17.9: Moving and Sorting Proteins to Their Final Destinations
- Page ID
- 89015
Like proteins packaged in RER, those made in the cytoplasm go to different destinations before they become functional. What’s more, cells can truly multitask (unlike most of us!), handling the synthesis and distribution (or secretion) of many proteins at the same time! Let’s begin with a look at the sorting mechanisms for proteins sequestered by the endomembrane system.
17.9.1 Traffic on the Endomembrane Highway
We have already seen that, once packaged in the RER cisternae, proteins begin posttranslational modification (e.g., by “core glycosylation”). Transport vesicles that bud off from the RER carry packaged and membrane proteins to the cis vesicles of the Golgi apparatus. There, vesicle fusion is mediated by the recognition of complementary integral membrane proteins embedded in the two membranes. Later, such packaged proteins are sorted to different organelles or to the plasma membrane. Protein sorting starts as proteins move from the cis to the trans face of the Golgi vesicles, where specific sorting proteins associate with different packaged proteins in the trans Golgi vesicles. The packaged proteins then sort to vesicles that bud off from trans Golgi stacks. These vesicles move to their final destinations, recognizing and then fusing with appropriate membranes. James E. Rothman, Randy W. Schekman, and Thomas C. Südhof won the 2013 Nobel Prize in Physiology or Medicine for their studies of the regulation of vesicle traffic (check out 2013 Physiology or Medicine Nobel Prize for more information). Let’s follow some proteins in and on RER membranes through the cell (also animated at Events of Protein Trafficking) from the RER to their cellular destinations, as summarized in Figure 17.21.
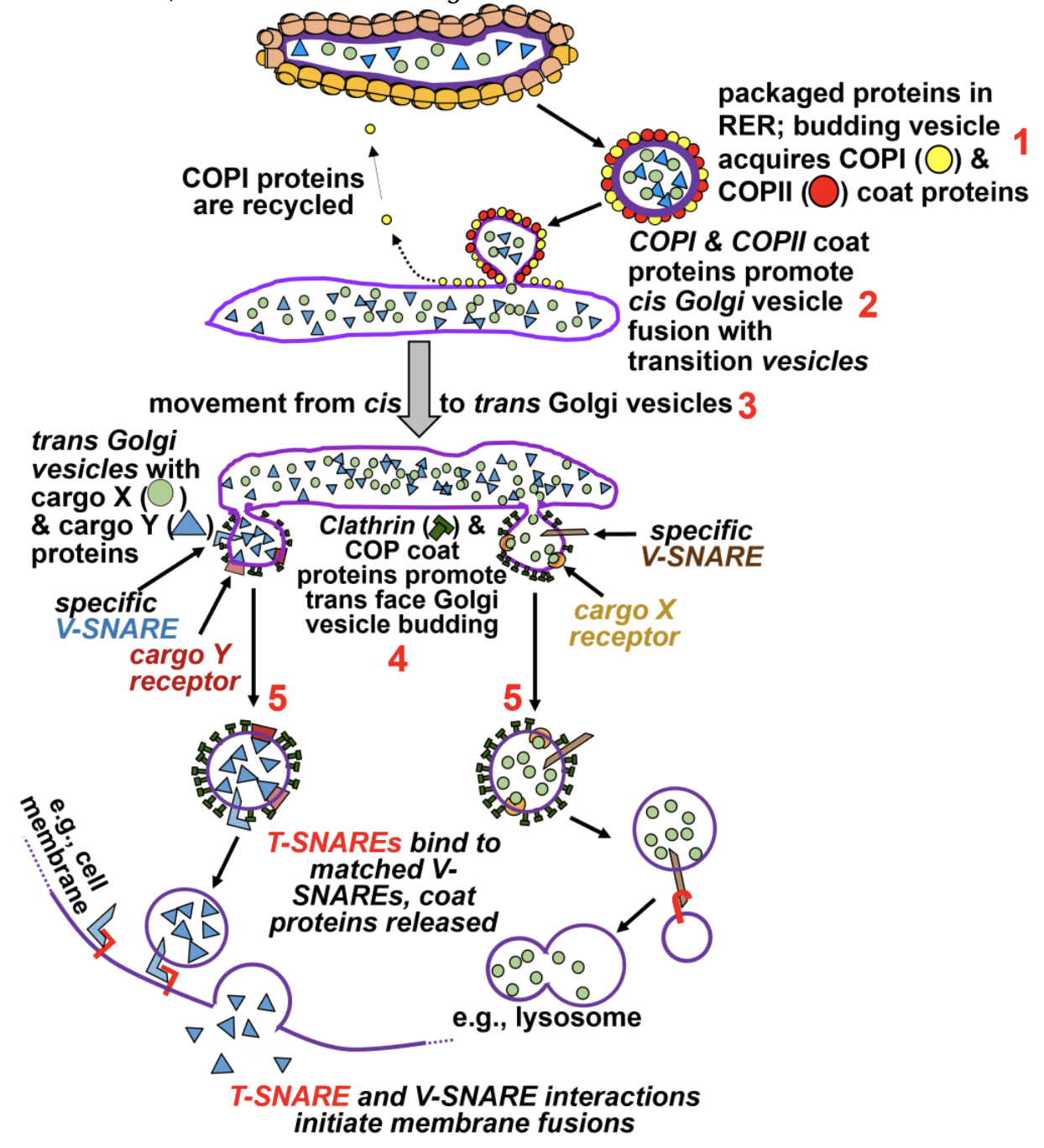
Here are the steps to follow the traffic of protein sorting shown in the illustration:
- Transition vesicles, carrying their mix of packaged proteins, bud off from the RER with the help of COPI and COPII coat proteins, dissociating from ribosomes. Transition vesicles, however, remain associated with the COP proteins.
