25: Light and Photosynthesis
- Page ID
- 113917
\( \newcommand{\vecs}[1]{\overset { \scriptstyle \rightharpoonup} {\mathbf{#1}} } \)
\( \newcommand{\vecd}[1]{\overset{-\!-\!\rightharpoonup}{\vphantom{a}\smash {#1}}} \)
\( \newcommand{\id}{\mathrm{id}}\) \( \newcommand{\Span}{\mathrm{span}}\)
( \newcommand{\kernel}{\mathrm{null}\,}\) \( \newcommand{\range}{\mathrm{range}\,}\)
\( \newcommand{\RealPart}{\mathrm{Re}}\) \( \newcommand{\ImaginaryPart}{\mathrm{Im}}\)
\( \newcommand{\Argument}{\mathrm{Arg}}\) \( \newcommand{\norm}[1]{\| #1 \|}\)
\( \newcommand{\inner}[2]{\langle #1, #2 \rangle}\)
\( \newcommand{\Span}{\mathrm{span}}\)
\( \newcommand{\id}{\mathrm{id}}\)
\( \newcommand{\Span}{\mathrm{span}}\)
\( \newcommand{\kernel}{\mathrm{null}\,}\)
\( \newcommand{\range}{\mathrm{range}\,}\)
\( \newcommand{\RealPart}{\mathrm{Re}}\)
\( \newcommand{\ImaginaryPart}{\mathrm{Im}}\)
\( \newcommand{\Argument}{\mathrm{Arg}}\)
\( \newcommand{\norm}[1]{\| #1 \|}\)
\( \newcommand{\inner}[2]{\langle #1, #2 \rangle}\)
\( \newcommand{\Span}{\mathrm{span}}\) \( \newcommand{\AA}{\unicode[.8,0]{x212B}}\)
\( \newcommand{\vectorA}[1]{\vec{#1}} % arrow\)
\( \newcommand{\vectorAt}[1]{\vec{\text{#1}}} % arrow\)
\( \newcommand{\vectorB}[1]{\overset { \scriptstyle \rightharpoonup} {\mathbf{#1}} } \)
\( \newcommand{\vectorC}[1]{\textbf{#1}} \)
\( \newcommand{\vectorD}[1]{\overrightarrow{#1}} \)
\( \newcommand{\vectorDt}[1]{\overrightarrow{\text{#1}}} \)
\( \newcommand{\vectE}[1]{\overset{-\!-\!\rightharpoonup}{\vphantom{a}\smash{\mathbf {#1}}}} \)
\( \newcommand{\vecs}[1]{\overset { \scriptstyle \rightharpoonup} {\mathbf{#1}} } \)
\( \newcommand{\vecd}[1]{\overset{-\!-\!\rightharpoonup}{\vphantom{a}\smash {#1}}} \)
\(\newcommand{\avec}{\mathbf a}\) \(\newcommand{\bvec}{\mathbf b}\) \(\newcommand{\cvec}{\mathbf c}\) \(\newcommand{\dvec}{\mathbf d}\) \(\newcommand{\dtil}{\widetilde{\mathbf d}}\) \(\newcommand{\evec}{\mathbf e}\) \(\newcommand{\fvec}{\mathbf f}\) \(\newcommand{\nvec}{\mathbf n}\) \(\newcommand{\pvec}{\mathbf p}\) \(\newcommand{\qvec}{\mathbf q}\) \(\newcommand{\svec}{\mathbf s}\) \(\newcommand{\tvec}{\mathbf t}\) \(\newcommand{\uvec}{\mathbf u}\) \(\newcommand{\vvec}{\mathbf v}\) \(\newcommand{\wvec}{\mathbf w}\) \(\newcommand{\xvec}{\mathbf x}\) \(\newcommand{\yvec}{\mathbf y}\) \(\newcommand{\zvec}{\mathbf z}\) \(\newcommand{\rvec}{\mathbf r}\) \(\newcommand{\mvec}{\mathbf m}\) \(\newcommand{\zerovec}{\mathbf 0}\) \(\newcommand{\onevec}{\mathbf 1}\) \(\newcommand{\real}{\mathbb R}\) \(\newcommand{\twovec}[2]{\left[\begin{array}{r}#1 \\ #2 \end{array}\right]}\) \(\newcommand{\ctwovec}[2]{\left[\begin{array}{c}#1 \\ #2 \end{array}\right]}\) \(\newcommand{\threevec}[3]{\left[\begin{array}{r}#1 \\ #2 \\ #3 \end{array}\right]}\) \(\newcommand{\cthreevec}[3]{\left[\begin{array}{c}#1 \\ #2 \\ #3 \end{array}\right]}\) \(\newcommand{\fourvec}[4]{\left[\begin{array}{r}#1 \\ #2 \\ #3 \\ #4 \end{array}\right]}\) \(\newcommand{\cfourvec}[4]{\left[\begin{array}{c}#1 \\ #2 \\ #3 \\ #4 \end{array}\right]}\) \(\newcommand{\fivevec}[5]{\left[\begin{array}{r}#1 \\ #2 \\ #3 \\ #4 \\ #5 \\ \end{array}\right]}\) \(\newcommand{\cfivevec}[5]{\left[\begin{array}{c}#1 \\ #2 \\ #3 \\ #4 \\ #5 \\ \end{array}\right]}\) \(\newcommand{\mattwo}[4]{\left[\begin{array}{rr}#1 \amp #2 \\ #3 \amp #4 \\ \end{array}\right]}\) \(\newcommand{\laspan}[1]{\text{Span}\{#1\}}\) \(\newcommand{\bcal}{\cal B}\) \(\newcommand{\ccal}{\cal C}\) \(\newcommand{\scal}{\cal S}\) \(\newcommand{\wcal}{\cal W}\) \(\newcommand{\ecal}{\cal E}\) \(\newcommand{\coords}[2]{\left\{#1\right\}_{#2}}\) \(\newcommand{\gray}[1]{\color{gray}{#1}}\) \(\newcommand{\lgray}[1]{\color{lightgray}{#1}}\) \(\newcommand{\rank}{\operatorname{rank}}\) \(\newcommand{\row}{\text{Row}}\) \(\newcommand{\col}{\text{Col}}\) \(\renewcommand{\row}{\text{Row}}\) \(\newcommand{\nul}{\text{Nul}}\) \(\newcommand{\var}{\text{Var}}\) \(\newcommand{\corr}{\text{corr}}\) \(\newcommand{\len}[1]{\left|#1\right|}\) \(\newcommand{\bbar}{\overline{\bvec}}\) \(\newcommand{\bhat}{\widehat{\bvec}}\) \(\newcommand{\bperp}{\bvec^\perp}\) \(\newcommand{\xhat}{\widehat{\xvec}}\) \(\newcommand{\vhat}{\widehat{\vvec}}\) \(\newcommand{\uhat}{\widehat{\uvec}}\) \(\newcommand{\what}{\widehat{\wvec}}\) \(\newcommand{\Sighat}{\widehat{\Sigma}}\) \(\newcommand{\lt}{<}\) \(\newcommand{\gt}{>}\) \(\newcommand{\amp}{&}\) \(\definecolor{fillinmathshade}{gray}{0.9}\)-
Define photosynthesis and photoautotrophs
-
Describe properties of light important for photosynthesis
-
Outline the steps in both the light-dependent and light-independent reactions in photosynthesis
-
Explain photorespiration and the three major photosynthetic pathways used by plants
Photoautotrophs
Plants, algae, and certain bacteria (cyanobacteria and green and purple sulfur bacteria) are among the organisms capable of performing photosynthesis (Figure \(\PageIndex{1}\)). Because they use light to manufacture their own food, they are called photoautotrophs (literally, “self-feeders using light”, Greek autos = self and trophe = nutrition). Other organisms, such as animals, fungi, and most other bacteria, are termed heterotrophs (“other feeders”), because they must rely on the sugars produced by photosynthetic organisms for their energy needs. A third very interesting group of bacteria synthesize sugars, not by using sunlight’s energy, but by extracting energy from inorganic chemical compounds; hence, they are referred to as chemoautotrophs.
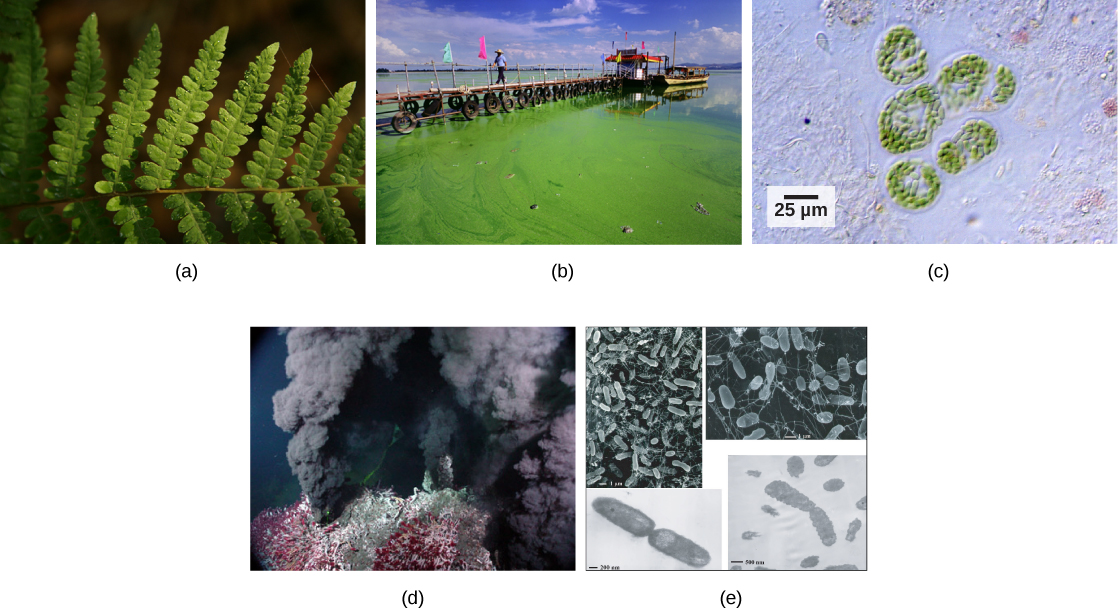
Figure \(\PageIndex{1}\): Photoautotrophs including (a) plants, (b) algae, and (c) cyanobacteria synthesize their organic compounds via photosynthesis using sunlight as an energy source. Cyanobacteria and planktonic algae can grow over enormous areas in water, at times completely covering the surface. In a (d) deep sea vent, chemoautotrophs, such as these (e) thermophilic bacteria, capture energy from inorganic compounds to produce organic compounds. The ecosystem surrounding the vents has a diverse array of animals, such as tubeworms, crustaceans, and octopi that derive energy from the bacteria. (credit a: modification of work by Steve Hillebrand, U.S. Fish and Wildlife Service; credit b: modification of work by "eutrophication&hypoxia"/Flickr; credit c: modification of work by NASA; credit d: University of Washington, NOAA; credit e: modification of work by Mark Amend, West Coast and Polar Regions Undersea Research Center, UAF, NOAA).
