4.12: Control of Enzymes
- Page ID
- 3041
\( \newcommand{\vecs}[1]{\overset { \scriptstyle \rightharpoonup} {\mathbf{#1}} } \)
\( \newcommand{\vecd}[1]{\overset{-\!-\!\rightharpoonup}{\vphantom{a}\smash {#1}}} \)
\( \newcommand{\id}{\mathrm{id}}\) \( \newcommand{\Span}{\mathrm{span}}\)
( \newcommand{\kernel}{\mathrm{null}\,}\) \( \newcommand{\range}{\mathrm{range}\,}\)
\( \newcommand{\RealPart}{\mathrm{Re}}\) \( \newcommand{\ImaginaryPart}{\mathrm{Im}}\)
\( \newcommand{\Argument}{\mathrm{Arg}}\) \( \newcommand{\norm}[1]{\| #1 \|}\)
\( \newcommand{\inner}[2]{\langle #1, #2 \rangle}\)
\( \newcommand{\Span}{\mathrm{span}}\)
\( \newcommand{\id}{\mathrm{id}}\)
\( \newcommand{\Span}{\mathrm{span}}\)
\( \newcommand{\kernel}{\mathrm{null}\,}\)
\( \newcommand{\range}{\mathrm{range}\,}\)
\( \newcommand{\RealPart}{\mathrm{Re}}\)
\( \newcommand{\ImaginaryPart}{\mathrm{Im}}\)
\( \newcommand{\Argument}{\mathrm{Arg}}\)
\( \newcommand{\norm}[1]{\| #1 \|}\)
\( \newcommand{\inner}[2]{\langle #1, #2 \rangle}\)
\( \newcommand{\Span}{\mathrm{span}}\) \( \newcommand{\AA}{\unicode[.8,0]{x212B}}\)
\( \newcommand{\vectorA}[1]{\vec{#1}} % arrow\)
\( \newcommand{\vectorAt}[1]{\vec{\text{#1}}} % arrow\)
\( \newcommand{\vectorB}[1]{\overset { \scriptstyle \rightharpoonup} {\mathbf{#1}} } \)
\( \newcommand{\vectorC}[1]{\textbf{#1}} \)
\( \newcommand{\vectorD}[1]{\overrightarrow{#1}} \)
\( \newcommand{\vectorDt}[1]{\overrightarrow{\text{#1}}} \)
\( \newcommand{\vectE}[1]{\overset{-\!-\!\rightharpoonup}{\vphantom{a}\smash{\mathbf {#1}}}} \)
\( \newcommand{\vecs}[1]{\overset { \scriptstyle \rightharpoonup} {\mathbf{#1}} } \)
\( \newcommand{\vecd}[1]{\overset{-\!-\!\rightharpoonup}{\vphantom{a}\smash {#1}}} \)
\(\newcommand{\avec}{\mathbf a}\) \(\newcommand{\bvec}{\mathbf b}\) \(\newcommand{\cvec}{\mathbf c}\) \(\newcommand{\dvec}{\mathbf d}\) \(\newcommand{\dtil}{\widetilde{\mathbf d}}\) \(\newcommand{\evec}{\mathbf e}\) \(\newcommand{\fvec}{\mathbf f}\) \(\newcommand{\nvec}{\mathbf n}\) \(\newcommand{\pvec}{\mathbf p}\) \(\newcommand{\qvec}{\mathbf q}\) \(\newcommand{\svec}{\mathbf s}\) \(\newcommand{\tvec}{\mathbf t}\) \(\newcommand{\uvec}{\mathbf u}\) \(\newcommand{\vvec}{\mathbf v}\) \(\newcommand{\wvec}{\mathbf w}\) \(\newcommand{\xvec}{\mathbf x}\) \(\newcommand{\yvec}{\mathbf y}\) \(\newcommand{\zvec}{\mathbf z}\) \(\newcommand{\rvec}{\mathbf r}\) \(\newcommand{\mvec}{\mathbf m}\) \(\newcommand{\zerovec}{\mathbf 0}\) \(\newcommand{\onevec}{\mathbf 1}\) \(\newcommand{\real}{\mathbb R}\) \(\newcommand{\twovec}[2]{\left[\begin{array}{r}#1 \\ #2 \end{array}\right]}\) \(\newcommand{\ctwovec}[2]{\left[\begin{array}{c}#1 \\ #2 \end{array}\right]}\) \(\newcommand{\threevec}[3]{\left[\begin{array}{r}#1 \\ #2 \\ #3 \end{array}\right]}\) \(\newcommand{\cthreevec}[3]{\left[\begin{array}{c}#1 \\ #2 \\ #3 \end{array}\right]}\) \(\newcommand{\fourvec}[4]{\left[\begin{array}{r}#1 \\ #2 \\ #3 \\ #4 \end{array}\right]}\) \(\newcommand{\cfourvec}[4]{\left[\begin{array}{c}#1 \\ #2 \\ #3 \\ #4 \end{array}\right]}\) \(\newcommand{\fivevec}[5]{\left[\begin{array}{r}#1 \\ #2 \\ #3 \\ #4 \\ #5 \\ \end{array}\right]}\) \(\newcommand{\cfivevec}[5]{\left[\begin{array}{c}#1 \\ #2 \\ #3 \\ #4 \\ #5 \\ \end{array}\right]}\) \(\newcommand{\mattwo}[4]{\left[\begin{array}{rr}#1 \amp #2 \\ #3 \amp #4 \\ \end{array}\right]}\) \(\newcommand{\laspan}[1]{\text{Span}\{#1\}}\) \(\newcommand{\bcal}{\cal B}\) \(\newcommand{\ccal}{\cal C}\) \(\newcommand{\scal}{\cal S}\) \(\newcommand{\wcal}{\cal W}\) \(\newcommand{\ecal}{\cal E}\) \(\newcommand{\coords}[2]{\left\{#1\right\}_{#2}}\) \(\newcommand{\gray}[1]{\color{gray}{#1}}\) \(\newcommand{\lgray}[1]{\color{lightgray}{#1}}\) \(\newcommand{\rank}{\operatorname{rank}}\) \(\newcommand{\row}{\text{Row}}\) \(\newcommand{\col}{\text{Col}}\) \(\renewcommand{\row}{\text{Row}}\) \(\newcommand{\nul}{\text{Nul}}\) \(\newcommand{\var}{\text{Var}}\) \(\newcommand{\corr}{\text{corr}}\) \(\newcommand{\len}[1]{\left|#1\right|}\) \(\newcommand{\bbar}{\overline{\bvec}}\) \(\newcommand{\bhat}{\widehat{\bvec}}\) \(\newcommand{\bperp}{\bvec^\perp}\) \(\newcommand{\xhat}{\widehat{\xvec}}\) \(\newcommand{\vhat}{\widehat{\vvec}}\) \(\newcommand{\uhat}{\widehat{\uvec}}\) \(\newcommand{\what}{\widehat{\wvec}}\) \(\newcommand{\Sighat}{\widehat{\Sigma}}\) \(\newcommand{\lt}{<}\) \(\newcommand{\gt}{>}\) \(\newcommand{\amp}{&}\) \(\definecolor{fillinmathshade}{gray}{0.9}\)It is appropriate that we talk at this point about mechanisms cells use to control enzymes. There are four general methods that are employed. They include
- allosterism
- covalent modification
- access to substrate
- control of enzyme synthesis/breakdown
Some enzymes are controlled by more than one of these methods.
Allosterism
The term allosterism refers to the fact that the activity of certain enzymes can be affected by the binding of small molecules to the enzyme. In allostery, the molecules that are binding are non-substrate molecules that bind at a place on the enzyme other than the active site.
An excellent example of allosteric control is the regulation of HMG-CoA reductase, which catalyzes an important reaction in the pathway leading to the synthesis of cholesterol. Binding of cholesterol to the enzyme reduces the enzyme’s activity significantly. Cholesterol is not a substrate for the enzyme, but, notably, is the end-product of the pathway that HMG-CoA catalyzes a reaction in. When enzymes are inhibited by an end-product of the pathway in which they participate, they are said to be feedback inhibited.
Feedback inhibition always operates by allosterism and further, provides important and efficient control of an entire pathway. By inhibiting an early enzyme in a pathway, the flow of materials for the entire pathway is stopped or reduced, assuming there are not alternate supply methods. In the cholesterol biosynthesis pathway, stopping this one enzyme has the effect of shutting off (or at least slowing down) the entire pathway.
Another excellent example is the enzyme aspartate transcarbamoylase (ATCase), which catalyzes an early reaction in the synthesis of pyrimidine nucleotides. This enzyme has two allosteric effectors, ATP and CTP, that are not substrates and that bind at a regulatory site on the enzyme that is apart from the catalytic, active site. CTP, which is the end-product of the pathway, is a feedback inhibitor of the enzyme. ATP, on the other hand, acts to activate the enzyme when it binds to it.
