7.2: Vision
- Page ID
- 103288
\( \newcommand{\vecs}[1]{\overset { \scriptstyle \rightharpoonup} {\mathbf{#1}} } \)
\( \newcommand{\vecd}[1]{\overset{-\!-\!\rightharpoonup}{\vphantom{a}\smash {#1}}} \)
\( \newcommand{\id}{\mathrm{id}}\) \( \newcommand{\Span}{\mathrm{span}}\)
( \newcommand{\kernel}{\mathrm{null}\,}\) \( \newcommand{\range}{\mathrm{range}\,}\)
\( \newcommand{\RealPart}{\mathrm{Re}}\) \( \newcommand{\ImaginaryPart}{\mathrm{Im}}\)
\( \newcommand{\Argument}{\mathrm{Arg}}\) \( \newcommand{\norm}[1]{\| #1 \|}\)
\( \newcommand{\inner}[2]{\langle #1, #2 \rangle}\)
\( \newcommand{\Span}{\mathrm{span}}\)
\( \newcommand{\id}{\mathrm{id}}\)
\( \newcommand{\Span}{\mathrm{span}}\)
\( \newcommand{\kernel}{\mathrm{null}\,}\)
\( \newcommand{\range}{\mathrm{range}\,}\)
\( \newcommand{\RealPart}{\mathrm{Re}}\)
\( \newcommand{\ImaginaryPart}{\mathrm{Im}}\)
\( \newcommand{\Argument}{\mathrm{Arg}}\)
\( \newcommand{\norm}[1]{\| #1 \|}\)
\( \newcommand{\inner}[2]{\langle #1, #2 \rangle}\)
\( \newcommand{\Span}{\mathrm{span}}\) \( \newcommand{\AA}{\unicode[.8,0]{x212B}}\)
\( \newcommand{\vectorA}[1]{\vec{#1}} % arrow\)
\( \newcommand{\vectorAt}[1]{\vec{\text{#1}}} % arrow\)
\( \newcommand{\vectorB}[1]{\overset { \scriptstyle \rightharpoonup} {\mathbf{#1}} } \)
\( \newcommand{\vectorC}[1]{\textbf{#1}} \)
\( \newcommand{\vectorD}[1]{\overrightarrow{#1}} \)
\( \newcommand{\vectorDt}[1]{\overrightarrow{\text{#1}}} \)
\( \newcommand{\vectE}[1]{\overset{-\!-\!\rightharpoonup}{\vphantom{a}\smash{\mathbf {#1}}}} \)
\( \newcommand{\vecs}[1]{\overset { \scriptstyle \rightharpoonup} {\mathbf{#1}} } \)
\( \newcommand{\vecd}[1]{\overset{-\!-\!\rightharpoonup}{\vphantom{a}\smash {#1}}} \)
\(\newcommand{\avec}{\mathbf a}\) \(\newcommand{\bvec}{\mathbf b}\) \(\newcommand{\cvec}{\mathbf c}\) \(\newcommand{\dvec}{\mathbf d}\) \(\newcommand{\dtil}{\widetilde{\mathbf d}}\) \(\newcommand{\evec}{\mathbf e}\) \(\newcommand{\fvec}{\mathbf f}\) \(\newcommand{\nvec}{\mathbf n}\) \(\newcommand{\pvec}{\mathbf p}\) \(\newcommand{\qvec}{\mathbf q}\) \(\newcommand{\svec}{\mathbf s}\) \(\newcommand{\tvec}{\mathbf t}\) \(\newcommand{\uvec}{\mathbf u}\) \(\newcommand{\vvec}{\mathbf v}\) \(\newcommand{\wvec}{\mathbf w}\) \(\newcommand{\xvec}{\mathbf x}\) \(\newcommand{\yvec}{\mathbf y}\) \(\newcommand{\zvec}{\mathbf z}\) \(\newcommand{\rvec}{\mathbf r}\) \(\newcommand{\mvec}{\mathbf m}\) \(\newcommand{\zerovec}{\mathbf 0}\) \(\newcommand{\onevec}{\mathbf 1}\) \(\newcommand{\real}{\mathbb