Photosynthesis is the process on which photoautotrophs rely to capture the energy in solar radiation (the “photo-” part) as high-energy electrons and use it to produce the carbon-carbon bonds of carbohydrate molecules (the “-synthesis” part). The carbon used to make these molecules comes from the carbon dioxide (CO2) in the atmosphere. Those carbohydrates are the energy source that heterotrophs use to power the synthesis of ATP via cellular respiration. Because photosynthesis removes carbon from the atmosphere and incorporates it into organic molecules which eventually become the plant’s leaves, stems, roots, and fruits, photosynthesis is sometimes said to fix carbon. Fix, in this sense, means to secure or sequester rather than to repair.
Properties of Light
Recall that light travels in waves and that light is made up of particles are called photons. The length of the wave is measured from one peak to the next and is called the wavelength, which differs for different colors of light (Figure \(\PageIndex{2}\). Within the visible wavelengths of light, the longest wavelengths are red light; outside the visible range of wavelengths, even longer wavelengths include infrared radiation, microwaves, and radio waves. Shorter visible wavelengths include blue and purple light, and beyond the visible range even shorter wavelengths include UV light, X-rays, and Gamma rays.
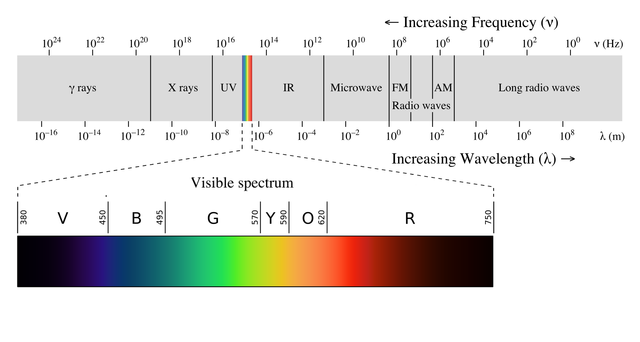
Figure \(\PageIndex{2}\): Visible spectrum. "EM spectrum revised" by Philip Ronan is licensed under CC BY-SA 3.0.
Steps of Photosynthesis
The photons in light provide the energy that drives photosynthesis. The chemical formula is the same for the two types of simple sugars produced by photosynthesis: glucose and fructose: C6H12O6. The equation that summarizes photosynthesis is:
water + carbon dioxide -> oxygen, water, and simple sugars
12H20 + 6CO2 -> 6O2 + 6H2O + C6H12O6
This balanced equation tells us that 12 molecules of water plus 6 molecules of carbon dioxide, in the presence of chlorophyll, accessory pigments, and light, produces 6 molecules of oxygen gas, returns 6 molecules of water back to the cell, and produces one molecule of a simple sugar like glucose or fructose.
Photosynthesis takes place in two sequential stages: the light-dependent reactions and the light independent-reactions (Calvin cycle). In the light-dependent reactions, energy from sunlight is absorbed by chlorophyll and that energy is converted into stored chemical energy. Light-dependent reactions require water and produce oxygen and energy in the form of ATP and NADPH. In the light-independent reactions, the chemical energy harvested during the light-dependent reactions drive the assembly of sugar molecules from carbon dioxide. Therefore, although the light-independent reactions do not use light as a reactant, they require the products of the light-dependent reactions to function. In addition, several enzymes of the light-independent reactions are activated by light. ATP and NAPDH move energy from light-dependent reactions to light-independent reactions. In all autotrophic eukaryotes, photosynthesis takes place inside the chloroplast. Figure \(\PageIndex{3}\) illustrates the components inside the chloroplast (a membrane bound organelle in plant cells) where the light-dependent and light-independent reactions take place.
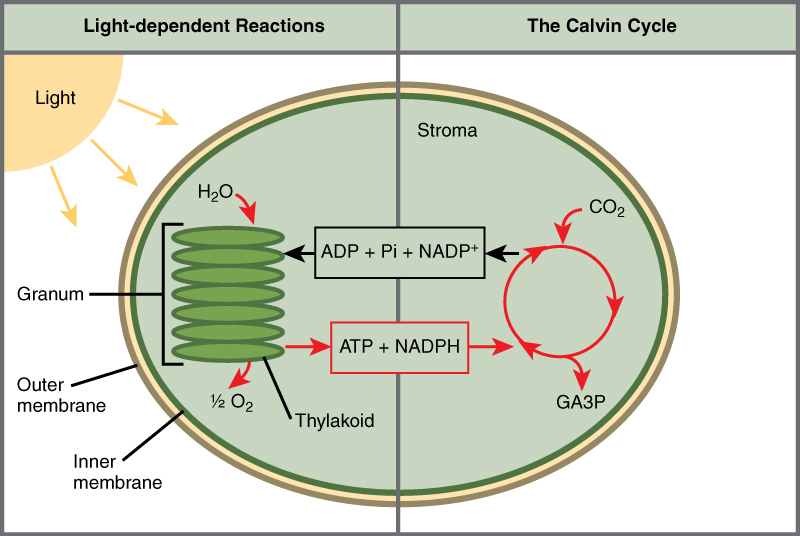
Figure \(\PageIndex{3}\): Photosynthesis takes place in two stages: light-dependent reactions and the light-independent reactions (Calvin cycle or Calvin-Benson cycle). Light-dependent reactions, which take place in the thylakoid membrane, use light energy to make ATP and NADPH. In the process, water is used and oxygen is produced. Energy from ATP and NADPH are used to power the Calvin cycle, which produces GA3P from carbon dioxide. ATP is broken down to ADP and Pi, and NADPH is oxidized to NADP+. The cycle is completed when the light reactions convert these molecules back into ATP and NADPH.
