17.11: Signal Transduction
- Page ID
- 89017
\( \newcommand{\vecs}[1]{\overset { \scriptstyle \rightharpoonup} {\mathbf{#1}} } \)
\( \newcommand{\vecd}[1]{\overset{-\!-\!\rightharpoonup}{\vphantom{a}\smash {#1}}} \)
\( \newcommand{\id}{\mathrm{id}}\) \( \newcommand{\Span}{\mathrm{span}}\)
( \newcommand{\kernel}{\mathrm{null}\,}\) \( \newcommand{\range}{\mathrm{range}\,}\)
\( \newcommand{\RealPart}{\mathrm{Re}}\) \( \newcommand{\ImaginaryPart}{\mathrm{Im}}\)
\( \newcommand{\Argument}{\mathrm{Arg}}\) \( \newcommand{\norm}[1]{\| #1 \|}\)
\( \newcommand{\inner}[2]{\langle #1, #2 \rangle}\)
\( \newcommand{\Span}{\mathrm{span}}\)
\( \newcommand{\id}{\mathrm{id}}\)
\( \newcommand{\Span}{\mathrm{span}}\)
\( \newcommand{\kernel}{\mathrm{null}\,}\)
\( \newcommand{\range}{\mathrm{range}\,}\)
\( \newcommand{\RealPart}{\mathrm{Re}}\)
\( \newcommand{\ImaginaryPart}{\mathrm{Im}}\)
\( \newcommand{\Argument}{\mathrm{Arg}}\)
\( \newcommand{\norm}[1]{\| #1 \|}\)
\( \newcommand{\inner}[2]{\langle #1, #2 \rangle}\)
\( \newcommand{\Span}{\mathrm{span}}\) \( \newcommand{\AA}{\unicode[.8,0]{x212B}}\)
\( \newcommand{\vectorA}[1]{\vec{#1}} % arrow\)
\( \newcommand{\vectorAt}[1]{\vec{\text{#1}}} % arrow\)
\( \newcommand{\vectorB}[1]{\overset { \scriptstyle \rightharpoonup} {\mathbf{#1}} } \)
\( \newcommand{\vectorC}[1]{\textbf{#1}} \)
\( \newcommand{\vectorD}[1]{\overrightarrow{#1}} \)
\( \newcommand{\vectorDt}[1]{\overrightarrow{\text{#1}}} \)
\( \newcommand{\vectE}[1]{\overset{-\!-\!\rightharpoonup}{\vphantom{a}\smash{\mathbf {#1}}}} \)
\( \newcommand{\vecs}[1]{\overset { \scriptstyle \rightharpoonup} {\mathbf{#1}} } \)
\( \newcommand{\vecd}[1]{\overset{-\!-\!\rightharpoonup}{\vphantom{a}\smash {#1}}} \)
\(\newcommand{\avec}{\mathbf a}\) \(\newcommand{\bvec}{\mathbf b}\) \(\newcommand{\cvec}{\mathbf c}\) \(\newcommand{\dvec}{\mathbf d}\) \(\newcommand{\dtil}{\widetilde{\mathbf d}}\) \(\newcommand{\evec}{\mathbf e}\) \(\newcommand{\fvec}{\mathbf f}\) \(\newcommand{\nvec}{\mathbf n}\) \(\newcommand{\pvec}{\mathbf p}\) \(\newcommand{\qvec}{\mathbf q}\) \(\newcommand{\svec}{\mathbf s}\) \(\newcommand{\tvec}{\mathbf t}\) \(\newcommand{\uvec}{\mathbf u}\) \(\newcommand{\vvec}{\mathbf v}\) \(\newcommand{\wvec}{\mathbf w}\) \(\newcommand{\xvec}{\mathbf x}\) \(\newcommand{\yvec}{\mathbf y}\) \(\newcommand{\zvec}{\mathbf z}\) \(\newcommand{\rvec}{\mathbf r}\) \(\newcommand{\mvec}{\mathbf m}\) \(\newcommand{\zerovec}{\mathbf 0}\) \(\newcommand{\onevec}{\mathbf 1}\) \(\newcommand{\real}{\mathbb R}\) \(\newcommand{\twovec}[2]{\left[\begin{array}{r}#1 \\ #2 \end{array}\right]}\) \(\newcommand{\ctwovec}[2]{\left[\begin{array}{c}#1 \\ #2 \end{array}\right]}\) \(\newcommand{\threevec}[3]{\left[\begin{array}{r}#1 \\ #2 \\ #3 \end{array}\right]}\) \(\newcommand{\cthreevec}[3]{\left[\begin{array}{c}#1 \\ #2 \\ #3 \end{array}\right]}\) \(\newcommand{\fourvec}[4]{\left[\begin{array}{r}#1 \\ #2 \\ #3 \\ #4 \end{array}\right]}\) \(\newcommand{\cfourvec}[4]{\left[\begin{array}{c}#1 \\ #2 \\ #3 \\ #4 \end{array}\right]}\) \(\newcommand{\fivevec}[5]{\left[\begin{array}{r}#1 \\ #2 \\ #3 \\ #4 \\ #5 \\ \end{array}\right]}\) \(\newcommand{\cfivevec}[5]{\left[\begin{array}{c}#1 \\ #2 \\ #3 \\ #4 \\ #5 \\ \end{array}\right]}\) \(\newcommand{\mattwo}[4]{\left[\begin{array}{rr}#1 \amp #2 \\ #3 \amp #4 \\ \end{array}\right]}\) \(\newcommand{\laspan}[1]{\text{Span}\{#1\}}\) \(\newcommand{\bcal}{\cal B}\) \(\newcommand{\ccal}{\cal C}\) \(\newcommand{\scal}{\cal S}\) \(\newcommand{\wcal}{\cal W}\) \(\newcommand{\ecal}{\cal E}\) \(\newcommand{\coords}[2]{\left\{#1\right\}_{#2}}\) \(\newcommand{\gray}[1]{\color{gray}{#1}}\) \(\newcommand{\lgray}[1]{\color{lightgray}{#1}}\) \(\newcommand{\rank}{\operatorname{rank}}\) \(\newcommand{\row}{\text{Row}}\) \(\newcommand{\col}{\text{Col}}\) \(\renewcommand{\row}{\text{Row}}\) \(\newcommand{\nul}{\text{Nul}}\) \(\newcommand{\var}{\text{Var}}\) \(\newcommand{\corr}{\text{corr}}\) \(\newcommand{\len}[1]{\left|#1\right|}\) \(\newcommand{\bbar}{\overline{\bvec}}\) \(\newcommand{\bhat}{\widehat{\bvec}}\) \(\newcommand{\bperp}{\bvec^\perp}\) \(\newcommand{\xhat}{\widehat{\xvec}}\) \(\newcommand{\vhat}{\widehat{\vvec}}\) \(\newcommand{\uhat}{\widehat{\uvec}}\) \(\newcommand{\what}{\widehat{\wvec}}\) \(\newcommand{\Sighat}{\widehat{\Sigma}}\) \(\newcommand{\lt}{<}\) \(\newcommand{\gt}{>}\) \(\newcommand{\amp}{&}\) \(\definecolor{fillinmathshade}{gray}{0.9}\)When hydrophobic chemical effector molecules, such as steroid hormones, reach a target cell, they can cross the hydrophobic membrane and bind to an intracellular receptor to initiate a response. When large effector molecules (e.g., protein hormones) or highly polar hormones (e.g., adrenalin) reach a target cell, they can’t cross the cell membrane. Instead, they bind to transmembrane protein receptors on cell surfaces. A conformational change initiated on the extracellular domain of the membrane receptor protein induces further allosteric change on its cytoplasmic domain. A sequential series of molecular events then converts information delivered by the external effector into intracellular information, a process called signal transduction. A general outline of signal-transduction is illustrated below in Figure 17.29.

