Marc_W2024_Reading_4
- Page ID
- 133174
\( \newcommand{\vecs}[1]{\overset { \scriptstyle \rightharpoonup} {\mathbf{#1}} } \)
\( \newcommand{\vecd}[1]{\overset{-\!-\!\rightharpoonup}{\vphantom{a}\smash {#1}}} \)
\( \newcommand{\id}{\mathrm{id}}\) \( \newcommand{\Span}{\mathrm{span}}\)
( \newcommand{\kernel}{\mathrm{null}\,}\) \( \newcommand{\range}{\mathrm{range}\,}\)
\( \newcommand{\RealPart}{\mathrm{Re}}\) \( \newcommand{\ImaginaryPart}{\mathrm{Im}}\)
\( \newcommand{\Argument}{\mathrm{Arg}}\) \( \newcommand{\norm}[1]{\| #1 \|}\)
\( \newcommand{\inner}[2]{\langle #1, #2 \rangle}\)
\( \newcommand{\Span}{\mathrm{span}}\)
\( \newcommand{\id}{\mathrm{id}}\)
\( \newcommand{\Span}{\mathrm{span}}\)
\( \newcommand{\kernel}{\mathrm{null}\,}\)
\( \newcommand{\range}{\mathrm{range}\,}\)
\( \newcommand{\RealPart}{\mathrm{Re}}\)
\( \newcommand{\ImaginaryPart}{\mathrm{Im}}\)
\( \newcommand{\Argument}{\mathrm{Arg}}\)
\( \newcommand{\norm}[1]{\| #1 \|}\)
\( \newcommand{\inner}[2]{\langle #1, #2 \rangle}\)
\( \newcommand{\Span}{\mathrm{span}}\) \( \newcommand{\AA}{\unicode[.8,0]{x212B}}\)
\( \newcommand{\vectorA}[1]{\vec{#1}} % arrow\)
\( \newcommand{\vectorAt}[1]{\vec{\text{#1}}} % arrow\)
\( \newcommand{\vectorB}[1]{\overset { \scriptstyle \rightharpoonup} {\mathbf{#1}} } \)
\( \newcommand{\vectorC}[1]{\textbf{#1}} \)
\( \newcommand{\vectorD}[1]{\overrightarrow{#1}} \)
\( \newcommand{\vectorDt}[1]{\overrightarrow{\text{#1}}} \)
\( \newcommand{\vectE}[1]{\overset{-\!-\!\rightharpoonup}{\vphantom{a}\smash{\mathbf {#1}}}} \)
\( \newcommand{\vecs}[1]{\overset { \scriptstyle \rightharpoonup} {\mathbf{#1}} } \)
\( \newcommand{\vecd}[1]{\overset{-\!-\!\rightharpoonup}{\vphantom{a}\smash {#1}}} \)
Learning Objectives Associated with Winter_2024_Bis2A_Facciotti_Reading_04GC.15 Define and correctly use the acid dissociation constant, Ka. |
pKa 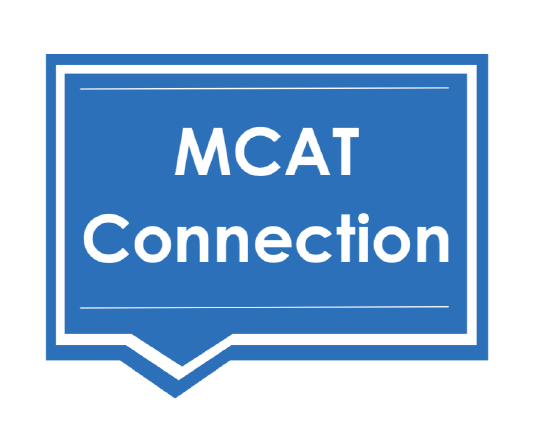
pKa is defined as the negative log10 of the dissociation constant of an acid, its Ka.
pKa = -log10[Ka]
The pKa is a quantitative measure of how readily an acid gives up a proton to a solution and is thus a measure of the "strength" of the acid. Strong acids have a small pKa, weak acids have a larger pKa.
As noted, the carboxylic acid functional group R-COOH is found in many biomolecules. This functional group has a pKa between 2-4 in aqueous solution and is considered a weak acid. At many biologically relevant pH values, the carboxylic acid only partially dissociates into H+ cations and R-COO- anions. In a population of molecules containing the carboxylic acid functional group, it is not uncommon - at biologically relevant pH values - to simultaneously find molecules in both the protonated (R-COOH) and deprotonated (R-COO-) forms. By contrast, HCl (hydrogen chloride), a common strong acid, has a pKa << 0. This means that it will fully dissociate into H+ and Cl- at all biologically relevant pH values. HCl is a strong acid and will almost never be found in the protonated form.
