7.4: Size and Shape
- Page ID
- 14888
The final type of developmental patterning that evolution can act on is the size and shape of tissues or organs. These are generally considered "morphometric" scaling issues and are classified as "allometric" changes. Morphometrics is the study of how a continuous geometry (like the outer surface of a body) can be warped. Allometry studies this in the context of evolution and development. One of the people who defined the field, D'arcy Wentworth Thompson, came up with morphometric changes that allow one known species of fish to be warped into another known species (Figure 7).
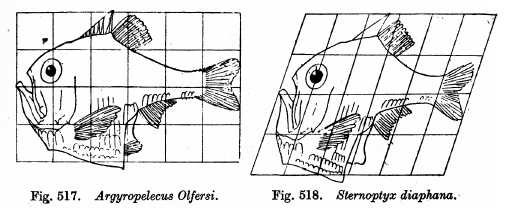
While the extent of the role that allometry plays in evolution isn't totally clear, we know from examples that it does play some role. It's easy to see this if we look around at related species. For example, hominid skulls are very alike and often differ in ways that are easily explained by small variations in developmental growth patterns. Extra or earlier cell proliferation here, less or later cell proliferation there. While we know many genetic markers of cell proliferation (for example cell-cycle regulator Cyclin D1) increase cell-proliferation, there are many different upstream activators of proliferation depending on tissue/cell-type and developmental stage. To begin to understand the role of allometry in evolution, researchers compare tissue growth and the regulators of that growth between fairly closely related species.
Two places where both the development and evolution of size and shape changes are fairly well understood are beak shapes in birds and elytra in beetles. One of the most famous examples of evolution is Darwin's Finches. This group of finches underwent an adaptive radiation on the Galapagos Islands beginning about 2.3 million years ago12. Since then, the finches have adapted to different island niches into 14 different species. Comparing beak morphology between these species uncovered two conserved allometric programs for changing cell proliferation patterns. In early development, beak width is regulated by levels of BMP4 - higher BMP4 means a deeper and wider beak. For example, ground finches have high BMP4 expression in a region that signals to the predominate skeletogenic region of the early beak - the pre-nasal cartilage (pnc)13. Likewise, high levels of calmodulin next to the pnc are associated with longer beaks, like the beaks of cactus finches (Figure 8).

Slightly later in development, an upper beak structure called the pre-maxillary bone (pmx) begins to grow. This will eventually form the parts of the upper beak that structurally and functionally differ between many bird species. This part of the beak also expresses different sets of genes in different beak shapes. TGFβ receptor type II (TGFβIIr), β-catenin, and Dickkopf-3 (Dkk3) are all signal transduction cascade genes that are expressed at much higher levels in the large ground finch beaks compared to smaller beaked finches. These three genes were expressed in broader domains in larger beaks than in smaller beaks when looking at all five species in the figure above (Figure 9)14.
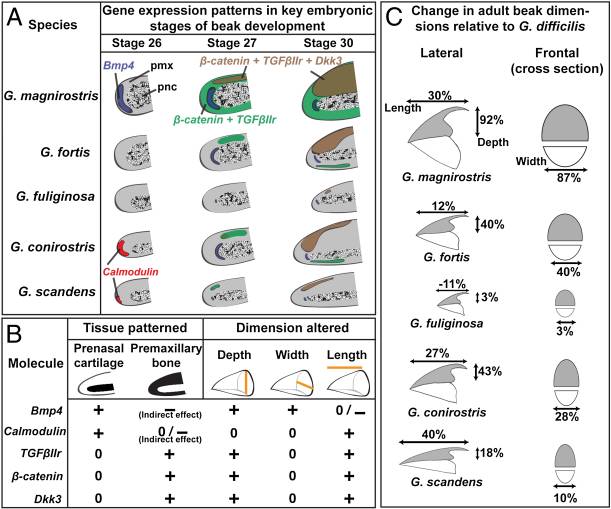
These findings buoyed the idea that allometric growth can fuel rapid evolution by changing the scale of a particular feature. Changing the scale just refers to scaling something up or down, making it larger or smaller in one or all dimensions. For example a large square is simply a scaled-up version of a small square. A rectangle would be a square that has been scaled larger in one dimension but not the other. Researchers noticed right away that simply changing scaling of the upper beak could explain a lot of the variation in Geospiza and this could be explained by the coordinate system of genetics above (or even a simpler genetic system). However, scaling wasn't enough to explain the variation in other related finch species. To explain that variation, researchers found that both scaling and shearing had to be considered. Shearing refers to a geometric transformation like that seen in the fish example from Thompson et al. above. In a shear transformation, each point moves along x at a distance proportional to its y coordinate (or vice versa) giving a diagonal line from a straight line. Shearing plus scaling was enough to explain at least the length and depth axes of the finch beaks studied (Figure 10)
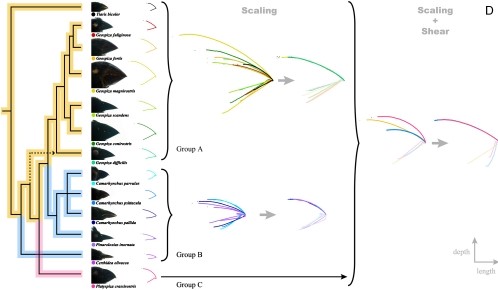
The next question to answer is how broadly applicable is this coordinate system for beak shape transformation? Increasing or decreasing the expression levels of these genes does change chick beak shape in the ways predicted by Mallarino et al.14. This information might lead us to predict that evolution would use this coordinate system to make the wide variety of beaks that we see in living and fossil birds. However, studies of non-Geospiza finches show that evolution is more variable than we might imagine. If we have time, we will discuss the paper: "Closely related bird species demonstrate flexibility between beak morphology and underlying developmental programs".