Light-Dependent Reactions
There are two types of chlorophyll, a green pigment that captures light for photosynthesis, Chlorophyll a and Chlorophyll b.
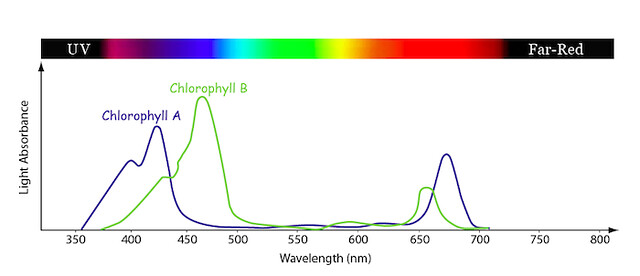
Figure \(\PageIndex{4}\): Chlorophyll A & B Absorption Spectrum. Image by byr7 is licensed under CC BY 2.0
The graph above shows % absorbance of different wavelengths by these two chlorophylls (Figure \(\PageIndex{4}\)). The Y axis (the vertical one) shows the percentage of the light that is absorbed (rather than reflected). High levels of absorption mean that the chlorophyll molecule uses that wavelength of light for energy. Low absorption means that the molecule does not use that wavelength, and is thus reflected away. The X axis indicates the wavelength of light in nanometers (nm) and the bar at the top represents the color of the light at the wavelength shown. The blue line is a typical absorption curve for chlorophyll a, while the green line shows chlorophyll b. Both chlorophyll a and b absorb blue and red light wavelengths and reflect green. Chlorophyll a has a peak in the violet and red regions and chlorophyll b in the blue and orange regions. Notice how their absorbance is very low in the green region. That’s why we think of chlorophyll as green, and why we perceive leaves, which have chlorophyll as the predominant pigment, as green.
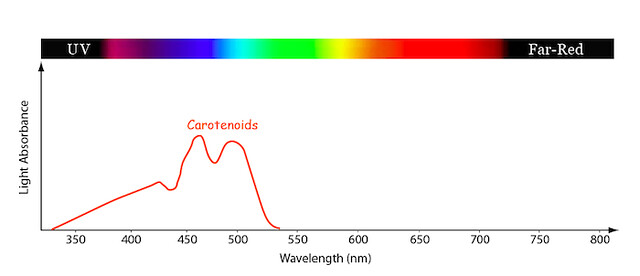
Figure \(\PageIndex{5}\): Carotenoids absorption spectrum. Image by byr7 is licensed under CC BY 2.0.
The graph above shows the absorbance of carotenoid pigments, which are an accessory pigment in photosynthesis and present throughout the growing season (Figure \(\PageIndex{5}\)). Carotenoids absorb light in the green range, but reflect in yellow and red. We don’t see these pigments during the growing season because they are much lower in concentration than the chlorophylls, so the green reflected light overwhelms the orange, and we see green. But when the chlorophyll fades in the fall the orange can be seen in beautiful fall leaf colors.
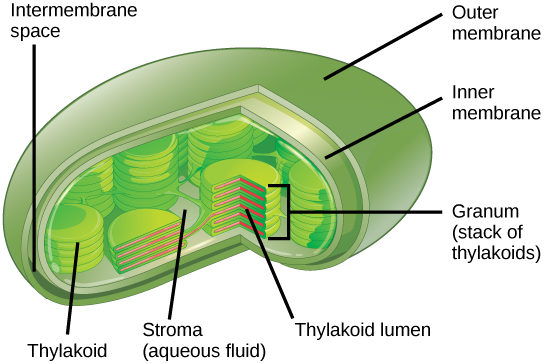
Figure \(\PageIndex{6}\): Photosynthesis takes place in chloroplasts, which have an outer membrane and an inner membrane. The space between the outer and inner membranes is called the intermembrane space. Stacks of thylakoids called grana form a third membrane layer. The thylakoids form stacks called grana. The liquid inside the inner membrane is called the stroma, and the space inside the thylakoid is called the thylakoid lumen.
The chloroplast has a double membrane envelope (composed of an outer membrane and an inner membrane) with an interior called the stroma (Figure \(\PageIndex{6}\)). Within the stroma are coin-like thylakoids and stacks of thylakoids are called grana. The thylakoids are also surrounded by a membrane, called the thylakoid membrane. The thylakoid membrane encloses an internal space called the thylakoid lumen. Embedded in the thylakoid membrane is chlorophyll, as well as the accessory pigments, and numerous proteins that make up the electron transport chain (Figure \(\PageIndex{7}\). In the light-dependent reactions protein complexes and pigment molecules work together to produce NADPH and ATP.
