising agility. The genus Paramecium includes protists that have organized their cilia into a plate-like primitive mouth, called an oral groove, which is used to capture and digest bacteria.
Stramenopiles: Diatoms, Brown Algae, Golden Algae, and Oomycetes
The other subgroup of chromalveolates, the stramenopiles, includes photosynthetic marine algae and heterotrophic protists. The unifying feature of this group is the presence of a textured, or “hairy,” flagellum. Many stramenopiles also have an additional flagellum that lacks hair-like projections. Members of this subgroup range in size from single-celled diatoms to the massive and multicellular kelp.
The diatoms are unicellular photosynthetic protists that encase themselves in intricately patterned, glassy cell walls composed of silicon dioxide in a matrix of organic particles. These protists are a component of freshwater and marine plankton. Most species of diatoms reproduce asexually, although some instances of sexual reproduction and sporulation also exist.
Like diatoms, golden algae are largely unicellular, although some species can form large colonies. Their characteristic gold color results from their extensive use of carotenoids, a group of photosynthetic pigments that are generally yellow or orange in color. Golden algae are found in both freshwater and marine environments, where they form a major part of the plankton community.
The brown algae are primarily marine, multicellular organisms that are known colloquially as seaweeds. Giant kelps are a type of brown algae. Some brown algae have evolved specialized tissues that resemble terrestrial plants, with root-like holdfasts, stem-like stipes, and leaf-like blades that are capable of photosynthesis. The stipes of giant kelps are enormous, extending in some cases for 60 meters. A variety of algal life cycles exists, but the most complex is alternation of generations, in which both haploid and diploid stages involve multicellularity.
The water molds, oomycetes (“egg fungus”), were so-named based on their fungus-like morphology, but molecular data have shown that the water molds are not closely related to fungi. The oomycetes are characterized by a cellulose-based cell wall and an extensive network of filaments that allow for nutrient uptake. As diploid spores, many oomycetes have two oppositely directed flagella (one hairy and one smooth) for locomotion.
Rhizaria
The Rhizaria supergroup includes many of the amoebas, most of which have threadlike or needle-like pseudopodia (Figure 5.4.3.135.4.3.13). Pseudopodia function to trap and engulf food particles and to direct movement in rhizarian protists. These pseudopods project outward from anywhere on the cell surface and can anchor to a substrate. The protist then transports its cytoplasm into the pseudopod, thereby moving the entire cell. This type of motion, called cytoplasmic streaming, is used by several diverse groups of protists as a means of locomotion or as a method to distribute nutrients and oxygen.
Foraminifera
Foraminiferans, or forams, are unicellular heterotrophic protists, ranging from approximately 20 micrometers to several centimeters in length, and occasionally resembling tiny snails. As a group, the forams exhibit porous shells, called tests that are built from various organic materials and typically hardened with calcium carbonate. The tests may house photosynthetic algae, which the forams can harvest for nutrition. Foram pseudopodia extend through the pores and allow the forams to move, feed, and gather additional building materials. Typically, forams are associated with sand or other particles in marine or freshwater habitats. Foraminiferans are also useful as indicators of pollution and changes in global weather patterns.
Radiolarians
A second subtype of Rhizaria, the radiolarians, exhibit intricate exteriors of glassy silica with radial or bilateral symmetry. Needle-like pseudopods supported by microtubules radiate outward from the cell bodies of these protists and function to catch food particles. The shells of dead radiolarians sink to the ocean floor, where they may accumulate in 100 meter-thick depths. Preserved, sedimented radiolarians are very common in the fossil record.
Archaeplastida
Red algae and green algae are included in the supergroup Archaeplastida. It was from a common ancestor of these protists that the land plants evolved, since their closest relatives are found in this group. Molecular evidence supports that all Archaeplastida are descendents of an endosymbiotic relationship between a heterotrophic protist and a cyanobacterium. The red and green algae include unicellular, multicellular, and colonial forms.