Many effects of signal transduction are mediated by a sequence, or cascade of protein phosphorylations catalyzed by protein kinases inside the cell, all of which share some common features as described in the elegant YouTube video at Cell Messaging by Signal Transduction. Here we will consider G-Protein-linked and enzyme-linked receptors.
315-2 Introduction to Signal Transduction
17.11.1 G-Protein-Mediated Signal Transduction by PKA (Protein Kinase A)
Figure 17.30 illustrates seven steps of G-protein-mediated signal transduction.

GTP-binding proteins (G-Proteins) transduce extracellular signals by inducing synthesis of second-messenger molecules in the cells. When hormones or other effector (signal) molecules bind to a membrane receptor, an allosteric change on the extracellular domain of the receptor is transmitted to the cytoplasmic domain of the receptor, increasing its affinity for trimeric G-proteins embedded in the cytoplasmic surface of responsive cell membranes. G-protein trimers consist of \(\alpha\) \(\beta\) and \(\gamma\) subunits. The receptor changes shape upon binding its effector signal molecule (steps 1 and 2). In this altered conformation, the receptor recognizes and binds to the G-protein trimer (step 3). Upon the binding of the trimer to the receptor, GTP displaces GDP on the \(\alpha\) subunit of the G-protein (step 4). After a conformational change in the \(\alpha\) subunit, it dissociates from the \(\beta\) and \(\gamma\) subunits (step 5). In this illustration, the GTP-\(\alpha\) subunit can now bind to a transmembrane adenylate cyclase enzyme (step 6). Finally, the initial extracellular chemical signal is transduced to an intracellular response, involving a second-messenger molecule (step 7). In this case, the second messenger is cAMP.
316-2 G-Protein Signal Transduction
The well-known fight-or-flight response to adrenalin in the liver cells of higher animals is a good example of a cAMP-mediated cellular response. Once formed, cAMP binds to and activates protein kinase A (PKA), setting off a phosphorylation cascade that leads to a physiological response. Details of a G-protein-mediated fight-or-flight signal amplification cascade are shown in Figure 17.31.

After activation of adenylate cyclase (steps 1 and 2 above), cAMP is synthesized. A cAMP binds to each of two of the four subunits of an inactive PKA (step 3). An allosteric change dissociates the tetramer into two cAMP-bound inert subunits and two active PKA subunits (step 4). Each active PKA enzyme catalyzes the phosphorylation and activation of the enzyme phosphorylase kinase (step 5). In step 6, phosphorylase kinase catalyzes glycogen phosphorylase phosphorylation.
In the cascade, activation of just a few enzyme molecules at the top of the sequence results in the activation of multiple subsequent enzymes, resulting in a much-amplified cellular response, referred to as an amplification cascade. The result can be an immediate dramatic response. At the end of the fight-or-flight phosphorylation cascade, a now-active glycogen phosphorylase catalyzes the hydrolysis glycogen to glucose-1-phosphate (step 7). This results in a rapid retrieval of free glucose from liver cells into the circulation.
See again how this works by reviewing the conversion of glucose-1-phosphate (G-1-P) to G-6-P in glycolysis and its fate in gluconeogenesis. Of course, the increase in circulating glucose provides the energy for the fight-or-flight decision.
317 G-Protein Activation of Protein Kinase A: a Fight-or-Flight Response
In addition to activating enzymes that break down glycogen, cAMP-activated PKA mediates cellular responses to different effectors, resulting in a phosphorylation cascade leading to several results:
- Activation of the enzymes catalyzing glycogen synthesis
- Activation of the lipases that hydrolyze fatty acids from triglycerides
- Microtubule assembly • Microtubule disassembly
- Mitogenic effects (activation of the enzymes of replication)
- Activation of transcription factors, which increases or decreases gene expression
Of course, when a cellular response is no longer needed by the organism, it must stop producing the signal molecules (hormones or other effectors). As their levels drop, effector molecules dissociate from their receptors and the response stops. This is all possible because the binding of signals to their receptors is freely reversible!
We take advantage of the power of amplification cascades in medicine. How does this happen on TV and/or in real life?