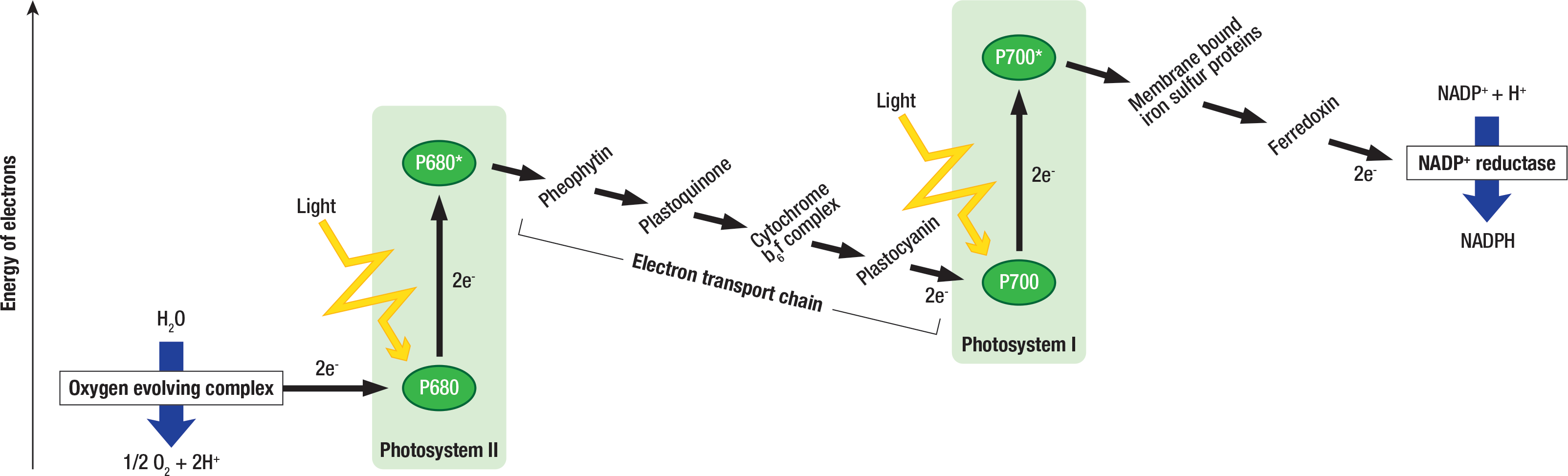
Figure \(\PageIndex{7}\): A diagram of the electron transport chain. Image by Jen Valenzuela (CC-BY).
When light hits a pigment molecule in the thylakoid membrane, the energy from the light photon promotes (pushes up) an electron in one of the pigment’s atoms to a higher orbital as seen in the cartoon and energy is gained (Figure \(\PageIndex{7}\). The electron can’t stay in that higher orbital indefinitely, and when it drops back to its home orbital it releases the energy it absorbed from light, denoted as energy loss. This released energy can be passed to another pigment molecule. This resonance energy travels down the antenna complex to the reaction center, where the captured energy pulls electrons out of water molecules, and water is split into oxygen gas, hydrogen ions, and electrons. This process of one pigment capturing the photon’s energy and passing that energy onto adjacent pigment molecules is the crucial step in energy transformation that takes place in photosynthesis. This is the step that takes light energy and converts it into chemical energy — one of the only known biological processes that allows this type of energy transformation.
Recall that the overall equation for photosynthesis is:
water + carbon dioxide -> oxygen, water, and simple sugars
12H20 + 6CO2 -> 6O2 + 6H2O + C6H12O6
This equation is made up of two parts called half-reactions. The half-reaction for the Light Reaction is as follows:
12H2O -> 6O2 + 24e– + 24H+
Light-Independent reactions
The Light-Independent Reactions is the second part of photosynthesis. It takes place in the stroma of the chloroplast. Unlike the Light-Dependent Reaction, it does not require light. In the Light-Independent Reactions, two compounds, NADPH and ATP, carry the energy from light that was originally transformed into hydrogen ions and electrons through the splitting of water. The NADPH and ATP, along with carbon dioxide from the atmosphere, enter a process called the Calvin Cycle, where the energy is used to fix carbon into a molecule abbreviated G3P as shown in Figure \(\PageIndex{8}\). This process requires the help of an important protein abbreviated RuBisCO (Ribulose-1,5-bisphosphate carboxylase/oxygenase) that catalyzes the step in the process where the carbon from atmospheric CO2 is incorporated into an organic molecule. RuBisCO is the most abundant protein in leaves and, given the number of leaves in the world, likely the most abundant protein on the planet.