One of the key ways we depict the difference between a strong acid or base and a weak acid or base in chemical equations is the use of a single arrow (strong acid/base) versus a double arrow (weak acid/base). The full dissociation of proton(s) from strong acids at equilibrium means that the likelihood of protons returning to the molecule they dissociated from is very small. The reaction goes mostly one way, hence the single arrow. In weak acids, the likelihood of the forward reaction happening is more similar to the likelihood of the reverse reaction happening. The consequence of this is partial dissociation at equilibrium, hence the double arrow in the chemical equation.
Figure 1. An example of strong acids, weak acids, strong bases, and weak bases in their biologically relevant protonated and deprotonated states. The value of their pKa is shown on the left. Attribution: Marc T. Facciotti.
In General Biology we ask you to relate pH and pKa to each other when discussing the protonation state of a weak acid or weak base in, for example, amino acids. How can we use the information given in this module to answer the question: Will the functional groups on the amino acid Glutamate be protonated or deprotonated at a pH of 2, at a pH of 8, or at a pH of 11?
To answer this kind of question, we need to create a relationship between pH and pKa. The relationship between pKa and pH is mathematically represented by the Henderson-Hasselbach equation shown below, where [A-] represents the deprotonated form of the acid and [HA] represents the protonated form of the acid.
Figure 2. The Henderson-Hasselbach equation
This equation has three core "parts":
1. The pH;
2. The pKa; and
3. log10[A-]/[HA].
Part 1 tells you about the proton concentration. Part 2 tells you about a property of the acid - how likely it is to "give up" its protons to solution. Part 3 tells you about how much of the acid is in its deprotonated form [A-] and in its protonated form [HA]. In most experimental conditions we usually assume that the pKa doesn't change (after all, it's a property of the molecule). So, this equation tells us that the pH and the ratio of deprotonated [A-] and protonated [HA] form of the acid are related to one another. If you are able to independently control the pH by adding more acid or base, you can control the ratio of deprotonated [A-] and protonated [HA] forms of the acid. You can, of course, use the Henderson-Hasselbach equation to solve the problem of knowing the protonation state of Glutamate's functional groups at different pHs. However, we can also develop an intuition about the relationship between these three quantities.
Titrations can help develop an intuitive understanding
Another useful way to develop an intuitive understanding of the relationship between pH, pKa and the protonation states of functional groups is to think about the results of a titration. The titration experiment typically involves the slow, stepwise, and gradual addition of one reagent (e.g. Reagent #1) into a mixture of other molecules (e.g. Solution #1). The experimenter slowly adds Reagent #1 (independent variable) into Solution #1 and makes observations of one or more properties (dependent variables) of the mixture after every step. Depending on the reagents, observations can be things like a change in color, change in viscosity, change in taste, or change in pH. The experimental data is usually plotted in a graph with increasing Reagent #1 on the x-axis and the measured solution property (e.g pH, color, viscosity, etc.) plotted on the y-axis.
Interpreting a Titration Graph
Below is a graph showing the titration of a solution of acetic acid (Solution #1). Acetic acid, the acid found in vinegar, can also be represented by the chemical formula CH3COOH and contains a single carboxyl functional group. In this experiment, the acetic acid is titrated with a base, represented as “OH” (Reagent #1) in the figure. The graph when read from left to right reports the change in pH of the solution when base (OH) is slowly added. Examining the graph, you see three phases of pH change:
(a) Adding between 0 to about 3 equivalents of OH causes a rapid rise of the pH. At the molecular level, this rise can be explained by each additional base equivalent reacting with H3O+ to create two neutral water molecules. This reduces the [H+] and therefore increases the pH. At this pH, the molecule (CH3COOH) has the "strength" to hold onto its H+ ions.
(b) When between 3 and 7 equivalents of OH are added, the pH hardly changes; it stays stable around a value equivalent to the pKa of CH3COOH (4.76). In this zone of the chart, acetic acid molecules start to "let go" of their H+ ions when OH is added. Each OH added “grabs” a proton from solution which is replaced by a proton “released” by CH3COOH molecules in solution. When the pH = pKa (around 5 equivalents of OH-), the Henderson-Hasselbach equation tells us that 50% of the acetic acid molecules in solution are protonated and 50% are deprotonated. As more equivalents of OH are added, more acetic acid molecules become deprotonated until all acetic acid molecules become deprotonated.
