4.2.3: Life Histories and Natural Selection
- Page ID
- 79386
Unit 4.2.3 - Life Histories and Natural Selection
- Please read and watch the following Learning Resources
- Reading the material for understanding, and taking notes during videos, will take approximately 1.5 hours.
- Optional Activities are embedded.
- Bolded terms are located at the end of the unit in the Glossary. There is also a Unit Summary at the end of the Unit.
- To navigate to Unit 4.2.4, use the Contents menu at the top of the page OR the right arrow on the side of the page.
- If on a mobile device, use the Contents menu at the top of the page OR the links at the bottom of the page.
- Describe how life history patterns are influenced by natural selection
- Explain different life history patterns and how different reproductive strategies affect species’ survival
- Describe how natural selection and environmental adaptation lead to the evolution of particular life-history patterns
Introduction
Life history theory is an analytical framework designed to study the diversity of life history strategies used by different organisms throughout the world, as well as the causes and results of the variation in their life cycles. It is a theory of biological evolution that seeks to explain aspects of organisms' anatomy and behavior by reference to the way that their life histories—including their reproductive development and behaviors, post-reproductive behaviors, and lifespan (length of time alive)—have been shaped by natural selection. A life history strategy is the "age- and stage-specific patterns" and timing of events that make up an organism's life, such as birth, weaning, maturation, death, etc. These events, notably juvenile development, age of sexual maturity, first reproduction, number of offspring and level of parental investment, senescence and death, depend on the physical and ecological environment of the organism.
In this 4-minute video, learn how life history traits include significant life events of a species, such as reproductive rate and reproductive lifespan.
Question after watching: How do these life history traits relate to natural selection?
The theory was developed in the 1950s and is used to answer questions about organism size, age of maturation, number of offspring, lifespan, and many others. In order to study these topics, life history strategies must be identified, and then models are constructed to study their effects. Finally, predictions about the importance and role of the strategies are made, and these predictions are used to understand how evolution affects the ordering and length of life history events in an organism's life, particularly the lifespan and period of reproduction. Life history theory draws on an evolutionary foundation, and studies the effects of natural selection on organisms, both throughout their lifetime and across generations. It also uses measures of evolutionary fitness to determine if organisms are able to maximize or optimize this fitness, by allocating resources to a range of different demands throughout the organism's life.
Organisms have evolved a great variety of life histories, from Pacific salmon, which produce thousands of eggs at one time and then die, to human beings, who produce a few offspring over the course of decades. The theory depends on principles of evolutionary biology and ecology and is widely used in other areas of science.
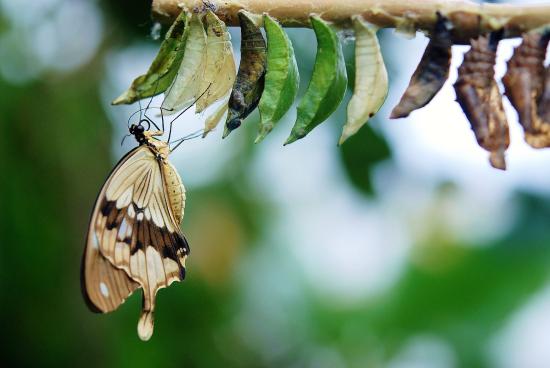
This 15-minute video introduces Pacific salmon and their life cycle. Keep these fish in mind as you read through the sections below.
Question after watching: What adaptations are noted in the video? Which do you notice that are not explicitly stated?
Life cycle
All organisms follow a specific sequence in their development, known as the life cycle. All events and adaptations of life cycle comprise the life history strategy of that species. The major events in this life cycle are often shaped by the demographic qualities of the organism. Some are more obvious than others, like teeth erupting in young children, while some events may have little variation between individuals, such as the length of gestation. Other events may show a lot of variation between individuals, such as age at first reproduction. Life cycles across all life forms can be divided into two major stages: growth and reproduction. These two cannot take place at the same time, so once reproduction has begun, growth usually ends. This shift is important because it can also affect other aspects of an organism's life, such as the organization of its group or its social interactions.