- These vesicles fuse with cis Golgi vesicles, a process also mediated by COP proteins. COPI proteins detach during or after fusion, recycling back to the RER.
- Packaged proteins and membrane proteins are further processed as they pass through the Golgi vesicle stack (e.g., undergoing terminal glycosylation).
- A key step in sorting packaged proteins occurs as vesicles bud off from the trans Golgi face with the help of clathrin, other COP proteins, and specific cargo-receptor proteins. The cargo-receptor proteins recruit specific packaged proteins (now called cargo proteins) and enrich them in their nascent vesicles. V-SNARE (for vesicle-SNARE) proteins also associate with the vesicles, guiding them to their ultimate destination.
When V-SNARE proteins on their vesicles next bind to complementary Target-Snare (T-SNARE) proteins on receiving membranes, the two membranes fuse, at which point the different types of vesicle part ways. Some vesicles follow a pathway to organelles, fusing with lysosomes or similar vesicles along the way to stock up on appropriate enzymes and other required protein content. Coat proteins come off the fusing vesicle and are recycled, while vesicle contents are transferred into the next generation vesicle. Those containing secretory proteins typically fuse to form larger secretory vesicles (or secretion vesicles). These will next fuse with the plasma membrane, releasing their contents to the extracellular fluid. Once again, coat proteins and clathrin dissociate from the secretory vesicle during this fusion. Secretory vesicles do not always release their contents immediately, but often remain in the cytoplasm, storing their proteins until cells are signaled to release them.
Other players have been left out of this discussion, notably those that hydrolyze nucleotide triphosphates to provide the energy for protein trafficking. In addition, you may recognize clathrin as a molecular player that also functions in receptor-mediated endocytosis. Maybe that’s not a surprise! After all, endocytosis is at least partly molecular traffic in the opposite direction of vesicle formation and secretion.
Cargo receptors that sort packaged proteins raise questions! Where do they come from, and why do they become active when they do?
17.9.2 Nuclear Protein Traffic
We saw earlier that large molecules (mRNAs, tRNAs) and even whole particles (i.e., ribosomal subunits) can cross the nuclear envelope through nuclear pores. But what about those large proteins that are destined to enter the nucleus?
It turns out that these proteins have nuclear localization signals rich in positively charged amino acids (lysine, proline), which enable binding to the negatively charged domain of a nuclear transport receptor protein in the cytosol (Figure 17.22).

As the complex of the two proteins approaches a nuclear pore, it interacts with nuclear pore fibrils, causing the pore to open. The two bound proteins then cross the double membrane of the nuclear envelope, where they accumulate against a concentration gradient. This active transport comes from ATP hydrolysis as the nuclear proteins enter the nucleus.
17.9.3 Mitochondrial Protein Traffic
Recall that mitochondria contain their own genome and translational machinery. Thus, they transcribe RNAs and translate proteins of their own. But genes in the nucleus also encode many of the proteins found in mitochondria. Figure 17.23 (below) illustrates how the mitochondria import these proteins.
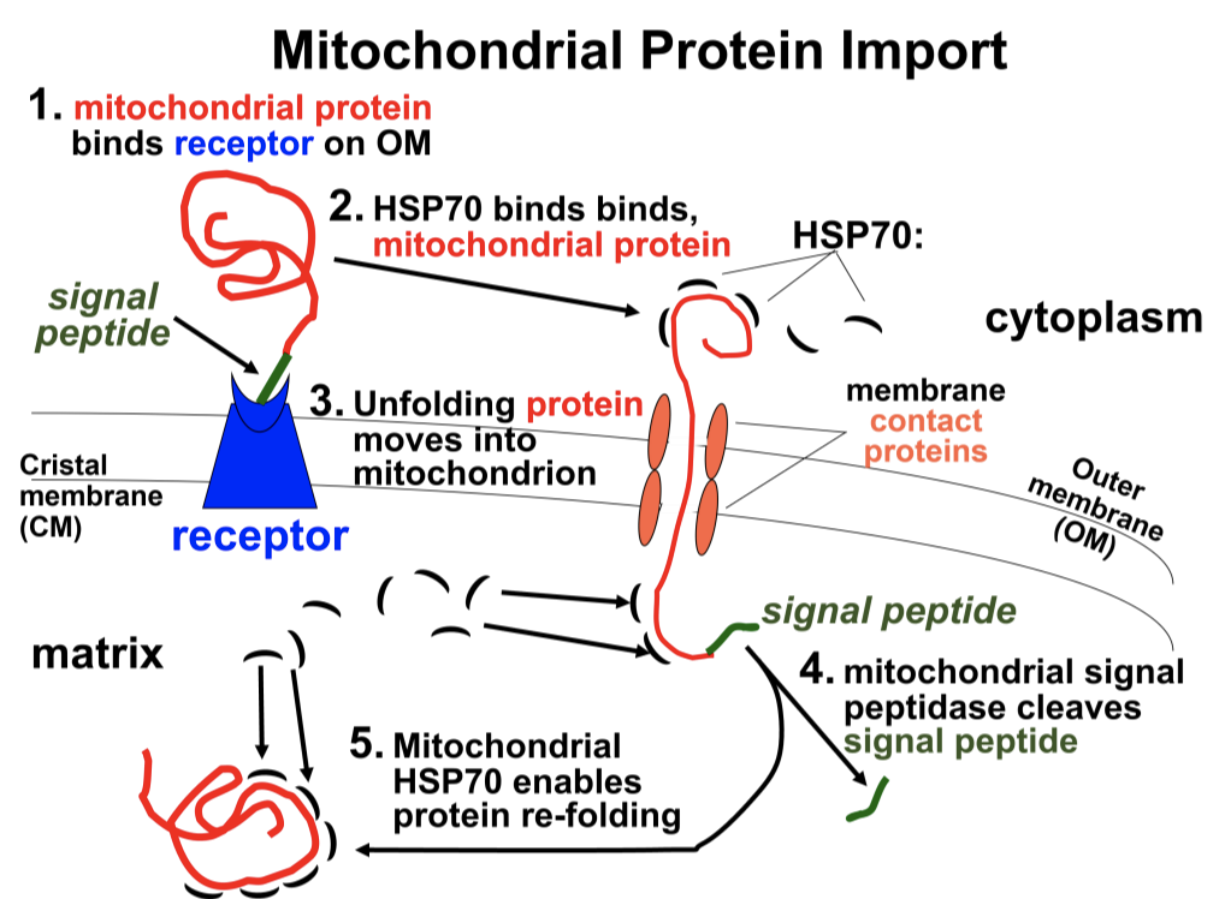
Unlike the cotranslational packaging of proteins by the RER, mitochondrial protein transfer is posttranslational. This means that mitochondrial proteins formed in the cytoplasm have already folded into a tertiary structure. However, the folded protein exposes an N-terminal signal peptide on its surface that recognizes and binds to a receptor protein at the outer mitochondrial membrane (1). The receptor protein spans both the mitochondrial outer membrane (OM) and the cristal membrane (CM). The receptor protein delivers the protein to be imported to membrane-contact proteins, which also span both mitochondrial membranes (2). The membrane-contact proteins act as channels or pores, through which a mitochondrial protein made in the cytoplasm will cross into the mitochondrial matrix. But there is a problem: the folded protein cannot cross the membrane by itself, precisely because it is folded!
The entry of completed proteins into a mitochondrion requires chaperone proteins—in this case, the HSP70 (heat-shock 70). HSP70 controls unfolding of the mitochondrial protein as it traverses the membranes into the matrix (3). Upon removal of the signal peptide by a mitochondrial signal peptidase (4) another HSP70 molecule resident in the matrix facilitates refolding of the protein into a biologically active shape (5). Recall that HSPs were initially discovered as 70 Kd proteins that accumulated in heat-stressed organisms!
309 Protein Traffic to Nuclei and Mitochondria
How do signal peptides mitochondrial cytoplasmic proteins differ from those of proteins to be secreted from the cell?