(c) Once this happens, at 7 or more equivalents of OH, no more protonated molecules are available to neutralize added OH. Adding more OH therefore starts rapidly raising the pH again.
Building a mental picture of this process can be a powerful tool to help you intuitively solve the problem that started this discussion. If you want to intuit the protonation state of a functional group, with a known pKa, at a given pH, you can start by imagining the situation when the pH = pKa. At this point, you know that the functional group is 50% protonated and 50% deprotonated. Therefore, if the pH in question is below the pKa moving from the pH = pKa to the target pH requires adding protons to the solution. When the solution becomes more acidic, there will be more H+ ions ready to protonate deprotonated functional groups and thus increasing the amount of protonated functional groups. On the titration curve below, this is like starting at the center of the flat portion of the curve and moving to the left. When the target pH is higher than the pKa the solution must become more basic in the shift from the starting pH to the target pH. Here, there will be fewer H+ ions than at the beginning, meaning that the functional groups will start to deprotonate thus increasing the proportion of unprotonated functional groups.
Figure 3. This graph depicts the protonation state of acetic acid as the pH changes. At a pH below the
This movie provides a visual demonstration of the explanation given above.
We close this section by returning to the original question: Will the functional groups on the amino acid glutamate be protonated or deprotonated at a pH of 2, at a pH of 8, at a pH of 11? In the example of acetic acid above, we developed an understanding of how to relate the protonation state of one functional group to its pKa and the pH of the solution its in to the balance/ratio of protonated to deprotonated state for a single functional group.
In biology, however, you will often be interested in the behavior of molecules with multiple functional groups, each with their own pKa values. An free amino acid like glutamate has three different functional groups, each with their own pKa. So, answering the question about the protonation state of glutamate at different pH values requires you to assess the protonation state and deprotonation state of each of those functional groups independently. The figure below shows a titration experiment for amino acid glutamate. Like the plot above, it shows the relationship between the pH and pKa for each of the three ionizable functional groups of glutamate. The protonation state of functional group can be evaluated independently at a specified pH value to ultimately determine the protonation state of the whole molecule.
Figure 4. This graph depicts the protonation state of glutamate as the pH changes. At a pH below the pKa for each functional group on the amino acid, the functional group is protonated. At a pH above the pKa for the functional group it is deprotonated. If the pH equals the pKa, the functional group is 50% protonated and 50% deprotonated.
Attribution: Ivy Jose
Interactive Exercise
Quick Reference: pKa and pH Comparison Chart
pKa | pH |
pKa = -log10[Ka] | pH= −log10[H+] |
|
|
|
|
• While pKa depends largely on the physical properties of a molecule, it can also be influenced/changed by the local environment the molecule finds itself in. |
|
PRACTICE POST-GUIDE
General Practice
3. Why: Many biologically important reactions involve transferring an ion or a charged group from one molecule to another. The pH can greatly influence the rate of such reactions. The pKa gives you an idea about the reactivity of a particular side chain or functional group on a biomolecule. Amino acids are key biomolecules that undergo protonation/deprotonation. If you are given the structure of an amino acid, you should be able to identify its different functional groups and the properties of the amino acid at various pH values if given the pKa values.
How to practice: So, go through each of the amino acids (find their structures in the readings or online) and identify their functional groups and use the reported pKa values to determine whether the functional group should be protonated or deprotonated at different pH values.
Exercise #3 is good practice for the following learning goals: “GC.33 Define and correctly use pKa. GC.32 Explain the relationship between pKa and pH and apply these concepts to predict the protonation state of a functional group at different pH values.”
4. Why: Once you understand how to read and interpret pH, the next step is to learn how to apply this concept in the context of a biological phenomena. This exercise is intended to start you thinking about how a change in pH can influence the function of biomolecules, in this case the transport of oxygen by the protein hemoglobin. You should start thinking about how pH can influence the protonation state of functional groups (in this case on proteins), how the concept of equilibrium relates to this and thereby start to create a mental picture of functional groups switching between functional protonated and deprotonated states.
How to practice: Let’s look at a figure from a sample MCAT question published by the MCAT board that shows the levels of oxygen bound to hemoglobin at three different pH values. Hemoglobin is a protein in red blood cells. It binds oxygen in the lungs and releases that oxygen to tissues that need it.