17.11.2 Signal Transduction using PKC (Protein Kinase C)
A different G-protein-mediated signaling pathway activates a protein kinase C (PKC). The activation of PKC is illustrated in Figure 17.32 (below).
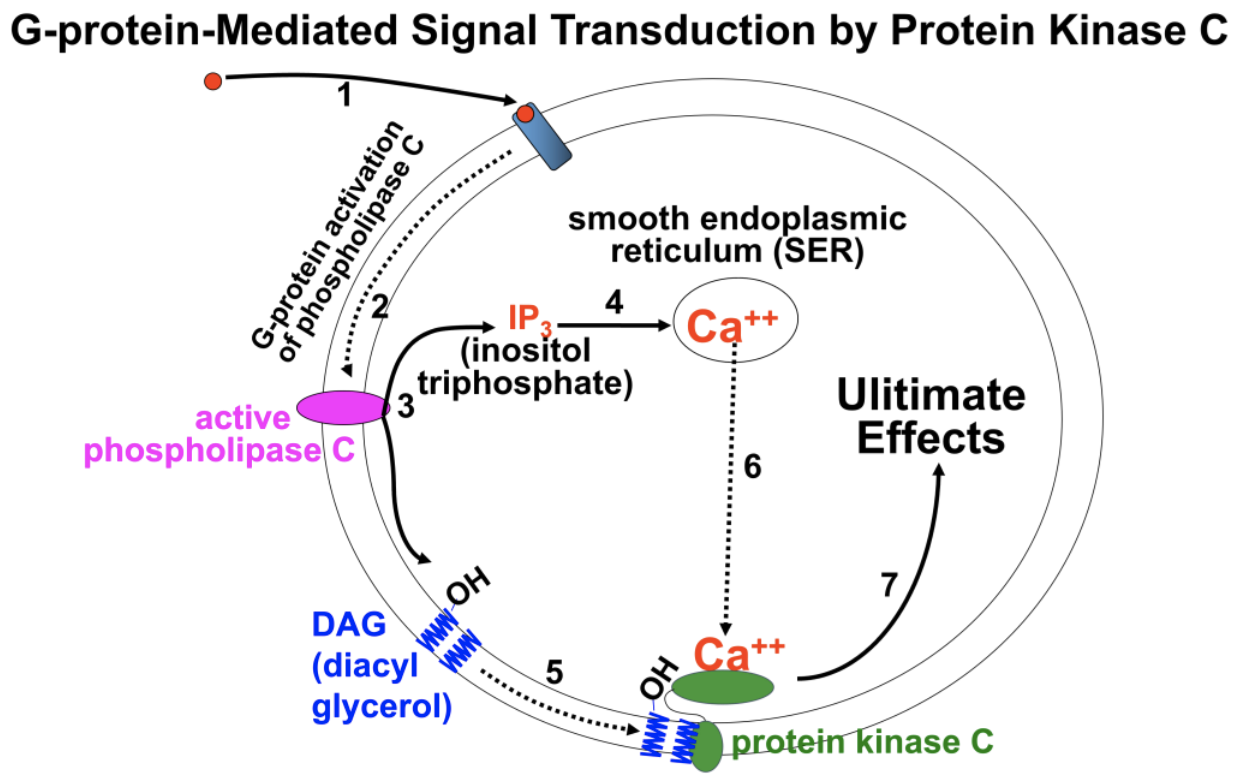
The role of G-proteins is similar for PKA- and ‘PKC-signal transduction’, but PKC activation does not involve cAMP and requires two intervening cellular responses to an effector. The net result is to generate different second messengers. These in turn activate different phosphorylation cascades. Like PKA, PKC-mediated signal transduction also amplifies the cell’s first molecular response to the effector. Here are the steps:
- An effector-signal molecule binds to its receptor.
- The effector-bound receptor now binds the G-protein trimer, the GTP for GDP swap on the alpha subunit of the trimer, dissociating the beta-m and gamma-subunits from the trimer. The receptor-bound GTP-alpha- subunit activates an inactive membrane-bound phospholipase C enzyme.