R}\) \(\newcommand{\twovec}[2]{\left[\begin{array}{r}#1 \\ #2 \end{array}\right]}\) \(\newcommand{\ctwovec}[2]{\left[\begin{array}{c}#1 \\ #2 \end{array}\right]}\) \(\newcommand{\threevec}[3]{\left[\begin{array}{r}#1 \\ #2 \\ #3 \end{array}\right]}\) \(\newcommand{\cthreevec}[3]{\left[\begin{array}{c}#1 \\ #2 \\ #3 \end{array}\right]}\) \(\newcommand{\fourvec}[4]{\left[\begin{array}{r}#1 \\ #2 \\ #3 \\ #4 \end{array}\right]}\) \(\newcommand{\cfourvec}[4]{\left[\begin{array}{c}#1 \\ #2 \\ #3 \\ #4 \end{array}\right]}\) \(\newcommand{\fivevec}[5]{\left[\begin{array}{r}#1 \\ #2 \\ #3 \\ #4 \\ #5 \\ \end{array}\right]}\) \(\newcommand{\cfivevec}[5]{\left[\begin{array}{c}#1 \\ #2 \\ #3 \\ #4 \\ #5 \\ \end{array}\right]}\) \(\newcommand{\mattwo}[4]{\left[\begin{array}{rr}#1 \amp #2 \\ #3 \amp #4 \\ \end{array}\right]}\) \(\newcommand{\laspan}[1]{\text{Span}\{#1\}}\) \(\newcommand{\bcal}{\cal B}\) \(\newcommand{\ccal}{\cal C}\) \(\newcommand{\scal}{\cal S}\) \(\newcommand{\wcal}{\cal W}\) \(\newcommand{\ecal}{\cal E}\) \(\newcommand{\coords}[2]{\left\{#1\right\}_{#2}}\) \(\newcommand{\gray}[1]{\color{gray}{#1}}\) \(\newcommand{\lgray}[1]{\color{lightgray}{#1}}\) \(\newcommand{\rank}{\operatorname{rank}}\) \(\newcommand{\row}{\text{Row}}\) \(\newcommand{\col}{\text{Col}}\) \(\renewcommand{\row}{\text{Row}}\) \(\newcommand{\nul}{\text{Nul}}\) \(\newcommand{\var}{\text{Var}}\) \(\newcommand{\corr}{\text{corr}}\) \(\newcommand{\len}[1]{\left|#1\right|}\) \(\newcommand{\bbar}{\overline{\bvec}}\) \(\newcommand{\bhat}{\widehat{\bvec}}\) \(\newcommand{\bperp}{\bvec^\perp}\) \(\newcommand{\xhat}{\widehat{\xvec}}\) \(\newcommand{\vhat}{\widehat{\vvec}}\) \(\newcommand{\uhat}{\widehat{\uvec}}\) \(\newcommand{\what}{\widehat{\wvec}}\) \(\newcommand{\Sighat}{\widehat{\Sigma}}\) \(\newcommand{\lt}{<}\) \(\newcommand{\gt}{>}\) \(\newcommand{\amp}{&}\) \(\definecolor{fillinmathshade}{gray}{0.9}\)Figure \(\PageIndex{1}\) appears at first glance to be just a pattern of colored leaves, but hidden within it is the three-dimensional shape of an ant. Can you see the ant among the leaves? This figure is an example of a stereogram, which is a two-dimensional picture that reveals a three-dimensional object when viewed correctly. If you can’t see the hidden image, it doesn’t mean that there is anything wrong with your eyes. It’s all in how your brain interprets what your eyes are sensing. The eyes are special sensory organs, and vision is one of our special senses.