Red Algae
Red algae, or rhodophytes, are primarily multicellular, lack flagella, and range in size from microscopic, unicellular protists to large, multicellular forms grouped into the informal seaweed category. The red algae life cycle is an alternation of generations. Some species of red algae contain phycoerythrins, photosynthetic accessory pigments that are red in color and outcompete the green tint of chlorophyll, making these species appear as varying shades of red. Other protists classified as red algae lack phycoerythrins and are parasites. Red algae are common in tropical waters where they have been detected at depths of 260 meters. Other red algae exist in terrestrial or freshwater environments.
Green Algae: Chlorophytes and Charophytes
The most abundant group of algae is the green algae. The green algae exhibit similar features to the land plants, particularly in terms of chloroplast structure. That this group of protists shared a relatively recent common ancestor with land plants is well supported. The green algae are subdivided into the chlorophytes and the charophytes. The charophytes are the closest living relatives to land plants and resemble them in morphology and reproductive strategies. Charophytes are common in wet habitats, and their presence often signals a healthy ecosystem.
The chlorophytes exhibit great diversity of form and function. Chlorophytes primarily inhabit freshwater and damp soil, and are a common component of plankton. Chlamydomonas is a simple, unicellular chlorophyte with a pear-shaped morphology and two opposing, anterior flagella that guide this protist toward light sensed by its eyespot. More complex chlorophyte species exhibit haploid gametes and spores that resemble Chlamydomonas.
True multicellular organisms, such as the sea lettuce, Ulva, are represented among the chlorophytes. In addition, some chlorophytes exist as large, multinucleate, single cells. Species in the genus Caulerpa exhibit flattened fern-like foliage and can reach lengths of 3 meters. Caulerpa species undergo nuclear division, but their cells do not complete cytokinesis, remaining instead as massive and elaborate single cells (Figure \(\PageIndex{5}\)).
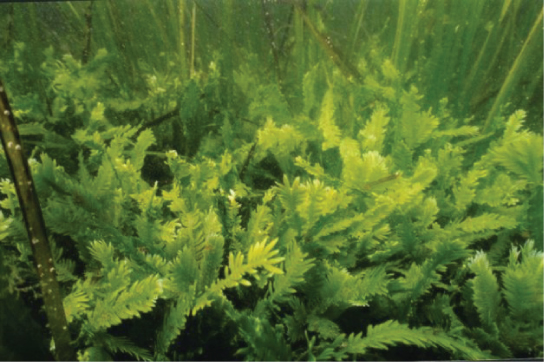
Amoebozoa
The amoebozoans characteristically exhibit pseudopodia that extend like tubes or flat lobes, rather than the hair-like pseudopodia of rhizarian amoeba. The Amoebozoa include several groups of unicellular amoeba-like organisms that are free-living or parasites.
Slime Molds
A subset of the amoebozoans, the slime molds, has several morphological similarities to fungi that are thought to be the result of convergent evolution. For instance, during times of stress, some slime molds develop into spore-generating fruiting bodies, much like fungi.
The slime molds are categorized on the basis of their life cycles into plasmodial or cellular types. Plasmodial slime molds are composed of large, multinucleate cells and move along surfaces like an amorphous blob of slime during their feeding stage (Figure 5.4.3.195.4.3.19). Food particles are lifted and engulfed into the slime mold as it glides along. Upon maturation, the plasmodium takes on a net-like appearance with the ability to form fruiting bodies, or sporangia, during times of stress. Haploid spores are produced by meiosis within the sporangia, and spores can be disseminated through the air or water to potentially land in more favorable environments. If this occurs, the spores germinate to form ameboid or flagellate haploid cells that can combine with each other and produce a diploid zygotic slime mold to complete the life cycle.
Unlike the cells of other living beings, which have only one nucleus that carries their genetic information, slime molds can organize into something like a cell with thousands of nuclei.
Question after watching:
Opisthokonta
The opisthokonts include the animal-like choanoflagellates, which are believed to resemble the common ancestor of sponges and, in fact, all animals. Choanoflagellates include unicellular and colonial forms, and number about 244 described species. These organisms exhibit a single, apical flagellum that is surrounded by a contractile collar composed of microvilli. The collar uses a similar mechanism to sponges to filter out bacteria for ingestion by the protist. The morphology of choanoflagellates was recognized early on as resembling the collar cells of sponges, and suggesting a possible relationship to animals.