- Active phospholipase C catalyzes formation of cytosolic ‘inositol triphosphate’ (‘\(\rm IP_3\)‘) and of membrane-bound ‘diacyl glycerol’ (‘DAG’), two of those other intracellular second messenger molecules.
- \(\rm IP_3\) interacts with receptors on smooth endoplasmic reticulum, causing the release of sequestered \(\rm Ca^{++}\) ions into the cytoplasm.
- DAG finds and binds PKC in the membrane.
- The \(\rm Ca^{++}\) ions also fin and bind PKC, completing activation of the ‘protein kinase C’ (‘PKC’) enyme.
- Activated PKC initiates a phosphorylation-amplification cascade leading to cell-specific responses.
318-2 G-Protein Activation of Protein Kinase C and Phospholipase C
PKC-mediated effects include the following:
- Neurotransmitter release
- Hormone (e.g., growth hormone, luteinizing hormone, and testosterone) secretion, leading to cell growth, division, and differentiation
- Glycogen hydrolysis, fat synthesis
Additional independent phospholipase C effects include these:
- Liver glycogen breakdown
- Pancreatic amylase secretion
- Platelet aggregation
PKA and PKC are both serine-threonine kinases, which place phosphates on serine or threonine in target polypeptides. Let’s consider tyrosine kinases next.
Research and explore the details of some of these effects.
17.11.3 Receptor Tyrosine Kinase-Mediated Signal Transduction
The intracellular activity of tyrosine kinase receptors is in the cytoplasmic domain of the receptor itself. When bound to its effector, receptor-kinases catalyze the phosphorylation of specific tyrosine amino acids in target proteins. While studying the actions of nerve growth factor (NGF) and epidermal growth factor (EGF) in stimulating growth and differentiation of nerves and skin, Stanley Cohen and Rita Levi-Montalcini discovered the EGF receptor, the first enzyme-linked tyrosine kinase, and they won the 1986 Nobel Prize in Physiology or Medicine for their discovery! Watch the animation of receptor-kinase signal transduction at the following link (a description is provided in the next few paragraphs).
319 Receptor-Kinase Signal Transduction
Monomer membrane receptor kinases dimerize when they bind effector ligands, activating kinase domains that catalyze multiple cross-phosphorylation of the monomers. The phosphorylated dimer kinases recruit and phosphorylate yet other cytoplasmic proteins to continue the response pathway. A typical response to EGF and NGF signaling is mitogenic. Not surprisingly, the mutations correlated with cancer cells often lie in mitogenic signaling pathways that lead to cell proliferation (growth and division). Cancer-causing genes, or oncogenes, were first discovered in viruses. But as humans, we own our own oncogenes!
For being the first to show that eukaryotic cells were the origin of a chicken retrovirus (the Rous sarcoma virus), J. Michael Bishop and Harold Varmus earned the 1989 Nobel Prize in Physiology or Medicine. Oncogenes turn out to be mutations of animal genes for proteins involved in mitogenic signal transduction pathways.
Under normal circumstances, mitogenic chemical signals binding to a receptor tyrosine kinase induces target cells to begin dividing (see the Youtube video link above). One of the end-products of a receptor kinase-mediated phosphorylation cascade is MAP kinase (mitogen-activated protein kinase). MAP kinase enters the nucleus where it catalyzes the phosphorylation of transcription factors and other nuclear proteins, that lead to cell proliferation and differentiation, as shown in Figure 17.33.

What might the “other nuclear proteins” in the illustration be?
The Ras protein-mediated activation of a phosphorylation cascade leading to the MAP kinase is an example of such a signal transduction pathway. It plays a central role in many receptor-kinase signaling events. The Ras gene was one of those originally discovered as an oncogene, the mutation of which leads to uncontrolled cell division (i.e., cancer). Ras gene protein activity may in fact be responsible for up to 30% of all cancers!
320-2 The RAS Oncogene: Its Normal Mitogenic Effects and Cancer
What kind of MAP-kinase mutation might cause cancer?