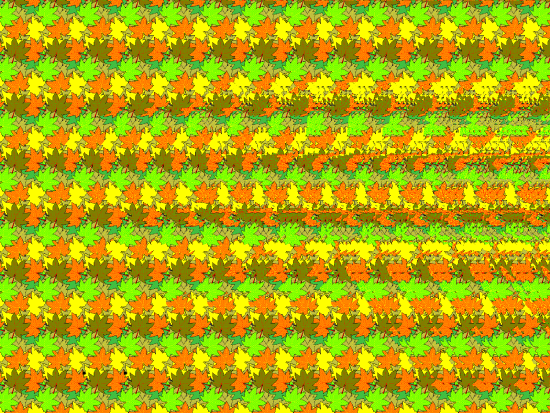
Figure \(\PageIndex{1}\): Stereogram
Vision
Vision is the ability to detect light patterns from the outside environment and interpret them into images. Basically vision, or sight, is the ability to sense light and see. The eye is the special sensory organ that collects and focuses light and forms images. However, the eye is not sufficient for us to see. The brain also plays a necessary role in vision.
Humans are bombarded with sensory information, and the sheer volume of visual information can be problematic. Fortunately, our visual system is able to attend to the most-important stimuli. The importance of vision to humans is further substantiated by the fact that about one-third of the human cerebral cortex is dedicated to analyzing and perceiving visual information.
Anatomy of the Eye
The photoreceptive (light receiving) cells of the eye, where conversion of light to nervous impulses occurs, are located in the retina (shown in Figure \(\PageIndex{2}\): (a)) on the inner surface of the back of the eye. Light passes through several layers of the eye that process it so that it can be interpreted by the retina (Figure \(\PageIndex{2}\): (b)). The cornea (Figures \(\PageIndex{2}\) and \(\PageIndex{3}\)), the front transparent layer of the eye, and the crystalline lens, a transparent convex structure behind the cornea, both refract (bend) light to focus the image on the retina. The iris, which is conspicuous as the colored part of the eye, is a circular muscular ring lying between the lens and cornea that regulates the amount of light entering the eye. In conditions of high light, the iris contracts, reducing the size of the pupil at its center. In conditions of low light, the iris relaxes and the pupil enlarges.
The main function of the lens is to focus light on the retina and fovea. The lens is dynamic, focusing and re-focusing light as the eye rests on near and far objects in the visual field. The lens is operated by muscles that stretch it flat or allow it to thicken, changing the focal length of light coming through it to focus it sharply on the retina.
The eyelids, with lashes at their leading edges, help to protect the eye from abrasions by blocking particles that may land on the surface of the eye. The inner surface of each lid is a thin membrane known as the palpebral conjunctiva. The conjunctiva extends over the white areas of the eye (the sclera), connecting the eyelids to the eyeball. Tears are produced by the lacrimal gland, located beneath the lateral edges of the nose. Tears produced by this gland flow through the lacrimal duct to the medial corner of the eye, where the tears flow over the conjunctiva, washing away foreign particles.
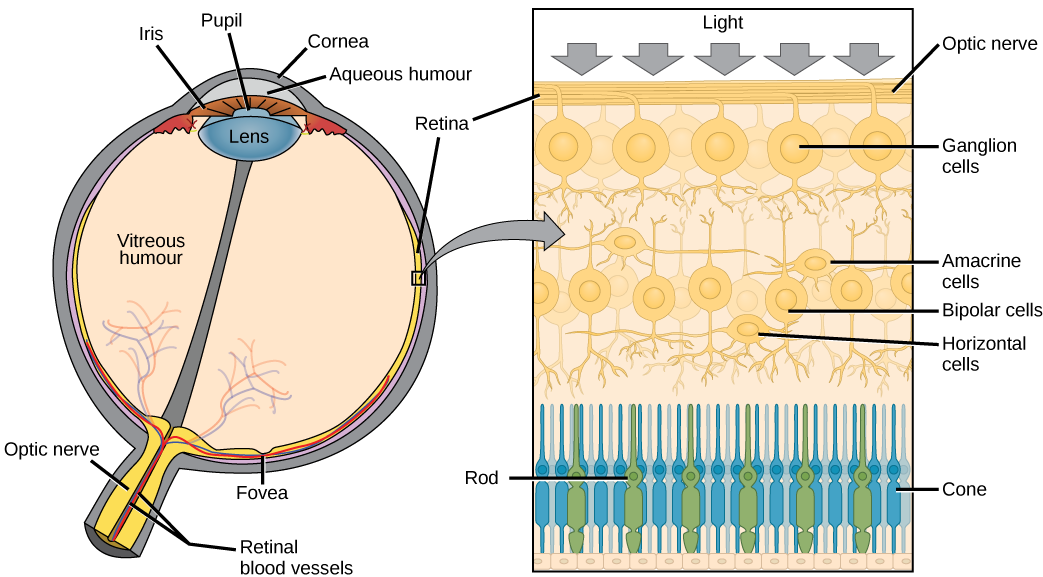
Figure \(\PageIndex{2}\): (a) The human eye is shown in cross section. (b) A blowup shows the layers of the retina.