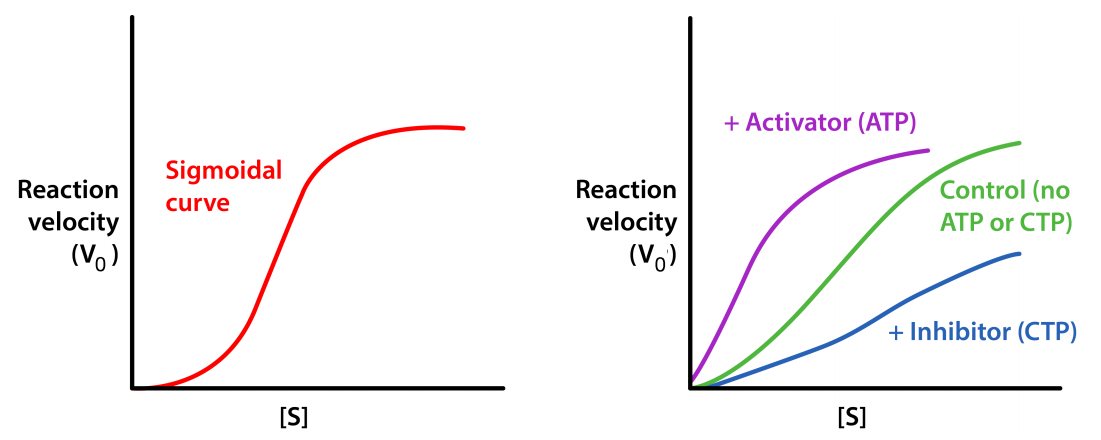
Allosterically, regulation of these enzymes works by inducing different physical states (shapes, as it were) that affect their ability to bind to substrate. When an enzyme is inhibited by binding an effector, it is converted to the T (also called tight) state, it has a reduced affinity for substrate and it is through this means that the reaction is slowed. On the other hand, when an enzyme is activated by effector binding, it converts to the R (relaxed) state and binds substrate much more readily. When no effector is present, the enzyme may be in a mixture of T and R state. The V vs. S plot of allosteric enzymes resembles the oxygen binding curve of hemoglobin (see HERE). Even though hemoglobin is not an enzyme and is thus not catalyzing a reaction, the similarity of the plots is not coincidental. In both cases, the binding of an external molecule is being measured – directly by the hemoglobin plot and indirectly by the enzyme plot, since substrate binding is a factor in enzyme reaction velocity.
Covalent Control of Enzymes
Some enzymes are synthesized in a completely inactive form and their activation requires covalent bonds in them to be cleaved. Such inactive forms of enzymes are called zymogens. Examples include the proteins involved in blood clotting and proteolytic enzymes of the digestive system, such as trypsin, chymotrypsin, and others. The zymogenic forms of these enzymes are known as trypsinogen and chymotrypsinogen, respectively. Synthesizing some enzymes in an inactive form makes very good sense when an enyzme’s activity might be harmful to the tissue where they are being made. For example, the painful condition known as pancreatitis arises when digestive enzymes made in the pancreas are activated too soon and end up attacking the pancreas.
Blood clotting involves polymerization of a protein known as fibrin. Since random formation of fibrin is extremely hazardous (heart attack/stroke), the body synthesizes fibrin as a zymogen (fibrinogen) and its activation results from a “cascade" of activations of proteases that arise when a signal is received from a wound. Similarly, removal of fibrin clots is also controlled by a zymogen (plasminogen), since random clot removal would also be hazardous.
Another common mechanism for control of enzyme activity by covalent modification is phosphorylation. The phosphorylation of enzymes (on the side chains of serine, threonine or tyrosine residues) is carried out by protein kinases. Enzymes activated by phosphorylation can be regulated by the addition of phosphate groups by kinases or their removal by phosphatases.
Other Controls of Enzymes
Other means of controlling enzymes relate to access to substrate (substrate-level control) and control of enzyme synthesis. Hexokinase is an enzyme that is largely regulated by availability of its substrate, glucose. When glucose concentration is low, the product of the enzyme’s catalysis, glucose-6-phosphate, accumulates and inhibits the enzyme’s function.

Regulation of enzymes by controlling their synthesis is covered later in the book in the discussion relating to control of gene expression.