Metabolism
Protists exhibit many forms of nutrition and may be aerobic or anaerobic. Protists that store energy by photosynthesis belong to a group of photoautotrophs and are characterized by the presence of chloroplasts. Other protists are heterotrophic and consume organic materials (such as other organisms) to obtain nutrition. Amoebas and some other heterotrophic protist species ingest particles by a process called phagocytosis, in which the cell membrane engulfs a food particle and brings it inward, pinching off an intracellular membranous sac, or vesicle, called a food vacuole (Figure \(\PageIndex{6}\)). The food particle is broken down into small molecules that can diffuse into the cytoplasm and be used in cellular metabolism. Undigested materials are expelled from the cell via exocytosis.
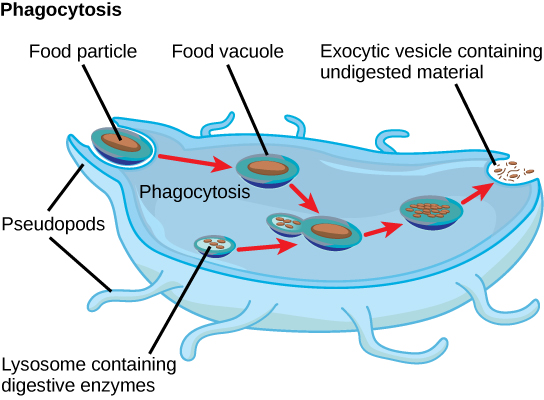
Subtypes of heterotrophs, called saprobes, absorb nutrients from dead organisms or their organic wastes. Some protists can function as mixotrophs, obtaining nutrition by photoautotrophic or heterotrophic routes, depending on whether sunlight or organic nutrients are available.
In this 11-minute video, the diverse ways that protist extract energy from their environment are explored.
Question after watching: What is the advantage of being a mixotroph in aquatic environments?
Yes, they might eat your brain, but there's a lot more to amoebas than that!
Question after watching:
Motility
Most protists are motile, but different types of protists have evolved different modes of movement (Figure \(\PageIndex{6}\)). Some protists have one or more flagella, which they rotate or whip. Others are covered in rows or tufts of tiny cilia that they coordinately beat to swim. Still others form cytoplasmic extensions called pseudopodia anywhere on the cell, anchor the pseudopodia to a substrate, and pull themselves forward. Some protists can move toward or away from a stimulus, a movement referred to as taxis. Movement toward light, termed phototaxis, is accomplished by coupling their locomotion strategy with a light-sensing organ.
In this 11-minute video, find out how these single-celled eukaryotes move through their viscous environment.
Question after watching: After making a table with the three main types of possible movements, which representative organisms would you include to help you remember each one?
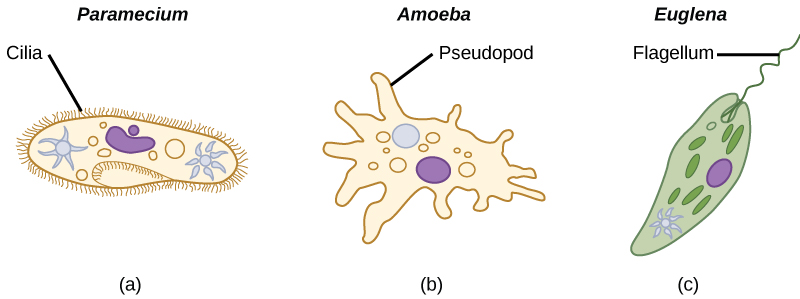
Life Cycles
Protists reproduce by many mechanisms. Most undergo some form of asexual reproduction, such as binary fission, to produce two daughter cells. In protists, binary fission can occur along the transverse or longitudinal axis of the cell, depending on the axis of orientation. Sometimes Paramecium uses binary fission to reproduce. Some protists such as the true slime molds exhibit multiple fission and simultaneously divide into many daughter cells. Others produce tiny buds that go on to divide and grow to the size of the parental protist.
Sexual reproduction, involving meiosis and fertilization, is also found among protists, and many protist species can switch from asexual to sexual reproduction when necessary. Sexual reproduction is often associated with periods of stress such as when nutrients are rare or environmental changes occur. Sexual reproduction may allow the protist to recombine genes and produce new variations in its progeny that may be better suited to surviving in the new environment.
In addition, sexual reproduction is often associated with the creation of resistant cysts that are a protective, resting stage of the life cycle of the protist. Depending on their habitat, the cysts may be particularly resistant to temperature extremes, desiccation, or low pH. This strategy also allows certain protists to “wait out” stressors until their environment becomes more favorable for survival or until they are carried (such as by wind, water, or transport on a larger organism) to a different environment because cysts exhibit little cellular metabolism and leave the organism in a sort of suspended animation.
Protist life cycles range from simple to complex. Certain parasitic protists have complicated life cycles and must infect different host species at different developmental stages to complete their life cycle. Some protists are unicellular in the haploid form and multicellular in the diploid form, a strategy employed by animals (gametes are unicellular, but fertilized eggs rapidly develop and divide to form multicellular animals). Other protists have multicellular stages in both haploid and diploid forms, a strategy called alternation of generations that is also used by plants.
In this 8.5-minute video, explore the ways that protists reproduce.
Question after watching: Explain in your own words why sexual reproduction can be useful if a protist’s environment changes.
Ecology
Nearly all protists exist in aquatic environments, including freshwater and marine environments, damp soil, and even snow. They are also common in the digestive tracts of animals and in the vascular tissues of plants. Others invade the cells of other protists, animals, and plants. Protists function in diverse ecological niches. Whereas some protist species are essential components of the food chain and generators of biomass, others function in the decomposition of organic materials. Still other protists are dangerous human pathogens or causative agents of devastating plant diseases. Several protist species are parasites that infect animals or plants. A few protist species live on dead organisms or their wastes and contribute to their decay.
Primary Producers
Protists are essential sources of nutrition for many other organisms. In some cases, as in plankton, protists are consumed directly. Alternatively, photosynthetic protists serve as producers of nutrition for other organisms. The protists themselves and their products of photosynthesis are essential—directly or indirectly—to the survival of organisms ranging from bacteria to mammals (Figure \(\PageIndex{7}\)). As primary producers, protists feed a large proportion of the world’s aquatic species. (On land, terrestrial plants serve as primary producers.) In fact, approximately one-quarter of the world’s photosynthesis is conducted by protists, particularly dinoflagellates, diatoms, and multicellular algae.
Protists do not create food sources only for sea-dwelling organisms. For instance, certain anaerobic parabasalid species exist in the digestive tracts of termites and wood-eating cockroaches, where they contribute an essential step in the digestion of cellulose ingested by these insects as they bore through wood.
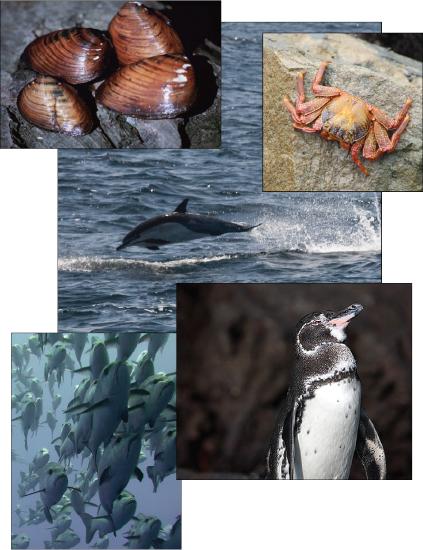
Protists as Bioremediators
Microbes are used for everything from baking to brewing, but wastewater treatment is where they do some of their most important work.
Question after watching:
Human Parasites
The human parasite, Trypanosoma brucei, belongs to a subgroup of Euglenozoa, the kinetoplastids. The kinetoplastid subgroup is named after the kinetoplast, a DNA mass carried within the single, oversized mitochondrion possessed by each of these cells. This subgroup includes several parasites, collectively called trypanosomes, which cause devastating human diseases and infect an insect species during a portion of their life cycle. T. brucei develops in the gut of the tsetse fly after the fly bites an infected human or other mammalian host. T. brucei is responsible for African sleeping sickness, common in central Africa, and confounds the human immune system by changing its thick layer of surface glycoproteins with each infectious cycle.
The glycoproteins are identified by the immune system as foreign antigens, and a specific antibody defense is mounted against the parasite. However, T. brucei has thousands of possible antigens, and with each subsequent generation, the protist switches to a glycoprotein coating with a different molecular structure. In this way, T. brucei is capable of replicating continuously without the immune system ever succeeding in clearing the parasite.
T. brucei attacks red blood cells. The parasite then travels to the insect salivary glands to be transmitted to another human or other mammal when the infected tsetse fly consumes another blood meal. The disease is associated with severe chronic fatigue, coma, and without treatment, eventual death. During epidemic periods, mortality from the disease can be high. Greater surveillance and control measures lead to a reduction in reported cases; some of the lowest numbers reported in 50 years (fewer than 10,000 cases in all of sub-Saharan Africa) have happened since 2009.
Plant Parasites
Protist parasites of terrestrial plants include agents that destroy food crops. The oomycete Plasmopara viticola parasitizes grape plants, causing a disease called downy mildew (Figure \(\PageIndex{8}\)). Grape plants infected with P. viticola appear stunted and have discolored, withered leaves. The spread of downy mildew nearly collapsed the French wine industry in the nineteenth century.
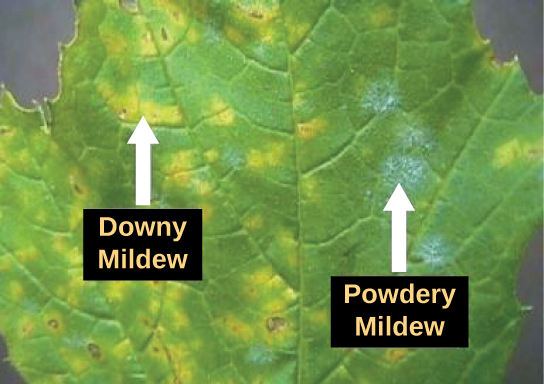
Phytophthora infestans is an oomycete responsible for potato late blight, which causes potato stalks and stems to decay into black slime (Figure \(\PageIndex{9}\)). Widespread potato blight caused by P. infestans precipitated the well-known Irish potato famine in the nineteenth century that claimed the lives of approximately 1 million people and led to the emigration of at least 1 million more from Ireland. Late blight continues to plague potato crops in certain parts of the United States and Russia, wiping out as much as 70 percent of crops when no pesticides are applied.
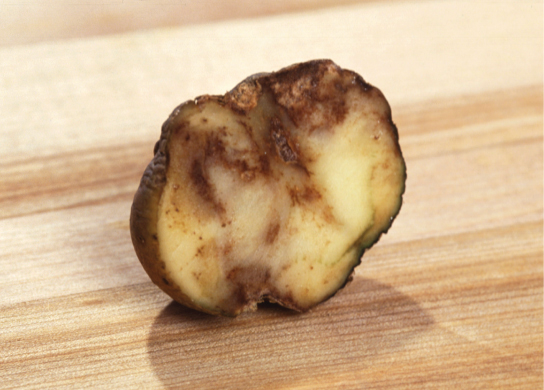
Protists with the capabilities to perform photosynthesis and to absorb nutrients from dead organisms are called ______________.
- photoautotrophs
- mixotrophs
- saprobes
- heterotrophs
- Answer
-
B. mixotrophs
Which of these locomotor organs would likely be the shortest?
- a flagellum
- a cilium
- an extended pseudopod
- a pellicle
- Answer
-
B. a cilium
Thumbnail: This scanning electron micrograph (SEM) revealed some of the external ultrastructural details displayed by a flagellated Giardia lamblia protozoan parasite. G. lamblia is the organism responsible for causing the diarrheal disease "giardiasis". (Public Domain; CDC / Janice Haney Carr).