.
Figure \(\PageIndex{3}\): The Eye in the Orbit The eye is located within the orbit and surrounded by soft tissues that protect and support its function. The orbit is surrounded by cranial bones of the skull.
There are two types of photoreceptor cells in the retina: rods and cones, named for their general appearance as illustrated in Figure \(\PageIndex{4}\): . Rods are strongly photosensitive and are located in the outer edges of the retina; Rods detect dim light and are used primarily for peripheral and nighttime vision. Cones are weakly photosensitive and are located near the center of the retina; Cones respond to bright light, and their primary role is in daytime, color vision.
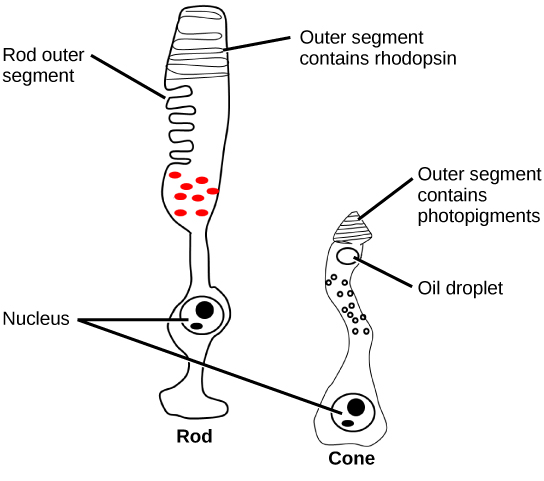
Figure \(\PageIndex{4}\): Rods and cones are photoreceptors in the retina. Rods respond in low light and can detect only shades of gray. Cones respond in intense light and are responsible for color vision. (credit: modification of work by Piotr Sliwa)
The fovea is the region in the center back of the eye that is responsible for acute vision. The fovea has a high density of just cones. When you bring your gaze to an object to examine it intently in bright light, the eyes orient so that the object’s image falls on the fovea. This is the area of the retina that gives us high clarity of vision. However, when looking at a star in the night sky or other object in dim light, the object can be better viewed by the peripheral vision because it is the rods in higher concentrations in the other regions of the retina, rather than the cones at the center, that operate better in low light. In low-light conditions, the rods allow us to see in shades of gray because cones require bright light to be stimulated and don't respond in low light conditions.
LINK TO LEARNING
Review the anatomical structure of the eye, clicking on each part to practice identification.
How the Eye Works
Figure \(\PageIndex{5}\) shows the anatomy of the human eye in cross-section. The eye gathers and focuses light to form an image and then changes the image to nerve impulses that travel to the brain. How the eye performs these functions is summarized in the following steps.
- Light passes first through the cornea, which is a clear outer layer that protects the eye and helps to focus the light by refracting, or bending, it.
- Light next enters the interior of the eye through an opening called the pupil. The size of this opening is controlled by the colored part of the eye, called the iris, which adjusts the size based on the brightness of the light. The iris causes the pupil to narrow in bright light and widen in dim light.
- The light then passes through the lens, which refracts the light even more and focuses it on the retina at the back of the eye as an inverted image.
- The retina contains photoreceptor cells of two types, called rods and cones. Rods, which are found mainly in all areas of the retina other than the very center, are particularly sensitive to low levels of light. Cones, which are found mainly in the center of the retina, are sensitive to light of different colors and allow color vision. The rods and cones convert the light that strikes them to nerve impulses.
- The nerve impulses from the rods and cones travel to the optic nerve via the optic disc, which is a circular area at the back of the eye where the optic nerve connects to the retina.