The first thing to do is to read the graph. What is plotted on the x-axis? What is plotted on the y-axis? For the sake of simplicity, let’s just say that pO2 is related to oxygen concentration in the blood. The larger the pO2, the greater the concentration.
According to the graph, is the binding of O2 to hemoglobin dependent on pH? If so, what is the relationship between binding affinity and pH? We will call binding affinity a measure of how strongly something binds to something else.
Now try to answer the multiple-choice question. Once you have done that, try to use your knowledge of how functional groups behave at different pH levels to propose a possible change to hemoglobin that might be driving this behavior.
Exercise #4 is excellent practice for learning goal: “MS.4 Predict the potential influence of changes of pH on the structure and function of biomolecules.”
5. Why: This exercise takes the theme from exercise #4 and extends it a bit more by requiring you to abstract the the protein-small molecule interaction a little bit and to use data predict it’s behavior in different areas of the digestive tract. It’s really the same problem that you’ve solved a number of times already but requires that you can think about what is happening to the drug/proteins more generically. Being able to take a concept and apply/transfer your understanding/skill in different situations is a sign you are beginning to master the core idea. This transfer of knowledge/skill is one of the major goals we have for you as you become more experienced practitioners.
How to practice: The figure on the next page is from the lecture slides. What is the pKa of the functional group that could be changing protonation state and influencing binding? Let’s imagine that this SM represents a drug that was delivered in pill form, had no solubility issues, and had a target somewhere in the digestive tract. Use the figure to predict where might be the most likely places to find the target protein. (NOTE: I didn’t make this a practice exam question, but I could turn it into a multiple-choice question easily. This also demonstrates how I can extend the concept and ask you about the same things in different ways).
This exercise starts to move you into the domain of predicting how changes in protonation state of functional groups might influence structure and function and thus is good practice for learning goal: “MS.4 Predict the potential influence of changes of pH on the structure and function of biomolecules.”
6. Why: One of the best ways to practice a skill and convince yourself that you understand it is to invent your own exercises that are similar, yet different, from examples you’ve seen before (like the examples in class, exercises above or exercises you do in discussion). This is NOT easy, even when you have a decent grasp of a topic. If you find this an impossible task, or you find yourself only creating the most basic questions, it’s probably a sign that you could stand some more practice or need more clarification. If you’re struggling, bring your work to office hours and/or arrange to meet your TA/prof. to work through some ideas/examples.
How to practice: Practice constructing scenarios like the ones we talked about in lecture and in the problems above where you find a functional group’s pKa and then play with different pH values. Ask yourself about the protonation state of the functional group. If you like, you can look up pKa values for amino acids (this could serve the double purpose of practicing your ability to recognize amino acids). Make up some imaginary scenarios where changing the pH changes the function of a protein or other molecule and try to explain what is happening.
7. Why: This is a point in the course when many students realize that the material is starting to stack up and some start to feel confused.
How to practice: Make sure to get help if you need it. There are many TAs with office hours to help, and you can go to ANY of them, not just “your” TA. Go to our office hours and workshops and bring your questions.
PRACTICE EXAM QUESTIONS
Try to figure out which learning objective(s) these sample questions are testing.
Question Q4.1
Q4.1 A free amino acid is shown below. What will be the net charge of this molecule in a highly alkaline solution (e.g. pH 13)?
- -2
- -1
- 0
- 1
- 2
|
Functional Group 1 |
Functional Group 2 |
Functional Group 3 |
pka |
3.9 |
9.9 |
2.0 |
Question Q4.2
Q4.2 More practice on pH and pKa in a biological context.
Given the data in the tables, which of the following pairwise interactions between the amino acid side chains would you expect to most strongly stabilize the interaction between two proteins at pH = 9? Assume the amino acids are part of a protein.
Glu pKa of side chain ~ 4.1
|
|
His pKa of side chain ~ 6.0
|
|
Ser
|
|
Arg pKa of side chain ~ 12.5
|
|
- Glu interacts with Glu
- His interacts with Arg
- Arg interacts with Glu
- Ser interacts with Glu
- Arg interacts with Ser
Question Q4.3
Q4.3 This question was on a previous BIS 2A midterm. Like the above question, it asks you to apply the relationship between pH and pKa and to use a reference table to find relevant data to predict the protonation state of a molecule of interest.
Based on the table below, which amino acid R group(s) will have a net positive charge at
pH = 7.0?
- Arginine
- Arginine & lysine
- Histidine & lysine
- Histidine & glutamic acid
- Glutamic acid