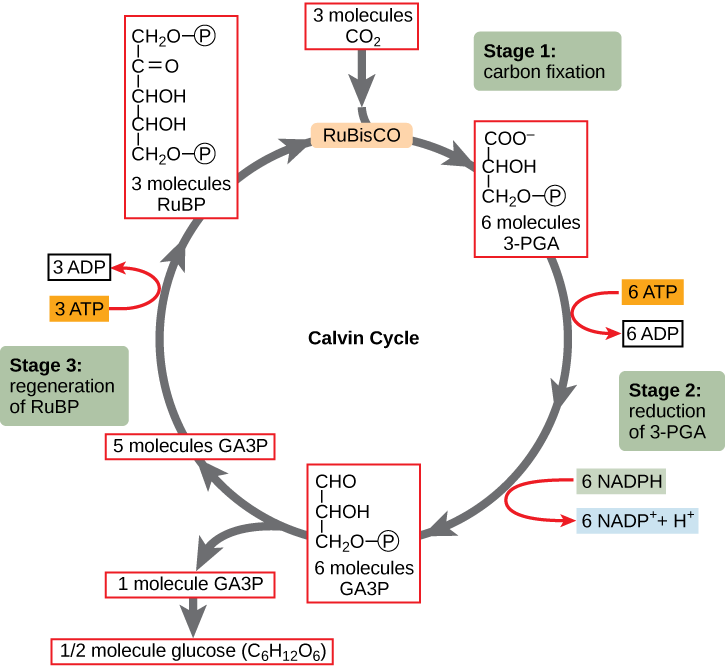
Figure \(\PageIndex{8}\): The light-independent reactions (Calvin cycle) has three stages. In stage 1, the enzyme RuBisCO adds carbon dioxide to RuBP, which immediately splits, producing two three-carbon 3-PGA molecules. In stage 2, two NADPH and two ATP are used to reduce 3-PGA to GA3P. In stage 3, RuBP, the molecule that starts the cycle, is regenerated so that the cycle can continue. One ATP is used in the process. Only one carbon dioxide molecule is incorporated at a time, so the cycle must be completed three times to produce a single three-carbon GA3P molecule, and six times to produce a six-carbon glucose molecule.
RuBisCO catalyzes a reaction between CO2 and RuBP (ribulose-1,5-bisphosphate). For each CO2 molecule that reacts with one RuBP, two molecules of another compound, 3-phosphoglycerate (3-PGA), form. 3-PGA has three carbon atoms and one phosphate. Each turn of the cycle involves only one RuBP and one carbon dioxide and forms two molecules of 3-PGA. The number of carbon atoms remains the same, as the atoms move to form new bonds during the reactions (3 atoms from 3 CO2 + 15 atoms from 3 RuBP = 18 atoms in 3 atoms of 3-PGA). This process is called carbon fixation, because CO2 is “fixed” from an inorganic form into organic molecules.
Six molecules of both ATP and NADPH are used to convert the six molecules of 3-PGA into six molecules of a chemical called glyceraldehyde 3-phosphate (G3P). The G3P produced by the carbon fixation process is called a triose phosphate, meaning it is a 3-carbon sugar (triose) with phosphorus and oxygen atoms (phosphate) attached. Triose phosphate moves out of the chloroplast into the mesophyll cell’s cytoplasm, where two of these three-carbon molecules are combined to produce the 6-carbon molecules glucose and fructose. The glucose and fructose molecules then combine to form sucrose, a 12-carbon organic molecule. Sucrose is important because it is the sugar that is transported by the phloem throughout the plant to provide energy and building blocks for other organic molecules like starch and cellulose.
Interestingly, at this point, only one of the G3P molecules leaves the light-independent reactions and is sent to the cytoplasm to contribute to the formation of other compounds needed by the plant. Because the G3P exported from the chloroplast has three carbon atoms, it takes three “turns” of the cycle to fix enough net carbon to export one G3P. But each turn makes two G3P, thus three turns make six G3P. One of these six is exported while the remaining five G3P molecules remain in the cycle and are used to regenerate RuBP, which enables the system to prepare for more CO2 to be fixed. Three more molecules of ATP are used in these regeneration reactions.
Recall that the overall equation for photosynthesis is:
water + carbon dioxide -> oxygen, water, and simple sugars
12H20 + 6CO2 -> 6O2 + 6H2O + C6H12O6
The half-reaction for the Light-Independent Reactions is:
24H+ + 24e– + 6CO2 -> C6H12O6 + 6H2O
Photorespiration and other Photosynthetic Pathways
Different plant species have adaptations that allow them to do different variations of the light-independent reactions. These are called photosynthetic pathways. Plants are classified as C3, C4, or CAM depending on their use of these pathways, but note that some plants can switch photosynthetic pathways depending on environmental conditions. The process for light-independent reactions described in the previous section was the C3 pathway: the compound formed during fixation (3-PGA) has three carbon atoms. Before discussing the details of the C4 pathway, it is important to understand the circumstances that led to these adaptations.
As its name suggests, ribulose-1,5-bisphosphate carboxylase/oxygenase (RuBisCO) catalyzes two different reactions (Figure \(\PageIndex{9}\)).. The first is adding CO2 to ribulose-1,5- bisphosphate (RuBP) — the carboxylase activity. The second is adding O2 to RuBP — the oxygenase activity. The oxygenase activity of RuBisCO forms the three-carbon molecule 3-phosphoglycerate (3-PGA), just as in the light-independent reactions, and the two-carbon molecule glycolate. The glycolate enters peroxisomes, where it uses O2 to form intermediates that enter mitochondria where they are broken down to CO2. So this process uses O2 and ATP and liberates CO2 as aerobic cellular respiration does, which is why it is called photorespiration. It undoes the work of photosynthesis, which is to build sugars, and wastes ATP which could be more useful to the plant in other ways.