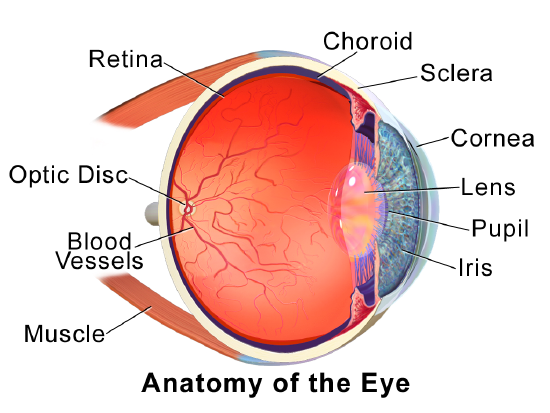
Figure \(\PageIndex{5}\): In this image, you can see the three layers of the eyeball, the sclera (cornea at the front), choroid, and retina. Lens, pupil, Irish, optic disc, option nerve (not labeled), blood vessels, and eye muscles are also visible. Trace the path of light through the eye as you read about in the five steps described in the text
Role of the Brain in Vision
The optic nerves from both eyes meet and cross just below the bottom of the hypothalamus in the brain. The information from both eyes is sent to the visual cortex in the occipital lobe of the cerebrum, which is part of the cerebral cortex. The visual cortex is the largest system in the human brain and is responsible for processing visual images. It interprets messages from both eyes and “tells” us what we are seeing.
Processing Visual Input
Light
Light travels in electromagnetic waves. Figure \(\PageIndex{6}\) of the electromagnetic spectrum shows that visible light for humans is just a small slice of the entire spectrum, which includes radiation that we cannot see as light because it is below the frequency of visible red light or above the frequency of visible violet light. The wavelength of light is expressed in nanometers (nm); one nanometer is one billionth of a meter.
Humans can see light that ranges between approximately 380 nanometers (nm) and 740 nm wavelengths (called visible light). We perceive different wavelengths within this range as different colors. Some other animals, though, can detect wavelengths outside of the human range. For example, bees see near-ultraviolet light in order to locate nectar guides on flowers, and some non-avian reptiles sense infrared light (heat that prey gives off).
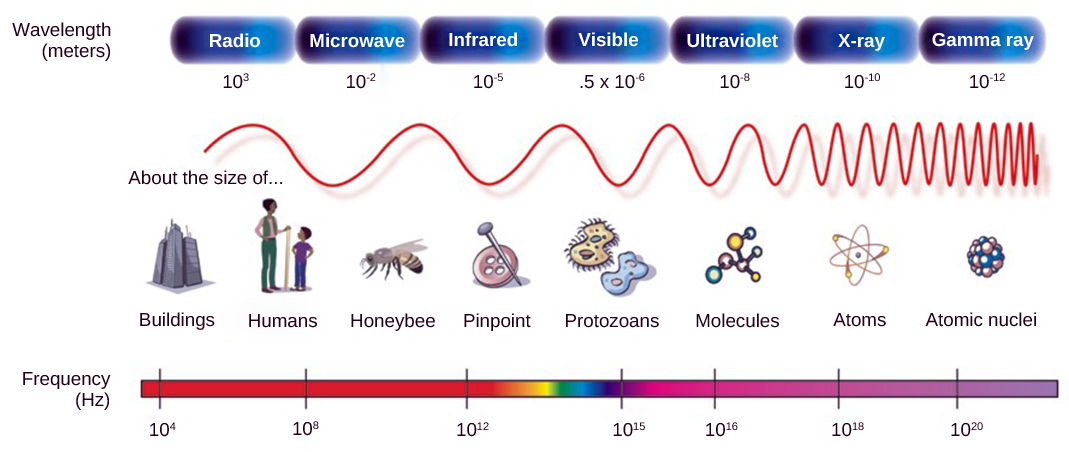
Figure \(\PageIndex{6}\): In the electromagnetic spectrum, visible light lies between 380 nm and 740 nm. (credit: modification of work by NASA)
Color Vision (Trichromatic Coding)
There are three types of cones, each with different photoreceptive proteins (photopsins). By being sensitive to different wavelengths of light, provide us with color vision (Figure \(\PageIndex{7}\)). By comparing the activity of the three different cones, the brain can extract color information from visual stimuli. For example, a bright blue light that has a wavelength of approximately 450 nm would activate the “red” cones minimally, the “green” cones marginally, and the “blue” cones predominantly. The relative activation of the three different cones is calculated by the brain, which perceives the color as blue. However, cones cannot react to low-intensity light, and rods do not sense the color of light. Therefore, our low-light vision is—in essence—in grayscale. In other words, in a dark room, everything appears as a shade of gray. If you think that you can see colors in the dark, it is most likely because your brain knows what color something is and is relying on that memory.