Each species has its own pattern and timing for these events, often known as its ontogeny, and the variety produced by this is what life history theory addresses. Evolution then works upon these stages to ensure that an organism adapts to its environment. For example, a human, between being born and reaching adulthood, will pass through an assortment of life stages, which include: birth, infancy, weaning, childhood and growth, adolescence, sexual maturation, reproduction, and senescence. All of these are defined in a specific biological way. In life history theory, evolution works on the life stages of particular species (e.g., length of juvenile period) but is also discussed for a single organism's functional, lifetime adaptation. In both cases, researchers assume adaptation—processes that establish fitness.
Traits
There are at least seven traits that are traditionally recognized as important in life history theory. The trait that is seen as the most important for any given organism is the one where a change in that trait creates the most significant difference in that organism's level of fitness. In this sense, an organism's fitness is determined by its changing life history traits. The way in which evolutionary forces act on these life history traits serves to limit the genetic variability and heritability of the life history strategies, although there are still large varieties that exist in the world.
Commonly studied life history traits:
- size at birth (animals)
- size of seeds/spores (plants and animals)
- growth pattern
- age and size at maturity
- number, size, and sex ratio of offspring
- age- and size-specific reproductive investments
- age- and size-specific mortality schedules
- length of life.
Life History Patterns and Energy Budgets
Energy is required by all living organisms for their growth, maintenance, and reproduction; at the same time, energy is often a major limiting factor in determining an organism’s survival. Plants, for example, acquire energy from the sun via photosynthesis, but must expend this energy to grow, maintain health, and produce energy-rich seeds to produce the next generation. Animals have the additional burden of using some of their energy reserves to acquire food. Furthermore, some animals must expend energy caring for their offspring. Thus, all species have an energy budget: they must balance energy intake with their use of energy for metabolism, reproduction and parental care, and energy storage (such as bears building up body fat for winter hibernation).
Parental Care and Fecundity
Fecundity is the potential reproductive capacity of an individual within a population. In other words, fecundity describes how many offspring could ideally be produced if an individual has as many offspring as possible, repeating the reproductive cycle as soon as possible after the birth of the offspring. In animals, fecundity is inversely related to the amount of parental care given to an individual offspring. Species, such as many marine invertebrates, that produce many offspring may provide little if any care for the offspring. Most of their reproductive energy budget is used to produce many tiny offspring. Animals with this strategy are often self-sufficient at a very early age. This is because of the energy tradeoff these organisms have made to maximize their evolutionary fitness. Because their energy is used for producing offspring instead of parental care, it makes sense that these offspring have some ability to be able to move within their environment and find food and perhaps shelter. Even with these abilities, their small size makes them extremely vulnerable to predation, so the production of many offspring allows enough of them to survive to maintain the species.
Animal species that have few offspring during a reproductive event usually give extensive parental care, devoting much of their energy budget to these activities, sometimes at the expense of their own health. This is the case with many mammals, such as humans, kangaroos, and pandas. The offspring of these species are relatively helpless at birth and need to develop before they achieve self-sufficiency.
Plants with low fecundity produce few energy-rich seeds (such as coconuts and chestnuts) with each having a good chance to germinate into a new organism; plants with high fecundity usually have many small, energy-poor seeds (like orchids) that have a relatively poor chance of surviving. Although it may seem that coconuts and chestnuts have a better chance of surviving, the energy tradeoff of the orchid is also very effective. It is a matter of where the energy is used, for large numbers of seeds or for fewer seeds with more energy.
Early versus Late Reproduction
The timing of reproduction in a life history also affects species survival. Organisms that reproduce at an early age have a greater chance of producing offspring, but this is usually at the expense of their growth and the maintenance of their health. Conversely, organisms that start reproducing later in life often have greater fecundity or are better able to provide parental care, but they risk that they will not survive to reproductive age. Examples of this can be seen in fishes. Small fish like guppies use their energy to reproduce rapidly, but never attain the size that would give them defense against some predators. Larger fish, like the bluegill or shark, use their energy to attain a large size, but do so with the risk that they will die before they can reproduce or at least reproduce to their maximum. These different energy strategies and trade-offs are key to understanding the evolution of each species as it maximizes its fitness and fills its niche. In terms of energy budgeting, some species “blow it all” and use up most of their energy reserves to reproduce early before they die. Other species delay having reproduction to become stronger, more experienced individuals and to make sure that they are strong enough to provide parental care if necessary.