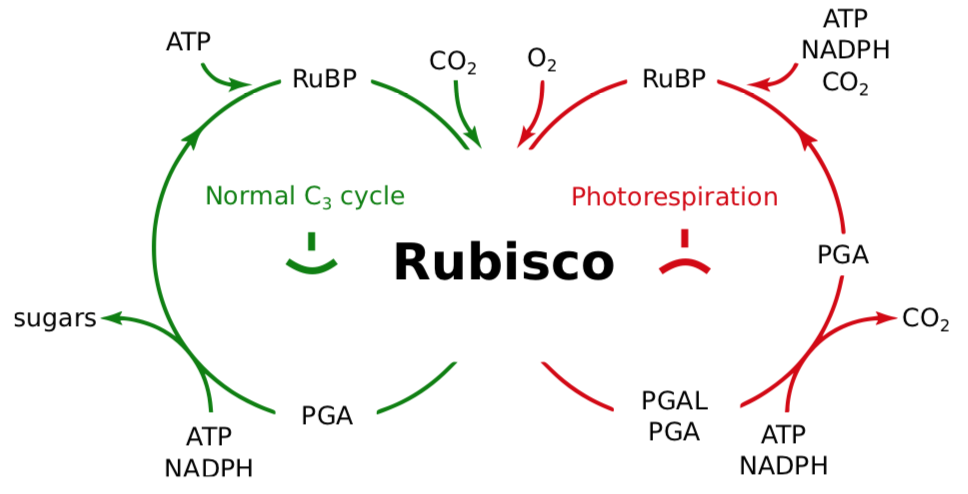
Figure \(\PageIndex{9}\): Rubisco is a two-faced enzyme.
Which action of RuBisCO predominates depends on the relative concentrations of O2 and CO2 with high CO2, low O2 favoring the carboxylase action and high O2, low CO2 favoring the oxygenase action. The light reactions of photosynthesis liberate oxygen, and more oxygen dissolves in the cytosol of the cell at higher temperatures. Therefore, high light intensities and high temperatures (above ~ 30°C) favor the second reaction and result in photorespiration.
If concentration of CO\(_2\) is high enough, assimilation will overcome photorespiration. Consequently, to minimize the amount of photorespiration plants employ Le Chatelier’s principle (“Equilibrium Law”) and increase concentration of carbon dioxide near RuBisCO. One solution to increased concentrations of carbon dioxide in leaf tissues is for plants to open their stomata to release O2 and obtain CO2. However, if conditions are hot or dry, this will result in too much water loss (transpiration). For this reason, C3 plants do best in cool, moist areas. Rice and potatoes are examples of C3 plants.
Plants can also increase the concentration of carbon dioxide near RuBisCO by temporarily bonding carbon dioxide with phosphoenolpyruvic acid (PEP, C\(_3\)) using carboxylase enzyme; this results in C\(_4\) molecules, like malate or malic acid, with four carbons in the skeleton. When plant needs it, that C\(_4\) splits into pyruvate (C\(_3\)) plus carbon dioxide, and the release of that carbon dioxide will increase its concentration. On the final step, pyruvate plus ATP react to restore PEP; recovery of PEP does cost ATP. This entire process is called the “C\(_\mathbf{4}\) pathway” (Figure \(\PageIndex{10}\)). These plants are called C4 plants.
C4 plants have structural changes in their leaf anatomy so that synthesizing the four-carbon sugar (the C4 pathway) and resuming the light-independent reactions (C3 pathways) are separated in different parts of the leaf with RuBisCO sequestered where the CO2 level is high and the O2 level low. After entering through stomata, CO2 diffuses into a mesophyll cell (Figure \(\PageIndex{10}\)) where it undergoes the C4 pathway. Being close to the leaf surface, these cells are exposed to high levels of O2, but they have no RuBisCO so cannot start photorespiration (nor the light-independent reactions). Then the four carbon molecules are transported in to bundle sheath cells (Figure \(\PageIndex{11}\)) deep in the leaf, so atmospheric oxygen cannot diffuse easily to them. In the bundle sheath cells, the four-carbon compound is broken down into carbon dioxide, which enters the light-independent reactions (C3 pathway) to form sugars, and pyruvic acid, which is transported back to a mesophyll cell where it is converted back into PEP.
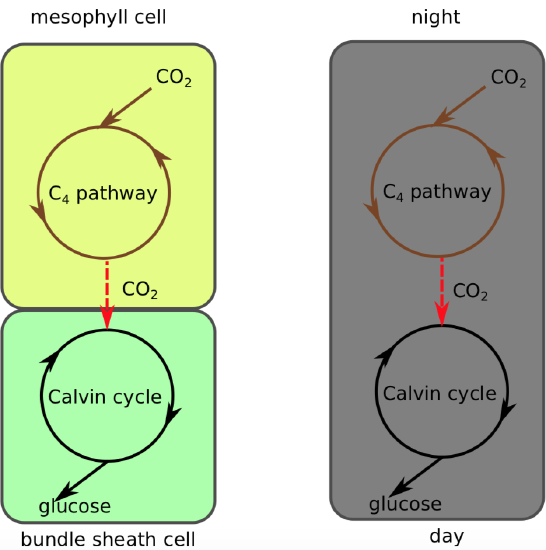
Figure \(\PageIndex{10}\): C\(_4\) plants (left) conduct the C4 pathway in the mesophyll cells and the Calvin cycle (C3 pathway) in the bundle sheath cells, meaning they spatially separate the two . CAM plants (right) conduct the C4 pathway at night and the Calvin cycle (C3 pathway) during the day, resulting an a temporal separation of the two.