With only one type of cone, color vision would not be possible, and a two-cone (dichromatic) system has limitations. Primates, including humans, use a three-cone (trichromatic) system, resulting in full color vision. The color we perceive is a result of the ratio of activity of our three types of cones. Humans have very sensitive perception of color and can distinguish about 500 levels of brightness, 200 different hues, and 20 steps of saturation, or about 2 million distinct colors.
Figure \(\PageIndex{7}\): Human rod cells and the different types of cone cells each have an optimal wavelength. However, there is considerable overlap in the wavelengths of light detected.
Retinal Processing
Visual signals leave the cones and rods, travel to the bipolar cells, and then to ganglion cells. A large degree of processing of visual information occurs in the retina itself, before visual information is sent to the brain.
Higher Processing
The myelinated axons of ganglion cells make up the optic nerves. Within the nerves, different axons carry different qualities of the visual signal. Some axons constitute the magnocellular (big cell) pathway, which carries information about form, movement, depth, and differences in brightness. Other axons constitute the parvocellular (small cell) pathway, which carries information on color and fine detail. Some visual information projects directly back into the brain, while other information crosses to the opposite side of the brain. This crossing of optical pathways produces the distinctive optic chiasma (Greek, for “crossing”) found at the base of the brain and allows us to coordinate information from both eyes.
Once in the brain, visual information is processed in several places, and its routes reflect the complexity and importance of visual information to humans and other animals.
Vision Problems
Vision problems are very common. Two of the most common are myopia and hyperopia, and they often start in childhood or adolescence. Another common problem, called presbyopia, occurs in most people beginning in middle adulthood. All three problems result in blurred vision due to the failure of the eyes to focus images correctly on the retina.
Myopia
Myopia, or nearsightedness, occurs when the light that comes into the eye does not directly focus on the retina but in front of it, as shown in Figure \(\PageIndex{8}\). This causes the image of distant objects to be out of focus but does not affect the focus of close objects. Myopia may occur because the eyeball is elongated from front to back or because the cornea is too curved. Myopia can be corrected through the use of corrective lenses, either eyeglasses or contact lenses. Myopia can also be corrected by refractive surgery performed with a laser.
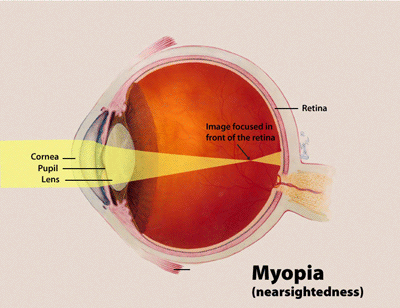
Figure \(\PageIndex{8}\): In myopia, the focal point of images is in front of the retina because the eyeball is elongated.
Hyperopia
Hyperopia, or farsightedness, occurs when the light that comes into the eye does not directly focus on the retina but behind it, as shown in Figure \(\PageIndex{9}\). This causes the image of close objects to be out of focus but does not affect the focus of distant objects. Hyperopia may occur because the eyeball is too short from front to back or because the lens is not curved enough. Hyperopia can be corrected through the use of corrective lenses or laser surgery.
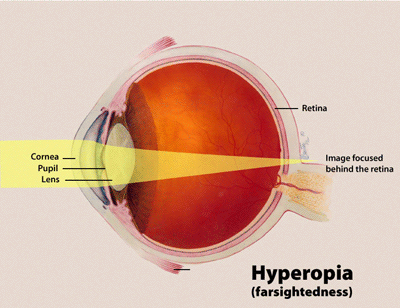
Figure \(\PageIndex{9}\): In hyperopia, the focal point of images is behind the retina because the eyeball is too short.