Single versus Multiple Reproductive Events
Some life history traits, such as fecundity, timing of reproduction, and parental care, can be grouped together into general strategies that are used by multiple species. Semelparity occurs when a species reproduces only once during its lifetime and then dies. Such species use most of their resource budget during a single reproductive event, sacrificing their health to the point that they do not survive. Examples of semelparity are bamboo, which flowers once and then dies, and the Chinook salmon (Figure \(\PageIndex{3}\)a), which uses most of its energy reserves to migrate from the ocean to its freshwater nesting area, where it reproduces and then dies. Scientists have posited alternate explanations for the evolutionary advantage of the Chinook’s post-reproduction death: a programmed suicide caused by a massive release of corticosteroid hormones, presumably so the parents can become food for the offspring, or simple exhaustion caused by the energy demands of reproduction; these are still being debated.
Iteroparity describes species that reproduce repeatedly during their lives. Some animals are able to mate only once per year, but survive multiple mating seasons. The pronghorn antelope is an example of an animal that goes into a seasonal estrus cycle (“heat”): a hormonally induced physiological condition preparing the body for successful mating (Figure \(\PageIndex{3}\)b). Females of these species mate only during the estrus phase of the cycle. A different pattern is observed in primates, including humans and chimpanzees, which may attempt reproduction at any time during their reproductive years, even though their menstrual cycles make pregnancy likely only a few days per month during ovulation (Figure \(\PageIndex{3}\)c).
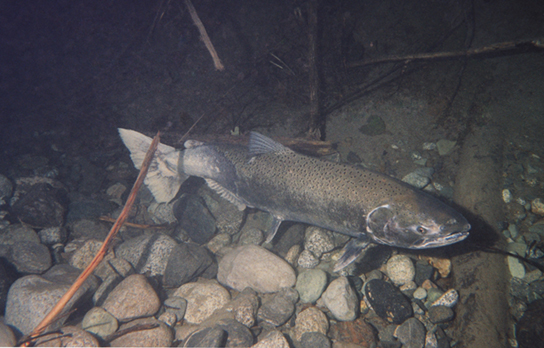
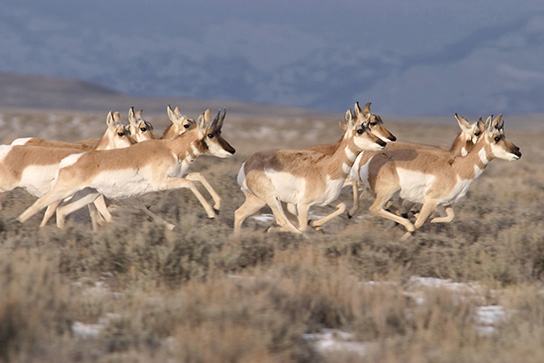
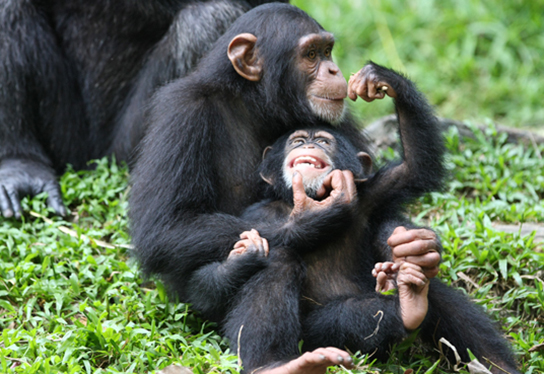
Which of the following is associated with long-term parental care?
- few offspring
- many offspring
- semelparity
- fecundity
- Answer
-
a. few offspring
Trade-offs
>An essential component of studying life history strategies is identifying the trade-offs[ that take place for any given organism. Energy use in life history strategies is regulated by thermodynamics and the conservation of energy, and the "inherent scarcity of resources", so not all traits or tasks can be invested in at the same time. Thus, organisms must choose between tasks, such as growth, reproduction, and survival, prioritizing some and not others. For example, there is a trade-off between maximizing body size and maximizing lifespan, and between maximizing offspring size and maximizing offspring number. This is also sometimes seen as a choice between quantity and quality of offspring. These choices are the trade-offs that life history theory studies.
One significant trade-off is between somatic effort (towards growth and maintenance of the body) and reproductive effort (towards producing offspring). Since an organism cannot put energy towards doing these simultaneously, many organisms have a period where energy is put just toward growth, followed by a period where energy is focused on reproduction, creating a separation of the two in the life cycle. Thus, the end of the period of growth marks the beginning of the period of reproduction. Another fundamental trade-off associated with reproduction is between mating effort and parenting effort. If an organism is focused on raising its offspring, it cannot devote that energy to pursuing a mate.
An important trade-off in the dedication of resources to breeding has to do with predation risk: organisms that have to deal with an increased risk of predation often invest less in breeding. This is because it is not worth as much to invest much in breeding when the benefit of such investment is uncertain.
These trade-offs, once identified, can then be put into models that estimate their effects on different life history strategies and answer questions about the selection pressures that exist on different life events. Over time, there has been a shift in how these models are constructed. Instead of focusing on one trait and looking at how it changed, scientists are looking at these trade-offs as part of a larger system, with complex inputs and outcomes.
The idea of constraints is closely linked to the idea of trade-offs discussed above. Because organisms have a finite amount of energy, the process of trade-offs acts as a natural limit on the organism's adaptations and potential for fitness. These limits can be physical, developmental, or historical, and they are imposed by the existing traits of the organism.
Populations can adapt and thereby achieve an "optimal" life history strategy that allows the highest level of fitness possible (fitness maximization). There are several methods from which to approach the study of optimality, including energetic and demographic. Achieving optimal fitness also encompasses multiple generations, because the optimal use of energy includes both the parents and the offspring. For example, "optimal investment in offspring is where the decrease in total number of offspring is equaled by the increase of the number who survive". Optimality is important for the study of life history theory because it serves as the basis for many of the models used, which work from the assumption that natural selection, as it works on a life history traits, is moving towards the most optimal group of traits and use of energy. This base assumption, that over the course of its lifespan an organism is aiming for optimal energy use, then allows scientists to test other predictions. However, actually gaining this optimal life history strategy cannot be guaranteed for any organism.
An organism's allocation of resources ties into several other important concepts, such as trade-offs and optimal strategies. The best possible allocation of resources is what allows an organism to achieve an optimal life history strategy and obtain the maximum level of fitness, and making the best possible choices about how to allocate energy to various trade-offs contributes to this. The allocation of resources also plays a role in variation, because the different resource allocations by different species create the variety of life history strategies.
Unpredictable environments
Many factors can determine the evolution of an organism's life history, especially the unpredictability of the environment. A very unpredictable environment—one in which resources, hazards, and competitors may fluctuate rapidly—selects for organisms that produce more offspring earlier in their lives, because it is never certain whether they will survive to reproduce again. Mortality rate, the number of individuals who die per 1,000 over a given time, might be the best indicator of a species' life history: organisms with high mortality rates—the usual result of an unpredictable environment—typically mature earlier than those species with low mortality rates, and give birth to more offspring at a time.
Early Theories about Life History: K-selected and r-selected Species
While reproductive strategies play a key role in life histories, they do not account for important factors like limited resources and competition. The regulation of population growth by these factors can be used to introduce a classical concept in population biology, that of K-selected versus r-selected species. "K" is the biological term for carrying capacity and "r" indicates a focus on reproductive effort (see Unit 4.2.5)
By the second half of the twentieth century, the concept of K- and r-selected species was used extensively and successfully to study populations. The concept relates not only reproductive strategies, but also to a species’ habitat and behavior, especially in the way that they obtain resources and care for their young. It includes the length of life and survivorship factors as well. For this analysis, population biologists have grouped species into two large categories—K-selected and r-selected—although they are really two ends of a continuum (Table \(\PageIndex{1}\)).
Characteristics of K-selected species | Characteristics of r-selected species |
---|---|
Mature late | Mature early |
Greater longevity | Lower longevity |
Increased parental care | Decreased parental care |
Increased competition | Decreased competition |
Fewer offspring | More offspring |
Larger offspring | Smaller offspring |
K-selected species are species selected by stable, predictable environments. Populations of K-selected species tend to exist close to their carrying capacity (hence the term K-selected) where intraspecific competition is high. These species have few, large offspring, a long gestation period, and often give long-term care to their offspring. While larger in size when born, the offspring are relatively helpless and immature at birth. By the time they reach adulthood, they must develop skills to compete for natural resources. In plants, scientists think of parental care more broadly: how long fruit takes to develop or how long it remains on the plant are determining factors in the time to the next reproductive event. Examples of K-selected species are primates including humans, elephants, and plants such as oak trees (Figure \(\PageIndex{3}\)a).
Oak trees grow very slowly and take, on average, 20 years to produce their first seeds, known as acorns. As many as 50,000 acorns can be produced by an individual tree, but the germination rate is low as many of these rot or are eaten by animals such as squirrels. In some years, oaks may produce an exceptionally large number of acorns, and these years may be on a two- or three-year cycle depending on the species of oak (K-selection).
As oak trees grow to a large size and for many years before they begin to produce acorns, they devote a large percentage of their energy budget to growth and maintenance. The tree’s height and size allow it to dominate other plants in the competition for sunlight, the oak’s primary energy resource. Furthermore, when it does reproduce, the oak produces large, energy-rich seeds that use their energy reserve to become quickly established (K-selection).
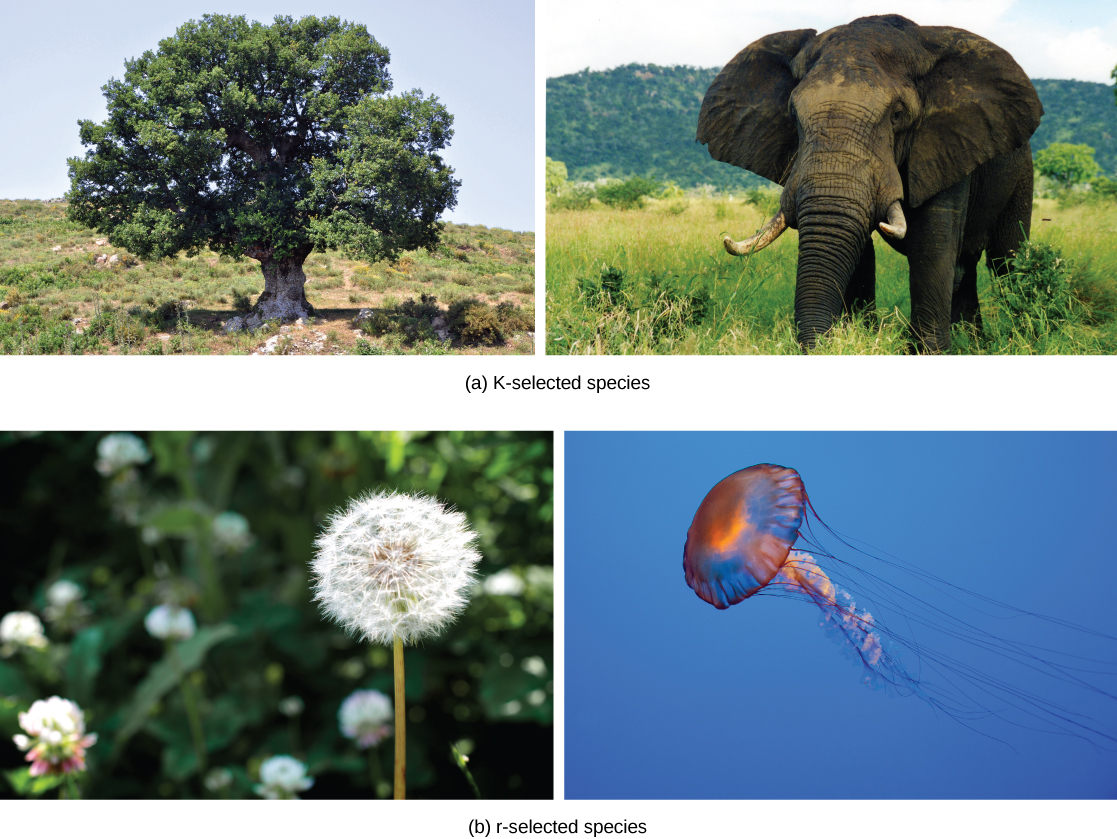
In contrast, r-selected species have a large number of small offspring (hence their r designation (Table \(\PageIndex{1}\)). This strategy is often employed in unpredictable or changing environments. Animals that are r-selected do not give long-term parental care and the offspring are relatively mature and self-sufficient at birth. Examples of r-selected species are marine invertebrates, such as jellyfish, and plants, such as the dandelion (Figure \(\PageIndex{3}\)b). Dandelions have small seeds that are wind dispersed over long distances. Many seeds are produced simultaneously to ensure that at least some of them reach a hospitable environment. Seeds that land in inhospitable environments have little chance for survival since their seeds are low in energy content. Note that survival is not necessarily a function of energy stored in the seed itself.
The following statements compare r-selected and K-selected species. Identify the statement that makes an accurate comparison.
- r-selected and K-selected species both have limitations in the amount of energy they can invest in reproduction, so they both use similar strategies.
- r-selected and K-selected species both have limitations in the amount of energy they can invest in reproduction, but they use completely different strategies.
- r-selected and K-selected species use similar reproductive strategies but r-selected species require less energy to reproduce than K-selected species.
- r-selected and K-selected species use different reproductive strategies because r-selected species require less energy to reproduce than K-selected species.
- Answer
-
b. r-selected and K-selected species both have limitations in the amount of energy they can invest in reproduction, but they use completely different strategies.
Modern Theories of Life History
The r- and K-selection theory, although accepted for decades and used for much groundbreaking research, has now been reconsidered, and many population biologists have abandoned or modified it. Over the years, several studies attempted to confirm the theory, but these attempts have largely failed. Many species were identified that did not follow the theory’s predictions. A highly unpredictable environment can lead to plasticity, in which individual organisms can shift along the spectrum of r-selected vs. K-selected life histories to suit the environment. Furthermore, the theory ignored the age-specific mortality of the populations which scientists now know is very important. New demographic-based models of life history evolution have been developed which incorporate many ecological concepts included in r- and K-selection theory and population age structure and mortality factors. These are discussed in the next sections.
Salmon is an essential resource to many First Nations people and communities in BC. Salmon is found in almost every part of BC, with the exception of northeast regions that are in the Peace and McKenzie River watersheds. For thousands of years, First Nations peoples have developed an intricate understanding and knowledge about salmon, including the interconnectedness of salmon with other life forms. Before contact, salmon was a foundational trade item, contributing significantly to the economy and social structure of First Nations societies. Salmon helped to ensure the sustenance of large, vibrant populations of people. First Nations developed a myriad of technologies to capture and preserve the salmon. The value and respect that the people had for the salmon is reflected in the representation of salmon in traditional stories, songs, dances, and art throughout BC.
The Pacific salmon life cycle depends on the species. Some spend hardly any time in natal streams; some spend years. Some mature at two years of age; some mature at five. Some live for only a couple of years; others live for ten. And some, like Steelhead and Cutthroat, can spawn more than once. Despite these variations, we can still make some general observations about the life stages of salmon.
All Pacific salmon are anadromous, which means they start in freshwater (streams, lakes, rivers, etc.), migrate to the ocean, then return home to spawn and die.
Adult spawners often journey for hundreds of miles to return to the waters their parents spawned in, and where they themselves were born. If they make it back, evading all sorts of obstacles—predation, competition, environmental impediments, the males and females court, and ultimately breed. At the crucial moment, the male releases sperm and the female releases eggs… at precisely the same time.
The eggs and sperm float in a fog of milt to the bottom of the stream or lake bed where the female has painstakingly prepared a redd (aka nest), which, covered with gravel, is expected to protect the eggs until they are ready to hatch.
While new salmon are preparing to enter the world, their parents die (usually only days after spawning), making them semelparous. Their bodies remain in the water or along the shore to decay and/or be eaten by other species. In this way, they continue to nourish the environment around them.
Indigenous wooden eagle and salmon sculpture "Capilano Suspension Bridge, West Vancouver, BC" CC-BY-SA Yuta Koike
Adapted from:
- First Nations Education Steering Committee (2019). FNESC/FNSA Secondary Science First Peoples Teacher Resource Guide. Unit 6: Salmon and Interconnectedness. 298 pp. http://www.fnesc.ca/wp/wp-content/uploads/2019/08/PUBLICATION-SCIENCE-FIRST-PEOPLES-Secondary-TRG-2019.pdf
- Pacific Salmon Foundation 2022 (https://psf.ca/)
A 7-minute short about the importance and life cycle of wild Pacific salmon.
A 15-minute video entitled "St'at'imc: The Salmon People"