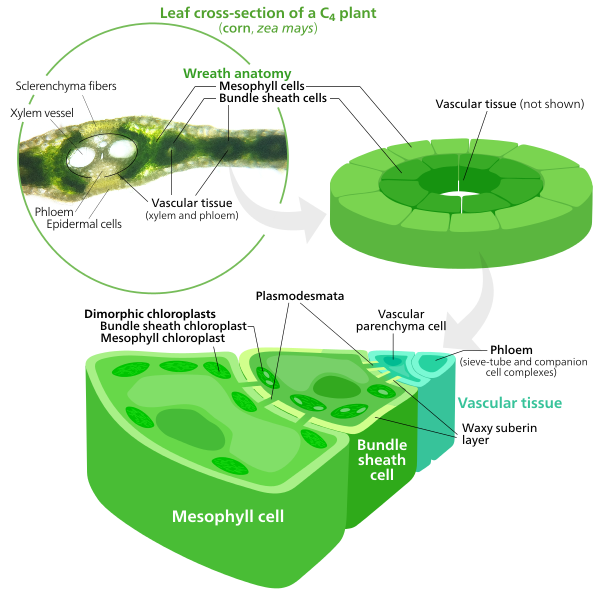
Figure \(\PageIndex{11}\): Cross section and diagram of a C4 plant, showing mesophyll cells surrounding bundle sheath cells in concentric circles. The bundle sheath cells are larger and have more chloroplasts than in other plants. This arrangement is called Kranz anatomy (wreath anatomy). Vascular bundles contain xylem (vessel elements and tracheids) and phloem (sieve-tube and companion cell complexes). A vascular parenchyma is also shown in the vascular bundle. Waxy suberin borders the bundle sheath cells. The chloroplasts in the mesophyll cells are distinct from those in the bundle sheath cells, and the chloroplasts are thus called dimorphic. Sclerenchyma fibers are just above and below the vascular bundle, and epidermal cells surround the entire leaf. Image by Kelvinsong (CC-BY-SA).
These C4 plants are well adapted to (and likely to be found in) habitats with high daytime temperatures and intense sunlight. Because they use the C4 pathway to prevent photorespiration, they do not have to open their stomata to the same extent as C3 plants and can thus conserve water. Some examples crabgrass, corn (maize), sugarcane, and sorghum. Although comprising only ~3% of the angiosperms by species, C4 plants are responsible for ~25% of all the photosynthesis on land.
CAM stands for crassulacean acid metabolism because it was first studied in members of the plant family Crassulaceae. CAM plants also do the C4 pathway. However, instead of segregating the C4 and C3 pathways in different parts of the leaf, CAM plants separate them in time instead (Figure \(\PageIndex{10}\), Table \(\PageIndex{1}\)). As a result, CAM plants do not need to open their stomata in the daytime to reduce photorespiration because they have already formed a four-carbon molecule at night that can be broken down to release carbon dioxide during the day.
Night | Morning |
---|---|
|
|
CAM plants thus thrive in conditions of high daytime temperatures, intense sunlight, and low soil moisture. Some examples of CAM plants include cacti (Figure \(\PageIndex{12}\)), pineapples, all epiphytic bromeliads, sedums, and the "ice plant" that invade the California coast line.
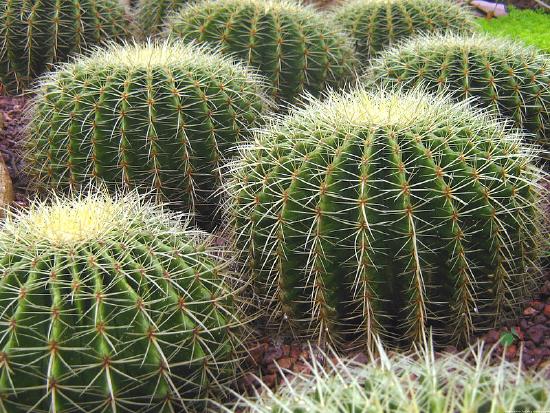
Figure \(\PageIndex{12}\): Cultivated cacti in the Singapore Botanic Gardens. Image by Calvin Teo (CC-BY-SA).
This chapter defines photosynthesis, autotrophs, and heterotrophs and describes key properties of light for the reactions of photosysnthesis. The chapter outlines the important steps in the photosynthesis reactions and differentiates between the light-dependent reactions and the light-independent reactions in photosynthesis. Finally, the chapter explains the concepts of photorespiration and compares the three major photosynthetic pathways used by plants (C3, C4, and CAM).
Contributors and Attributions
Modified by Kyle Whittinghill (University of Pittsburgh) from the following sources
- 11.2: Light and Photosynthesis by Tom Michaels, Matt Clark, Emily Hoover, Laura Irish, Alan Smith, and Emily Tepe. Original source: The Science of Plants: Understanding Plants and How They Grow by Tom Michaels; Matt Clark; Emily Hoover; Laura Irish; Alan Smith; and Emily Tepe
- Some of the explanations and pictures used in this section came from Introduction to photosynthesis and other pages at that McDaniel College site.
- 8.1 Overview of Photosynthesis, 8.2 The Light-Dependent Reactions of Photosynthesis, and 8.3 Using Light Energy to Make Organic Molecules from Biology 2e by OpenStax (licensed CC-BY). Access for free at openstax.org.
- 3.1 Discovery of Photosynthesis and 3.3 Enzymatic Stage from Introduction to Botany by Alexey Shipunov
- 13.3: Photosynthesis Overview and Equation, 13.5: The Light-dependent Reactions, 13.6: Light-independent Reactions, and 13.7: Photorespiration and Photosynthetic Pathways by Melissa Ha, Maria Morrow, & Kammy Algiers is licensed CC BY-NC 4.0.
- 16.2E Photorespiration and C4 Plants from Biology by John W. Kimball (licensed CC-BY)