Presbyopia
Presbyopia is a vision problem associated with aging in which the eye gradually loses its ability to focus on close objects. Presbyopia occurs because the image focuses behind the retina. The precise cause of presbyopia is not known for certain, but evidence suggests that the lens may become less elastic with age, and the muscles that control the lens may lose power as people grow older. The first signs of presbyopia – eyestrain, difficulty seeing in dim light, problems focusing on small objects, and fine print – are usually first noticed between the ages of 40 and 50. Most older people with this problem use corrective lenses to focus on close objects because surgical procedures to correct presbyopia have not been as successful as those for myopia and hyperopia.
The most common cause of blindness in the Western hemisphere is age-related macular degeneration (AMD). About 15 million people in the United States have this type of blindness, and 30 million people are affected worldwide. At present, there is no cure for AMD. The disease occurs with the death of a layer of cells called retinal pigment epithelium, which normally provides nutrients and other support to the macula of the eye. The macula is an oval-shaped pigmented area near the center of the retina that is specialized for high visual acuity and has the retina’s greatest concentration of cones. When the epithelial cells die and the macula is no longer supported or nourished, the macula also starts to die. Patients experience a black spot in the center of their vision, and as the disease progresses, the black spot grows outward. Patients eventually lose the ability to read and even to recognize familiar faces before developing total blindness.
In 2016, a landmark surgery was performed as a trial on a patient with severe AMD. In the first-ever operation of its kind, Dr. Pete Coffey of the University of London implanted a tiny patch of cells behind the retina in each of the patient’s eyes. The cells were retinal pigmented epithelial cells that had been grown in a lab from stem cells, which are undifferentiated cells that have the ability to develop into other cell types. By six months out from the operation, the new cells were still surviving, and the doctor was hopeful that the patient’s vision loss would stop and even be reversed. At that point, several other operations had already been planned to test the new procedure. If these cases are a success, Dr. Coffey predicts that the surgery will become as routine as cataract surgery and prevent millions of patients from losing their vision.
Section Summary
Vision is the only photo responsive sense. Visible light travels in waves and is a very small slice of the electromagnetic radiation spectrum. Light waves differ based on their frequency (wavelength = hue) and amplitude (intensity = brightness).
In the retina there are two types of light receptors (photoreceptors): cones and rods. Cones, which are the source of color vision, exist in three forms—L, M, and S—and they are differentially sensitive to different wavelengths. Cones are located in the retina, along with the dim-light, achromatic receptors (rods). Cones are found in the fovea, the central region of the retina, whereas rods are found everywhere else throughout the retina.
Visual signals travel from the eye over the axons of retinal ganglion cells, which make up the optic nerves. Ganglion cells come in several versions. Some ganglion cell axons carry information on form, movement, depth, and brightness, while other axons carry information on color and fine detail.
Questions
- Explain how the eye collects and focuses light to form an image and converts it to nerve impulses.
- Identify two common vision problems, including both their causes and their effects on vision.
- If a person is blind but their retina is functioning properly, where do you think the damage might be? Explain your answer.
- When you see colors, what receptor cells are activated? Where are these receptors located? What lobe of the brain is primarily used to process visual information?
5. Why do people over 55 often need reading glasses?
- Their cornea no longer focuses correctly.
- Their lens no longer focuses correctly.
- Their eyeball has elongated with age, causing images to focus in front of their retina.
- Their retina has thinned with age, making vision more difficult.
6. Why is it easier to see images at night using peripheral, rather than the central, vision?
- Cones are denser in the periphery of the retina.
- Bipolar cells are denser in the periphery of the retina.
- Rods are denser in the periphery of the retina.
- The optic nerve exits at the periphery of the retina.
Explore More
Some people “see” sounds, “hear” colors, or “taste” words. This rare ability is called synesthesia, and it is thought to be caused by cross-wiring of the senses in the brain. To learn more about this intriguing phenomenon, watch this fascinating TED animation: